Review article
New Maritime Development: Towards the Theory and Practice of Transformable Area Systems
Vejn Sredić1 and Martin Wynn2*
1Independent researcher, project manager and business development manager, based in Germany
2Associate Professor in Information Technology at the University of Gloucestershire, UK
Martin Wynn, Associate Professor in Information Technology at the University of Gloucestershire, UK
Received Date:February 14, 2024; Published Date:February 26, 2024
Abstract
The recent expansion in floating city structures has underscored the future potential of maritime and port-side developments for industry, housing, and other land uses. Transformable Area Systems (TASs) can play a key role in such sustainable coastal and maritime construction and have a major impact on how factories are planned and implemented. This article explains the basics of the TAS concept and assesses its potential in the context of marine developments. The article concludes that the increased modularity of TAS developments significantly enhances the potential to integrate and disintegrate individual objects and structures, and that TAS-based factories can offer new possibilities with regard to pre-producible, pre-testable, reusable, exchangeable, and scalable objects and structures. However, their application in practice is restricted at present by a lack of awareness and the large initial investment that is required. However, the cost-benefit equation for TASs is likely to re-balance in favour of net benefits, and will lead to more developments like those currently being constructed in the cash-rich nations of the Middle East. TASs have the potential to contribute to the improved functioning, sustainability, and future viability of factories and other structures, and their onward development, in maritime areas.
Keywords:Transformable Area Systems; TAS; Maritime Development; Factory of the Future, Fourth Industrial Revolution; Sustainable Development; Digitalisation; Lifecycles
Introduction
The Oxagon floating structure in Saudi Arabia has been heralded as a breakthrough in urban area design that is “redefining the traditional industrial model”, being an “integrated port and supply chain system” [1] (para. 1). There are an increasing number of other developments globally that use floating structures as a platform for industrial and residential uses. For example, Dubai Maritime City (Figure 1) “offers easy access to the sea, airports, and public transportation” and is “connected by a causeway to the wider road network, providing access to fast-moving expressways” [2] (para. 1). As a specialised maritime cluster, it acts as a “pit stop for all of the marine industry’s needs” (para. 2). In Kuwait, Sabah Al Ahmad Sea City is being built in ten phases and is considered a pioneering project in the region due to its environmentally sustainable construction techniques [3]. Construction of new maritime and port-side complexes out over the sea is in evidence in many countries, notably in the Middle East and China.
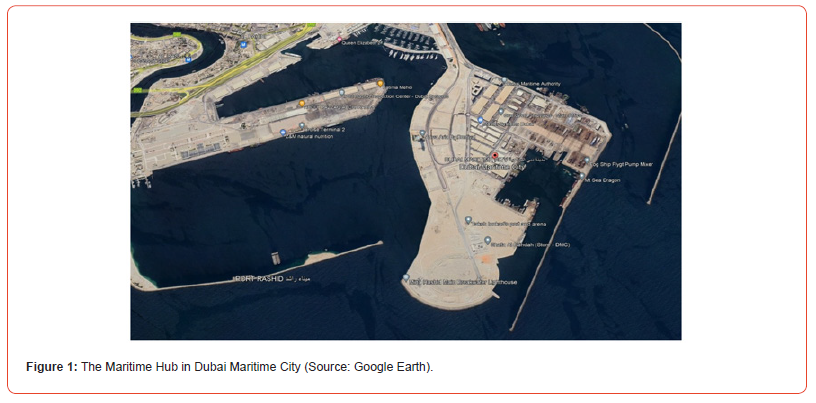
The Bluefield® concept was put forward over a decade ago as a “plug and produce” option for flexible maritime developments (Figure 2) [4], and subsequent research at the University of Gloucestershire led to further refinement of the conceptual underpinning and application potential of such construction projects [5]. This research centred on the development of Transformable Area System (TAS) concepts – discussed below – and Bluefield®, which concerns the development of TASs in maritime environments. These structures have several layers performing separate functions served by integrated modular supply and disposal infrastructure, and can be connected to other TAS elements.
