Review Article
Influence of Green Terraces in Evaluating the Action of Wind on Buildings
Isopescu Dorina Nicolina*, Maxineasa Sebastian George and Baciu Ioana Roxana
Faculty of Civil Engineering and Building Services, “Gheorghe Asachi” Technical University of lasi, Nr. 1, 700050, Romania
Isopescu Dorina Nicolina, Faculty of Civil Engineering and Building Services, “Gheorghe Asachi” Technical University of lasi, Nr. 1, 700050, Romania.
Received Date: August 30, 2021; Published Date: September 08, 2021
Abstract
The development of the construction sector, as a result of the special emphasis of recent years in the implementation of the principles of sustainability, has included various directions: from structural certification of environmentally friendly materials in terms of their durability and carbon dioxide emissions, and the innovation of building systems respecting the energy efficiency principles requirements, until to the innovation of the shape and volume concepts of buildings. All over the world there are recognized examples of nature-friendly architecture. The complexity of sustainable development in construction is increasingly becoming cross-cutting research, which covers the integrated techniques of materials science, building physics, with the circular economy, and with plant science. This challenge offers new opportunities for buildings to become less polluting and more resistant to climate change.
Green buildings, in fact, green roofs and facades, help to create viable and sustainable built spaces. The benefits are much more than the structural or environmental protection, green structural components in buildings can contribute to the well-being of occupants, such as noise reduction, and can improve indoor air quality.
This article examines the importance of green roofs in sustainable buildings and their effect on the strength and stability of buildings against wind action, based on design regulation in force. In addition to the general presentation of the literature, several recent applications of greenery in projects are presented, to illustrate the architectural added value.
Keywords:Green terrace; Wind actions; Sustainability; Building stability; Building durability
Introduction
Currently, one of the most debated issues worldwide is the relationship and mutual influence between the natural environment and the built environment. The built environment is an artificial environment created by mankind and, although it is the support of the civilization development, current research shows that this field of activity has several adverse effects, including the fact that it is responsible for 33% of total emissions of greenhouse gases and 20% of the total amount of waste produced globally. Also, the processes specific to the construction and use of buildings are responsible for 40% of global energy consumption [1,2]. All these observations based on substantiated analyses, emphasize the fact that the built environment has a negative major impact on the natural one, having a key role in achieving the requirements of the development of global sustainability.
Urbanization and the impact of this trend on climate change is one of the most analysed topics in global climate change debates. In the most developed countries, the urbanization process is expected to reach about 83% by 2030 [3]. Due to the accelerated economic development, the urbanization process shows accelerated growth in most countries, to the detriment of the natural landscape as well as the requirements of environmental protection [4]. An increasingly approached solution is the concept of greening the built environment as an integrated approach to achieving sustainable urban development, mitigating the impact of rapid urbanization, and improving the quality of life [5].
The Paris Agreement on Climate Change, as well as the policies and strategies promoted by the European Union, attach particular importance to increasing the green areas in cities, reducing carbon dioxide emissions and energy consumption, but also to developing new green technologies. A modern and viable solution for mitigating negative environmental effects is identified in greening systems, such as the horizontal green building components (roofs) and vertical green building components (walls) (Figure 1). By rehabilitating with these solutions, the existing built environment can become the support for the improvement of the urban micro-climate conditions. Even though they are currently hotly debated, these concepts are not new, being found in the concerns of society since ancient times [6].
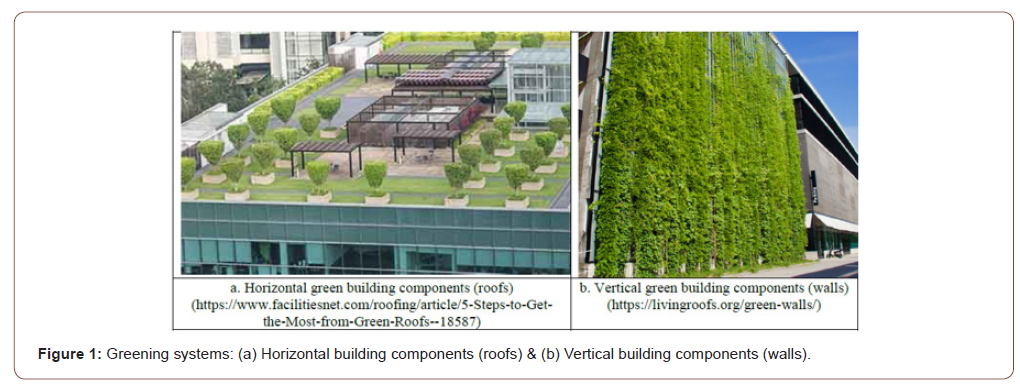
Urban areas are characterized by a lack of land, and in response to this, in some densely built-up areas, the vertical green strategy has been introduced to increase the area of green space following the requirements of international regulations. Vertical green systems can be made in two technologies, loadbearing green walls and green facades (Figure 2). Both systems have the advantage of optimizing the energy consumption of buildings [7] and increasing thermal comfort [8]. Scientific debates about the benefits of greening systems for the environment are complex and varied. Some researchers believe that vegetation attenuates the effect of heat islands both on an urban scale and on the building. Other researchers argue that green roofs are no better than other cold roofs, such as white roofs, when it comes to the heat island effect [9]. Also, other criticisms refer to the aspects related to plant species that can be used on the green roof, which must be resistant and be able to survive winter conditions, where temperatures drop well below zero degrees Celsius.

