Research Article
Application of Luminous Textile Coated by TiO2 For Photocatalytic Removal of Acetic Acid, Formaldehyde and Toluene from Indoor Air
Marwa Hamandi1, Ha‐Son Ngo1, Frédéric Dappozze1, Lina Lamaa2, Laure Peruchon2, Cédric Brochier2 and Chantal Guillard1*
1IRCELYON, CNRS‐Université Claude Bernard Lyon 1, 2 av. Albert Einstein, 69626 Villeurbanne Cedex, France
2Brochier Technologies, 90 rue Frédéric Faÿs, 69100 Lyon, France
Chantal Guillard, IRCELYON, CNRS‐Université Claude Bernard Lyon 1, 2 av. Albert Einstein, 69626 Villeurbanne Cedex, France.
Received Date: May 15, 2019; Published Date: May 20, 2019
Abstract
Luminous textile coated by silica and TiO2 is studied for indoor air treatment. The textile aging induced by UV exposure in the presence of TiO2 coating was studied, showing a good durability of the textile after protection by Silica layer unlike silica‐free textile. Interesting photocatalytic activity was achieved for the photocatalytic luminous textile to remove three different VOCs in air which are acetic acid (AA), formaldehyde and toluene. The effect of number of photons and residence time was carefully highlighted for both TiO2 P25 in powder or supported in the luminous textile. Then, a comparative study, between the classic system composed of TiO2 powder and the innovative system integrating photocatalyst and light, was described. The advantage of the photocatalytic luminous textile is their performance associated to an important energy gain due to the use of one UV‐LED to homogeneously irradiate an important surface of textile allowing the development of compact photocatalytic device.
Keywords: Luminous textile; Optical fibers; UV irradiation; Photocatalysis; VOCs degradation
Abbreviations: LED: Light‐Emitting Diode; UV: Ultraviolet; VOCs: Volatile Organic Compounds; AOP Advanced Oxidation Processes; PMMA: Polymethyl Methacrylate; CCD: Charge Coupled Device; GC: Gaz Chromatography; ppmv: parts per million by volume
Introduction
Air pollution from indoor environments is becoming increasingly a public health concern. The permanent presence of chemical pollutants causes various respiratory and chronic diseases [1]. From these various pollutants, there are volatile organic compounds (VOCs) and carboxylic acids which are present in air in different concentrations as a result of biogenic emissions, anthropogenic emissions, materials and lacquer coating [2‐5]. Thus, their removal from air is a major challenge to prevent human health issues.
Photocatalysis is a very promising technology for air treatment. The main advantage of this process is not just the remove of VOCs but also their mineralization at room temperature and atmospheric pressure due to generation of reactive oxygen species, OH•, O2• − and HO2 • from air and humidity. It occurs by activating a photocatalyst using a certain wavelength of UV light. A large range of semi‐conductors is available to induce photocatalysis, while TiO2 is seen as one of the most promising photocatalysts for practical applications. This material is cheap, abundantly available and chemically stable.
The first step in a photocatalytic reaction is when photons with sufficient energy are absorbed by the photocatalyst, generating an electron and a hole (e‐ and h+). These electrons and holes can induce redox reactions, often via the formation of radicals as shown in equations 1 and 2. These radical species allow oxidation of organic compounds.

The photocatalytic process is an efficient method to remove organic pollutants present in gaseous phase [6]. However, there are still some drawbacks with current methods, namely related to the reactor systems and UV light source. In fact, classical light sources are hazardous due to mercury toxicity with relatively high energy consumption (20‐150W) and a relatively low durability which is about 2000 hours. They have generally a low photonic efficiency and they emit large spectral wavelength. The solutions proposed in the present work concern the use of a luminous textile with treated optical fibers. In fact, these fibers are connected to one ultra‐violet light emitting diode (UV‐LED) source. Consequently, it can carry and supply the UV light onto all over the textile area with little energy (3W) and a better durability which exceeds 17000 hours. Once coated using a suspension of TiO2, the textile subsequently becomes a photocatalytic material leading UV light into the bulk of the photocatalytic bed and diffusing the light in a homogeneous manner over the entire surface. Some researches using optical fibers as a support and different reactors were developed for air and water treatment [3,4]. The first experimental study using optical fibers in photocatalysis was presented in 1994 for the water treatment of 4‐chlorophenol by Hofstadler, et al. [7]. Then, various works using optical fibers as support of photocatalyst and different reactors were proposed for the air and water treatment [8‐12]. This new luminous textile, called lightex® [13‐14] has been developed by Brochier Technologies [15]. Then, its photocatalytic efficiency for VOC degradation was demonstrated through formaldehyde degradation by P‐A. Bourgeois, et al. [16]. The coating method of the textile and its characterization was already studied [17]. For the present work, luminous textile coating was optimized by adding firstly silica to improve the textile durability in presence of TiO2 under UV in gas phase and the photoreactor was developed to be more adequate to the luminous textile and for industrial applications. Three different pollutants from three different chemical families are studied: a carboxylic acid, an aldehyde and an aromatic hydrocarbon acetic acid (99% purity), formaldehyde (99% purity) and toluene (99% purity) respectively.