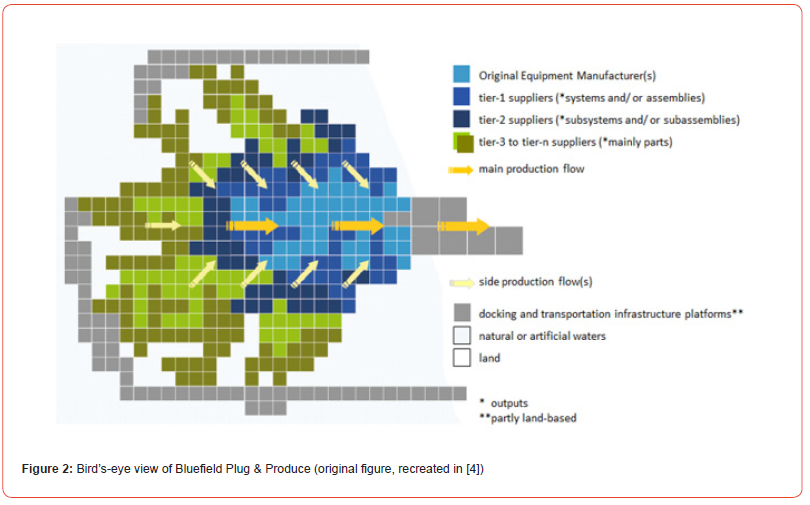
The original motivation for this research came from the evident difficulty in reconfiguring land-based factory sites and associated developments. This gave rise to the identification and definition of a range of conceptual components grouped under the umbrella term of Transformable Area Systems. Whilst the application of these concepts on land has been slow because of the physical hurdles of installing transformable sub-surface structures, there has been clearer application in maritime environments [6]. A number of patents were registered for the application of these concepts in maritime environments [7,8], and a research and development team in Germany is progressing further research on sustainable concrete structures, other materials and composites, couplings of floating elements, and other aspects of maritime TASs. Here, an overview of the key issues is set out and explained.
TAS Components and Terminology
There is a range of components underpinning the overall TAS development concept. A TAS is a modular, mobile, pluggable and scalable development, exhibiting greater transformability compared with traditional factory structures, for example. The TAS concept is not only an opportunity for port-side industry and factory development, but also for many different structures and land uses, and their combination. Bluefield® signifies the application of TASs in maritime environments.
The TAS substructure is below ground level, comprising various structures, objects and areas. For example, supply and disposal infrastructure, and machine foundations may be in the first susubstructure layer, whilst the base layer comprises a fluid tank system. Above ground level is the TAS superstructure (Figure 3). The general structure involves the dimensions, shapes, positions and connections of the main areas, objects and structures and their arrangement and linkage e.g., materials, production and traffic flows [5]..
TAS based port-side developments exhibit a transformability that allows the structure to adapt and change its configuration. This may entail construction extensions or relocation of structures. Transformation enablers [9] are properties that impact on the transformability of structures and include scalability, modularity, linking ability and pluggability. Fundamental enablers, on the other hand, concern the basic parameters of structures, and include things such as area size, area shape, and movable area size (MAS), this latter determining the area that can be moved and whose shape and other properties can be adapted as required [5]. Accelerators – such as pre-producibility, pre-testability, and reusability - are capabilities that speed various aspects of TAS development, such as the planning, building or transformation of different constructions [5,10]. The ability to move different areas, objects and structures within one location is considered inner mobility, whereas outer mobility is concerned with the ability of relocating areas, objects and structures to new sites [11]. Inner and outer transformability reflect the combined impact of enablers, accelerators and related units, whilst agility refers to the dynamics of factories, production networks and other building types and the capability to transform.
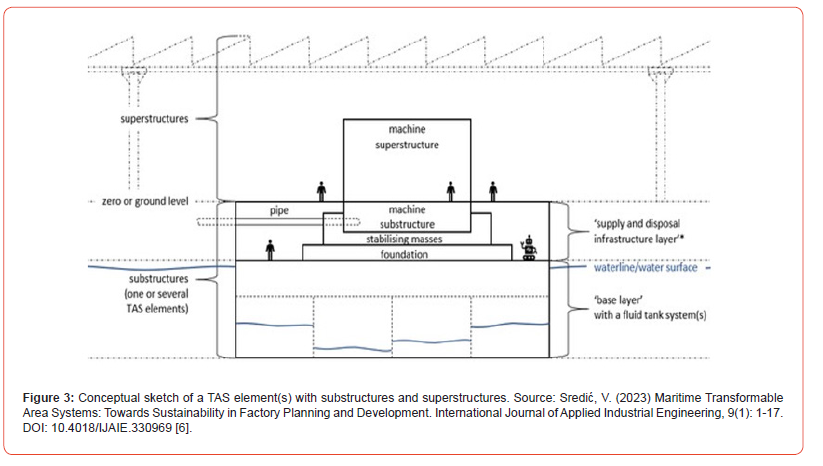
Transformability and modular factories are discussed in some factory planning literature [12,13], and are mentioned in Industry 4.0, and the “factory of the future” literature, but there is very little focus on the need for transformability of areas and the linkage with sustainability and the circular economy. TASs offer potential for production network and supply chain efficiency gains, as a more effective production and logistics flow structure can be maintained [4,5], and longer lifecycles achieved [5,6].