However, the presence of greening systems in the built environment certainly ensures an improvement in air quality through the process of photosynthesis, which is largely responsible for producing and maintaining the oxygen content of the Earth’s atmosphere and provides all organic compounds and most of the energy needed for life on Earth [10]. In the process of photosynthesis, plants transform water and carbon dioxide into large molecules such as glucose, cellulose, etc., and the chemical reactions of the formation of these substances are endothermic [11]. This explains the important role of plants in reducing carbon dioxide and dispersing pollutants into the atmosphere, as well as in reducing local temperature or reducing rainwater runoff.
Investigating the mechanisms of the mutual relationship between the built environment and the environment, aim to analyse the energy consumption of buildings, the use of renewable energy resources, as well as indoor and outdoor thermal comfort and air quality. Quantifying the causes and prospects of mitigating negative effects leads to technologies with the potential to improve thermal comfort and reduce carbon dioxide emissions, with substantial interventions in the architectural and structural configuration of buildings.
In the construction sector, designers and policymakers are stimulated to seek effective strategies for the sustainable development of buildings, to avoid the consumption of natural resources, and solve environmental problems. Greening systems are considered a promising solution to make buildings meet sustainability requirements, but their realization in the set of structural components of the constructions conducts to additional loads that modify the behavior of the building to various actions, which impose, in addition to the increased costs, appropriate design methods. Therefore, the main purpose of this paper is to present a short analysis of terrace-type green roofs in terms of their impact on the strength and stability design of buildings.
The scientific literature presents various research on green roofs, research on the species used, the plant substrate, and the performance of this structure on various climatic parameters [12- 14]. It is also noted that the study on the influence of green roof structure on the parameters that define the action of the wind is an important part of the research on these structures, this study being the main object and will be detailed in the next chapters.
The Evaluation of Wind Force in Structural Design
As the wind blows against a building, the resulting force called the “wind load” [15], must be taken up by the building’s strength structure and transferred to the foundations to avoid structural collapse. Wind loads depend on wind speed, shape, and volume of the building and therefore can be difficult to accurately predict. The norm Eurocode 1: Actions on structures - Part 1 - 4: General actions - Wind actions [16], gives guidance on the determination of natural wind actions for the structural design of the building and civil engineering works for each of the loaded areas under consideration. This includes the whole structure or parts of the structure or elements attached to the structure. The wind forces for the whole structure or a structural component should be determined by calculating forces from surface pressures or by calculating forces using force coefficients.
The wind force, Fw, acting on a structure or a structural element may be determined by vectorial summation of the forces Fwe, Fwi and Ffr calculated from the external and internal pressures and the frictional forces resulting from the friction of the wind parallel to the external surfaces [16], according to equation (1):

The design standard [16] prescribes a second methodology to determine the wind force Fw acting on a structure or a structural component, directly by using force coefficients as in expression (2):
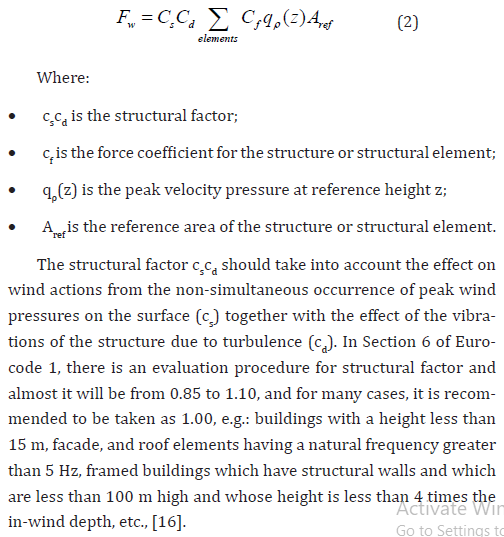
The force coefficient cf of structural elements of rectangular section with the wind blowing normally to a face should be determined by equation (3):

Where:
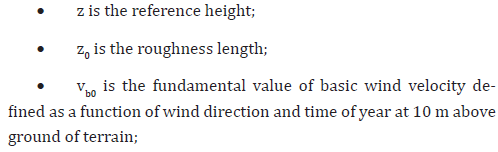
• ρ is the air density, 1.25 kg/m3.