The objectives of the publication are first to evaluate the aging of the photocatalytic luminous textile, then compare the disappearance and mineralization of acetic acid, formaldehyde and toluene in presence of photocatalytic luminous textile as a function of the number of photons activating the photocatalyst and finally compare the photonic efficiency and the electrical energy consumed using photocatalytic luminous textile and TiO2 powder using a UV‐lamp at the same wavelength.
Materials and Methods
Elaboration of optical fiber textile
Optical fiber textile connected to UV LED is produced by Brochier Technologies Company (UVtex®). It is composed of polyester fibers, which provide mechanical properties to the textile, and optical fibers which are in poly‐methyl methacrylate (PMMA) resin for the core with 480 μm of diameter and covered with 10μm of fluorinated polymer [16]. These two types of fibers are fixed to the textile shape by binding weave following the Jacquard process meaning the interlacing, in a same plane, of fibers disposed in the direction of the chain (textile fiber) and some fibers disposed perpendicular to chain fibers, in the direction of the weft (optical fibers). Classically, optical fibers are able to transmit light from the beginning to the end of the fiber. However, Brochier technologies has developed a micro texturing process which is a necessary treatment allowing a uniform light output across the surface of the optical fibers, according to their patents [13,14]. All optical fibers are gathered to one extremity in a connector sealed with organic adhesive. Once this textile is connected to light source, it allows homogeneous irradiance. The lamp connected to the luminous textile is a LED lamp 3W with UV emission centered at 365nm.
TiO2 deposition and aging study
The photocatalyst used is TiO2 Degussa P25. Before photocatalyst coating, luminous textile needs to be protected by silica suspension (Aerodisp W7622 purchased from Evonik Degussa) as demonstrated in a previous work of C. Indermuhle et al. [17].
Local emitted light intensities were measured by CCD spectrometer Avantes AvaSpec‐ULS2048. Emitted light intensity was measured through 50 points on the surface of the textile before and after TiO2 coating. The study of the coated textile aging is based on the survey of the intensity as function of irradiation time.
Analytical procedures and photocatalytic set‐up
To generate a gas stream of pollutant in air at known concentration, a carrier gas (air quality zero) was sent into a COV generator from Bronkhost filled with paraformaldehyde solid (99% purity) or with acetic acid (99% purity) or toluene (99% purity) liquid.

The inlet concentration of formaldehyde, acetic acid and toluene are respectively 7, 3.5 and 1 ppmv. This textile was coated by TiO2 P25 and connected to a UV‐LED lamp (Ultra‐Violet Light Emitting Diode) by a sealed sleeve, was fixed in a plane and compact reactor (Figure 1). All experiments were performed under continuous flow with a flow rate of 1000 mL.min‐1 and a relative humidity of 50%. The sample was treated overnight under UV irradiation to degrade all organic impurities existing in the sample then three steps were followed: at first, pollutant concentration was stabilized and homogenized by passing the reactor (step1). Then, it was introduced into the reactor and maintained in the dark until reaching a steady state of pollutant concentration (step2). Finally, UV light was switched on and maintained until stabilization of the concentration (step3).
All products and intermediates were analyzed continuously using GC‐PDID/FID (GC: Gas Chromatography; PDID: Pulse Discharge Ionization Detector; FID: Flame Ionization Detector) by filling the gas sample into two‐loop injection valve. A 100μL loop sent the sample through a BP5‐MS column to the FID detector, for toluene, acetic acid and oxygenated compounds separation, while a 500μL loop served a Shin carbon column connected to a PDID detector for CO2 and hydrocarbons analysis. Helium was used as carrier and discharge gas.