The Potential of Maritime TASs
TAS concepts are of particular relevance today as new developments are designed and constructed in different global maritime environments. The diverse advantages of floating structures are well-documented [14,15]. Fifty years ago, Scanlan [14] set out the potential benefits of floating structures, and more recently Wang et al. [16] have pointed out their potential in addressing coastal ecosystem degradation, rising sea levels and land shortages. In similar vein, Lim [17] (p. 391) concluded that “attractive candidates for floating out include transshipment ports, power stations . . . data centres, hydrocarbon storage tanks, prisons, vegetable farms [etc.]”. In sum, the transformability of floating structures and their benefits are increasingly recognised. One interesting aspect here put forward by Wang et al. [16] is the possible reuse of unwanted buildings moved from developed to developing world environments. The authors note “as floating structures are mobile, it is possible now to relocate used office buildings, residences, hospitals and factories to less developed countries for another cycle of productive life instead of demolishing it”.
The potential of water bodies and coastal areas for transformable construction is just beginning to be recognised. For example, the advantages of maritime-based factories using TAS components over land-based factories are becoming increasingly evident, notably the diminishing availability of new sites for factories in most developed economies. Simple pontoon systems normally have only one layer – the floating element(s) itself – which limits their use and potential. On the other hand, TAS developments allow the advantageous integration and passage of pipes, cables, and other infrastructural elements. A further benefit of maritime TASs is that the superstructure layer(s) can by and large be kept away from water, even though in ocean environments, changing wind and weather conditions, waves, salt, evaporation, and marine organisms impact structures, and make their development challenging, including the definition of appropriate composites, couplings and other aspects.
Transformable areas may comprise several floating TAS elements, which can be multifunctional or used for specific purposes. They can be used together with other advanced structures and objects, in which fundamental enablers (e.g., different MASs, shapes and area and substructure characteristics) will determine the degree and speed of transformability. TASs also offer combinability options with scalable TAS satellite units [5]. Further research and collaboration will be needed that draws upon different fields of knowledge and crosses disciplinary boundaries, and which exploits the opportunities offered by digitalisation and advanced energy generation options.
Summary Discussion
There are a number of issues regarding the future deployment of TASs meriting summary discussion. First, there are issues related to the structural requirements of TASs. Connections between floating elements and layers lead to design and operational complexity and have an impact on the design and sizes of structures, and their subsequent operation (and vice versa). However, current and new technologies will help address these issues, and pre-productivity, pre-testability, and reuse will also play their part. Second, and related to the above issue, the rapid advance and development of digital technologies will help address a range of issues in the design, planning and implementation of TAS constructions, supporting enhanced modelling, visualisation, simulation and project management. Third, TAS developments can contribute to sustainability and a transition to the circular economy. Artificially manufactured structures have more mass than all living beings [18], emphasising the value of the transformability and reuse of structures and their materials. Maritime TAS developments can also free up terrestrial areas for other needs and uses. Fourth, there are valid concerns regarding safety and the environmental impact of TAS developments. The need for buoyancy means that calm waters are preferable for TAS locations, and existing coast-side industrial zones could be used for the gradual introduction of TASs if safety and environmental matters are appropriately addressed. Fifth, the cost-benefit equation for TASs is likely to re-balance in favour of net benefits. At present, the large initial investment that is required is seen as a barrier in many parts of the world, and the necessary competencies to develop TASs are considerable. However, net costs will decrease as and when TASs become productised and standard; and the growing concerns around sustainability and the increasing costs of environmental degradation will also help re-balance the cost-benefit equation.
In summary, the potential advantages of maritime TASs and structures are many. They include the simplification of the transformation of structures; the provision of a transformable physical framework for sharing common services, which can be retained or adapted, consisting of supply and disposal facilities and infrastructures, and transportation infrastructures; structures can be pre-produced, assembled and integrated into new and existing TAS developments; flexible and sustainable structure lifecycles, characterised by high operational, energy, and resource efficiency; and an increase in the potential combination of uses and synergies. Lim [17], in his assessment of development deploying maritime structures, argues that “value arises from the fact that unlike landbased real estates, . . . [a float] may be repurposed and deployed” (p. 391). Other statements refer to the utilisation of maritime structures for a period of more than 100 years, highlighting their advantages and sustainability [17]. This, then, is the critical difference that may lead to a far wider use of maritime structures in future, as land becomes scarcer, the move towards net zero demands increased reuse and recycling of materials, and technological advances facilitate more efficient and flexible construction methods. Over time, TASs will become increasingly relevant and necessary, and require a fuller development of related theory and practice. It is hoped this article has made a small contribution to this endeavour.