Results and Discussions
Optical fiber textile durability
In spite of the advantages of PMMA such as its high ductibility, its big core diameter and low cost, one of major problem to use it in photocatalysis is the photochemical degradation of PMMA optical fibers in presence of UV and TiO2 [18,29]. Consequently, it is important to study their durability during UVA exposure in presence of TiO2. In a previous work [17], durability of this textile under aqueous solution was studied. For the present work durability was studied under air, ambient temperature and atmospheric pression. For this, two types of textile, with a surface of 200 cm2, were prepared. The first was coated by silica Aerodisp W7622, then coated by the photocatalyst, and the second was directly coated by the photocatalyst. Both were exposed to UVA at 365nm emitted by LED. The irradiance on the surface of the textile is about 1mW/cm² homogeneously distributed on all the textile surface throughout optical fiber textile. Along exposure time to UVA light, Irradiance is measured at 25 points on these textiles surface to have representative measurement using radiometric measurements (CCD). Then the irradiance is plotted as function of the average of each line. Figure 2 shows a significant difference between the two textiles coated by silica or not. In the case of luminous textile coated by silica and TiO2 (Figure 2b), the irradiance could be considered as stable during the study of durability. However, in the case of textile coated directly with TiO2 without silica (Figure 2a), an important inhomogeneity of the emitted light distribution over the overall surface of the textile was observed, as expected. The majority is emitted in the first centimeters of the fabrics. Therefore, it was demonstrated that the modification of emitted light intensity distribution is due to direct contact with TiO2 and that the protection by silica avoids the attack of TiO2 to the textile and allows having a good durability.
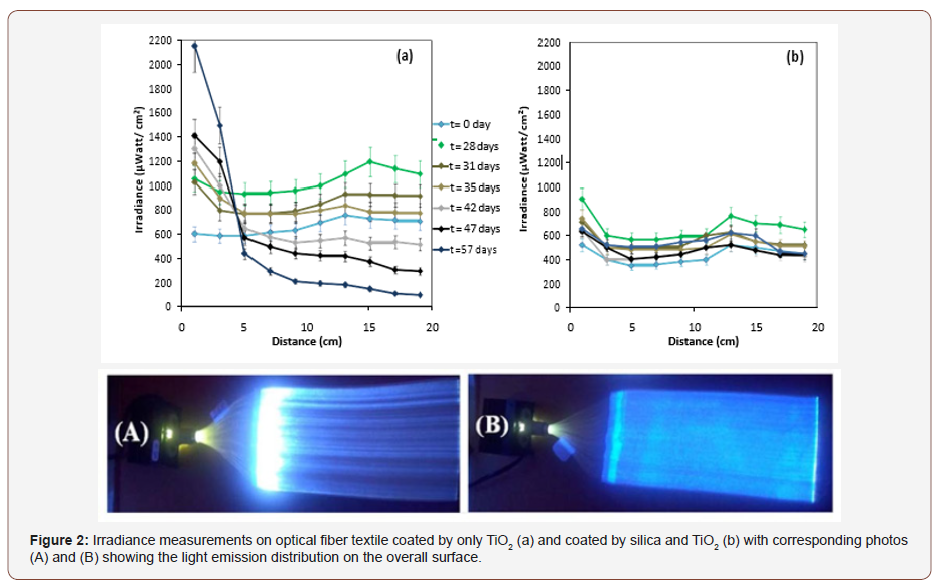
Comparative study for the degradation of 3 different molecules using photocatalytic LED/textile
This present section is the comparison of photocatalytic activity of luminous textile to degrade the three different pollutants. All samples were pretreated 24h under UVA irradiation using 1L min‐1 of air flow under 50% of relative humidity. The inlet concentrations are chosen to have an equivalent carbon number with 7, 3.5 and 1 ppmv for formaldehyde, AA and toluene respectively.
The conversion of pollutants was calculated from the difference between the inlet and outlet concentrations, using the following equation:

In which: Cin is the inlet concentration and the Cout is the outlet concentration.