Acknowledgement
None.
Conflict of Interest
None.
References
- NEOM (2024) OXAGON: Redefining the traditional industrial model.
- Dubai Maritime City (2024) The Premium Maritime Hub in Dubai.
- Jones D A, Nithyanandan M, Williams I (2012) Sabah Al-Ahmad Sea City Kuwait: development of a sustainable man-made coastal ecosystem in a saline desert. Aquatic Ecosystem Health & Management 15: 84-92.
- Sredic V (2011) Ganzheitliche Wertschöpfung und unbegrenzte Wandlungsfähigkeit durch Bluefield Plug & Produce. Konzept für einen universell einsetzbaren modularen und mobilen Industriebau [In English: Holistic value creation and unlimited transformability through Bluefield Plug & Produce: concept for a universally applicable modular and mobile industrial building]. wt Werkstattstechnik Online [wt Workshop Technology Online] 101(4): 194-199.
- Sredić V (2018) The Impact of Transformable Area Systems on Factory Planning Theory and Practice: A Study of Automobile Manufacturing Plants [PhD thesis]. University of Gloucestershire UK.
- Sredić V (2023) Maritime Transformable Area Systems: Towards Sustainability in Factory Planning and Development. International Journal of Applied Industrial Engineering 9(1): 1-17.
- Sredic V (2012) Nutzflächen-Schwimmplattform und Schwimmelemente zu deren Herstellung. Available at: https://depatisnet.dpma.de/DepatisNet/depatisnet?action=bibdat&docid=DE102011012113A1 (Patent Application DE102011012113(A1), GPTO, Application Number: DE20111012113 20110223) (accessed on 30 January 2024).
- Sredic V (2012) Nutzflächen-Schwimmplattform und Schwimmelemente zu deren Herstellung. Available at: https://depatisnet.dpma.de/DepatisNet/depatisnet?action=bibdat&docid=WO002012113508A1 (Patent Application WO2012113508(A1), EPO, Application Number: WO2012EP00560 20120207) (accessed on 30 January 2024).
- Wiendahl H P, Reichardt J, Nyhuis P (2015) Handbook Factory Planning and Design (R. Rossi, Trans.). Springer.
- Hildebrand T (2005) Theoretische Grundlagen der bausteinbasierten, technischen Gestaltung wandlungsfähiger Fabrikstrukturen nach dem PLUG+PRODUCE Prinzip [Dissertation]. Technische Universität Chemnitz [Technical University of Chemnitz].
- Wirth S, Enderlein H, Peterman J (2000) Kompetenznetze der Produktion. In Fakultät für Maschinenbau und Verfahrenstechnik, Institut für Betriebswissenschaften und Fabriksysteme (Ed.), Vortragsband IBF- Fachtagung 2000: Vernetzt planen und produzieren. Chemnitz: Technische Universität Chemnitz [Technical University of Chemnitz].
- Schenk M, Wirth S, Müller E (2014) Fabrikplanung und Fabrikbetrieb: Methoden für die wandlungsfähige. vernetzte und ressourceneffiziente Fabrik (2nd). Springer Vieweg.
- Grundig C G (2018) Fabrikplanung: Planungssystematik – Methoden – Anwendungen (6th ed.). Hanser.
- Scanlan H J (1974) Floating Factory for the Manufacture of Building Components (United States Patent No. 3,785,314). United States Patent and Trademark Office (USPTO).
- Wang CM, Wang B T (2015) Large Floating Structures: Technological Advances. Springer.
- C M Wang, S H Lim, Z Y Tay (2020) Lecture Notes in Civil Engineering: 41: WCFS2019: Proceedings of the World Conference on Floating Solutions. Singapore: Springer.
- Lim SH (2020) Seascape the Landscape of Singapore, Repurposing Land in a Land Scarce Nation. In Lecture Notes in Civil Engineering: 41: WCFS2019:385-410.
- Deutschlandfunk (2020) Künstlich hergestellte Produkte haben erstmals mehr Masse als alle Lebewesen.
-
Vejn Sredić and Martin Wynn*. New Maritime Development: Towards the Theory and Practice of Transformable Area Systems. Mod Concept Material Sci. 5(5): 2024. MCMS. MS.ID.000624.
-
Transformable Area Systems, TAS, Maritime Development, Factory of the Future, Fourth Industrial Revolution, Sustainable Development, Digitalisation, Lifecycles
-
This work is licensed under a Creative Commons Attribution-NonCommercial 4.0 International License.