The selectivity (%) was determined by comparing the concentration of carbon dioxide produced to the theoretical one (if mineralization is complete: 1 mole of toluene should lead to formation of 7 moles of CO2, one mole of AA should lead to formation of 2 moles of CO2 and one mole of formaldehyde leads to 1 mole of CO2 as follows:

As shown in figure 3 conversions of the three different pollutants are interesting with 93%, 71% and 90% for respectively formaldehyde, acetic acid and toluene. The selectivity of CO2 is relatively high with 99%, 90% and 74% putting in evidence the photocatalytic ability of this promising photocatalytic textile to remove these kinds of molecules. In this case, it is important to evaluate this system in terms of number of photons.
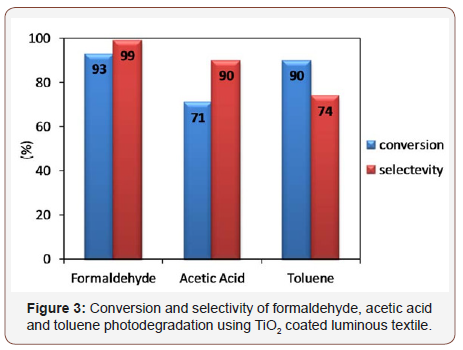
In fact, the number of photons is one of important parameters in photocatalysis. Therefore, the disappearance of different pollutants has been followed as function of the intensity of UV light as presented in figure 4. The number of photons was determined by the equation below:
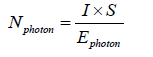
In which I: the irradiance (Watt. cm‐2); S: the reactor surface (cm2); Ephoton is the energy of one photon (Watt) The rate of the pollutant degradation is determined by this equation:
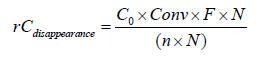
In which rCdisappearance (mol. min‐1.mg‐1): the rate of Carbon degradation; C0: inset concentration (mol.L‐1); Conv: conversion (%); F : flow rate (L.min‐1); N : Avogadro number; n : number of carbon in the molecule; m : weight of TiO2 in the textile (mg).
As shown in figure 4, the rate of the pollutants degradation increases when the photon number increase as expected due to the more important formation of (e‐, h+) pairs. In the case of toluene, a plateau is observed which is due probably to the formation of intermediate products on the surface of photocatalyst which exhibit competition reactions and which can saturate the photocatalyst active surface. This hypothesis is in agreement with the selectivity into CO2 which is only 74% opposite to the selectivity obtained in the case of acetic acid and formaldehyde which are 90%. While in the case of formaldehyde and acetic acid, the degradation rate is proportional to the number of photons with a linear curve confirming the facility of their degradation with fewer intermediates as they have less carbon than toluene and in agreement with their high CO2 selectivity.
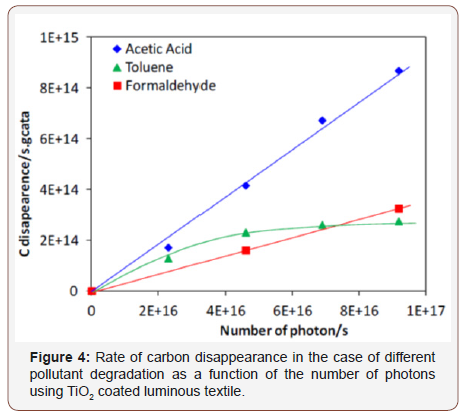
The highest disappearance rate of acetic acid can be explained considering the mechanism of photocatalytic degradation. Indeed, in presence of carboxylic group (COOH), this group can directly react with h+ according to photo‐Kolbe reaction while in the case of the degradation of formaldehyde and toluene, the main reactions occur with hydroxyl radical.
Comparative study between TiO2 powder and TiO2 coated on luminous textile
The photocatalyst used in the present study is commercial TiO2 P25 (anatase/rutile = 80/20) which has a particle size of 20‐30nm. Its density is equal to 3.8 and its specific surface area is about 50 m2 g‐1.
A comparative study between the use of TiO2 P25 in powder form or supported in a luminous textile to degrade AA is the subject of the second part of this paper. For this, luminous textile was used as described previously. In the case of the tests performed in presence of TiO2 P25 powder, the photocatalyst is homogeneously distributed on the surface of a Durapore® filter (Millipore, porosity 0.45μm) located in a cylindrical reactor (diameter 5cm) made of stainless steel and equipped with an optical Pyrex glass window (diameter 4cm) allowing penetration of light from a mercury UV lamp (PLL 18W, Philips) deposited outside the reactor.
The operating conditions used for both experiments are summarized in Table 1.
Table 1: Experimental conditions in the case of TiO2/P25 powder and TiO2/P25 supported on luminous Textile.

Experiments were carried out at the same residence time and the same inlet AA molecular flow. Residence time was determined according to the following equation:
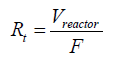
In which Rt: Residence Time (s); Vreactor: Volume of the reactor (L); F: Flow rate (L.s‐1).
The kinetics and mechanism of the photocatalytic degradation of acetic acid using TiO2 P25 powder was already well studied [20]. Otherwise, in the present work, the photocatalytic performance of the two systems is compared at the same acetic acid molecular flow and the same residence time.
As experiments with textile or TiO2 powder were done using two different reactors, it is judicious to determine the quantum efficiency in both cases.
Photonic efficiency was determined according to the following equation:
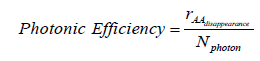
Table 2: Photocatalytic performance for AA degradation in the case of TiO2/P25 powder and TiO2/P25 supported on luminous textile in the same residence time (4.8 s) and the same molar flow (6.5 10-8 mol. s-1).

Table 2 summarizes the results obtained from experiments done by the two systems. Luminous textile demonstrates its efficacy by preserving an interesting photonic efficiency which is equivalent to the photonic efficiency of TiO2powder at a same residence time and a same acetic acid molecular flow.

However, the most important and interesting properties of these photocatalytic luminous textile is their good performance associated to a low electric energy consumption. The LED (3W) connected to luminous photocatalytic textile is compared to experiments using TiO2 powder irradiated by PLL lamp (18W). In the case of LED/ photocatalytic textile system, the electrical energy consumption is 11.5 times lower than this one obtained with PLL lamp/ TiO2 powder system. The photocatalytic luminous textile has the capacity to preserve the same photocatalytic performance with a lower electrical energy consumption, putting in evidence the photocatalytic ability of this luminous textile to remove volatile organic compounds with an interesting energy gain. Besides, the use of textile allows a better light distribution on the surface of the textile in a relatively large surface area using only one LED allowing the development of industrial compact photocatalytic device for air treatment (figure 5).
Conclusion
In the present work, the photocatalytic degradation of formaldehyde, acetic acid and toluene under continuous flow in air was studied. Durability of luminous textile was firstly optimized by adding a silica layer between the textile and the TiO2 coat. Then, this luminous textile coated with TiO2 was used to remove three families of pollutants including carboxilic, aromatic and aldehyde functions, as function of irradiance intensity. Our results show the efficiency of this type of luminous textile to remove and in particular mineralize VOC present in the indoor atmosphere containing VOC concentration below ppmv. Finally, two different systems which are classic system composed of TiO2 P25 powder and TiO2 coated luminous textile had been compared. At a same number of photons, a same residence time and a same inlet acetic acid molecular flow, degradation performance of AA in the presence of photocatalytic textile is equivalent to the degradation performance in presence of TiO2 powder and in addition allows an important energy gain in terms of electrical energy consumption. Actually, at equivalent performance, an energy gain of more than 11 times was found by using this type of photocatalytic luminous textile comparing to the use of TiO2 powder associated to classical lamp. This innovative photocatalyst combining TiO2 and light has demonstrated its efficiency to remove different pollutants in gas phase and to solve some of problems associated to commercial sources such as low energy efficiency or short lifetime for conventional lamps. Furthermore, it shows a uniform surface of light and it is used to irradiate large area of photocatalyst, by using only one LED. In conclusion, this system is ideal for industrial applications using compact reactor.
Acknowledgement
None.
Conflict of Interest
No conflict of interest.
References
- Bernstein JA, Alexis N, Bucchus H, Bernstein IL, Fritz P, et al. (2008) The health effects of nonindustrial indoor air pollution. J Allergy and Clinical Immunology 121(3): 585‐ 591.
- (1982) Ullrich D, R Nagel, B Seifert, Schriftenr Ver Wasser. Boden Lufthyg 53(2): 83‐298.
- Dabek‐Zltorzynska E, McGrath M (2000) Determination of lowmolecular- weight carboxylic acids in the ambient air and vehicle emissions: a review. J Fresenius Anal Chem 367(6): 507‐518.
- Chebbi A, Carlier P (1996) Carboxylic acids in the troposphere, occurrence, sources, and sinks: A review. Atmos Environ 30(24): 4233‐4249.
- Tetrault J (1999) Coatings for Display and Storage in Museums, CCI Technical Bulletin. Canadian Conservation Institute Ottawa 21: 58.
- Verbruggen SW (2015) TiO2 photocatalysis for the degradation of pollutants in gas phase: From morphological design to plasmonic enhancement. Journal of Photochemistry and Photobiology C: Photochemistry Reviews 24: 64‐82.
- Hofstadler K, Bauer R, Novalic S, Heisler G (1994) New Reactor Design for Photocatalytic Wastewater Treatment with TiO2 Immobilized on Fused-Silica Glass Fibers: Photomineralization of 4-Chlorophenol. Environ Sci Technol 28 (4): 670‐674.
- Peill NJ, Hoffmann MR (1995) Development and Optimization of a TiO2-Coated Fiber-Optic Cable Reactor: Photocatalytic Degradation of 4-Chlorophenol. Environmental Science & Technology 29(12): 2974‐2981.
- Sun RD, Nakajima A, Watanabe I, Watanabe T, Hashimoto K (2000) TiO2-coated optical fiber bundles used as a photocatalytic filter for decomposition of gaseous organic compounds. Journal of Photochemistry and Photobioloogy A: Chemistry 136(1‐2): 111‐116.
- Choi W, Ko JY, Park H, Chung JS (2001) Investigation on TiO2-coated optical fibers for gas-phase photocatalytic oxidation of acetone. Applied Catalysis B: Enviromental 31(3): 209‐220.
- Joo H, Jeong H, Jeon M, Moon I (2003) The use of plastic optical fibers in photocatalysis of trichloroethylene. Solar Energy Materials and solar Cells 79(1): 93‐101.
- Danion A, Disdier J, Guillard C, Abdelmalek F, N. Jaffrezic‐Renault N (2004) Characterization and study of a single-TiO2-coated optical fiber reactor. Applied Catalysis B: Environmental 525(3): 213‐223.
- Brochier C, Malhomme D, Deflin E‐ Brevet (2007) Textile tablecloth with depolluting properties by photocatalysis‐ n° de délivrance FR2910341, 2008‐06‐27 (A1) et FR 2910341, 2009‐02‐ 06 (B1).
- Brochier C, Deflin E, Breting T – Brevet N° WO 2008/061789, Complexe éclairant. «Complexe éclairant comportant une source lumineuse présentant une nappe de fibres optiques » ‐ FR2907194 (A1) 2008‐074‐18 et FR2907194 (B1) – 2010‐08‐27.
- SAS. www.brochiertechnologies.com.
- Bourgeois PA, Puzenat E, Peruchon L, Simonet F, Chevalier D, et al. (2012) Characterization of a new photocatalytic textile for formaldehyde removal from indoor air. Applied Catalysis B : Environmental 128: 171‐178.
- Indermuhle C, Puzenat E, Simonet F, Peruchon L, Brochier C, et al. (2016) Modelling of UV optical ageing of optical fibre fabric coated with TiO2. Applied Catalysis B: Environment 182: 229‐235.
- Taguenang JM, Kasu A, Ruffin PB, Brantley C, Edwards E, et al. (2008) Reversible UV degradation of PMMA plastic optical fibers. Optics Communications 281(8): 2089-2092.
- Yu JM, Tao XM, Tam HW, Demokan MS (2005) Modulation of refractive index and thickness of poly (methyl methacrylate) thin films with UV irradiation and heat treatment. Appl Surf Sci 252(5): 1283-1292.
- Ngo S, Betts L, Dappozze F, Ponczek M, George C, et al. (2017) Kinetics and mechanism of the photocatalytic degradation of acetic acid in absence or presence of O2. Journal of Photochemistry and Photobiology A: Chemistry 339: 80‐88.
-
Chantal Guillard, Marwa Hamandi, Ha‐Son Ngo, Frédéric Dappozze, Lina Lamaa, et al. Application of Luminous Textile Coated by TiO2 For Photocatalytic Removal of Acetic Acid, Formaldehyde and Toluene from Indoor Air. J Textile Sci & Fashion Tech. 2(5): 2019. JTSFT. MS.ID.00046.
-
Luminous textile, Optical fibers, UV irradiation, Photocatalysis, VOCs degradation
-
This work is licensed under a Creative Commons Attribution-NonCommercial 4.0 International License.