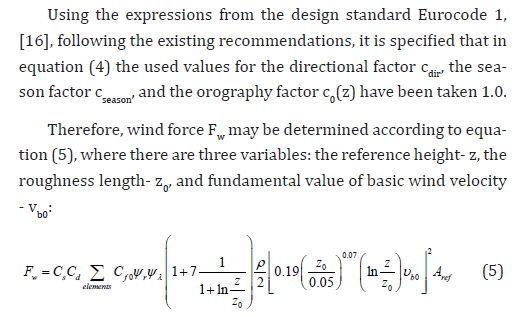
Analysing the structure of equation (5) it can be seen that the force given by the action of the wind is composed of the force from the vertical structural elements, respectively from the walls, as well as by the force at the roof level. Therefore, to evaluate the influence of the green terrace roof, the wind force at the roof level is defined according to equation (6):

The computation form of the equation (6) will allow, in the next chapter, to identify the influence of the green terrace roof on the wind force only at the roof level, considering that the force component given by the wind action on the vertical element is the same regardless of the type of terrace roof.
The Influence of Green Terrace Roof on Wind Force – Case Study
The case study was selected due to the need to improve the environment in crowded urban areas, respectively in residential areas where buildings usually have a height of about 15 m. This situation is characteristic of old residential areas with multi-store collective housing. Urban rehabilitation in these areas must take into account some specific features of these buildings, which, on the one hand, cannot be demolished given the legal situation regarding property rights, and on the other hand suffer the most from environmental pollution by lack of green areas and the impossibility of extending them to ground level. In this situation, modernization solutions to improve the quality of the environment are related to the transformation of traditional terrace roofs into green terrace roofs.
The following analysis was made on a parallelepiped-shaped building model, which keeps its cross-sectional area constant, but where the number of floors is variable and consequently the height of the building is variable. The reference area at the roof level, Aref,roof, has been considered equal to the cross-sectional area, regardless of the height at which it is from the ground. In the case study, the reference area at the roof level is assumed to be equal to 500 m2 (b = 20 m and d = 25 m). Taking also into account the recommendation given in Eurocode 1 that for all elements without free-end flow and no round corners the value 1.0 is given for ψr and ψλ, then the value of cf0 is determined using the following equation, (7):
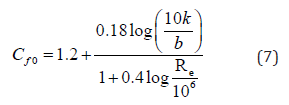
Where:
• k is the equivalent surface roughness which is equal to 0.2 mm for the traditional roof covering and may have the assumed value of 3.0 mm in the case of green roof covering;
• Re is the Reynolds number determined with the peak velocity pressure qρ(z), eq. (4), and
• with a diameter c of an equivalent cylinder having the volume equal to the parallelepiped shaped building model:
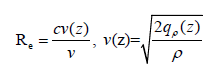
• ν is the kinematic viscosity of the air (15·10-6 m2/s).
The roughness length - z0 depends on the roughness of the site terrain and it is considered equal to 0.05 (terrain category II). Making some partial substitutions in the equation (7), it is obtained the expression (8) for the force coefficient:
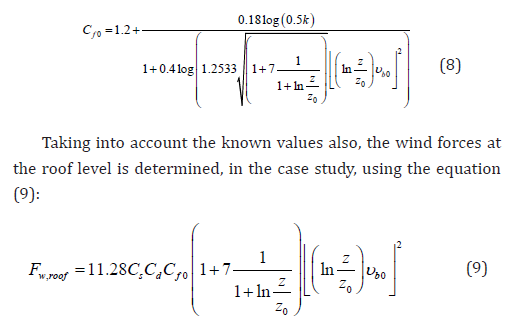
According to equation (9) the wind force at roof level, Fw,roof, has three variables: the force coefficient -cf0, the reference height -z, and the fundamental value of basic wind velocity -vb0. The recommended value for the structural factor cscd is equal to 1.0, therefore this value was used in the analysis. The results obtained for the force coefficient, cf0, are presented in Figures 3 & 5 related to the reference height - z and the fundamental value of basic wind velocity -vb0. In Figure 3, the variation of the force coefficient is evaluated for the equivalent surface roughness of the traditional terrace roof, k = 0.2 mm, while in Figure 5. is considered the equivalent surface roughness value for the case of green terrace roof, k = 3 mm.
Using the force coefficients evaluated for the two equivalent surface roughness values, the wind forces at the roof level can be evaluated. Thus, Figure 4 shows the graph of wind force depending on the variation of the reference height -z and the variation of the fundamental value of basic wind velocity - vb0, for the traditional terrace roof. Figure 6 shows the graph of wind force variation for the green terrace roof.
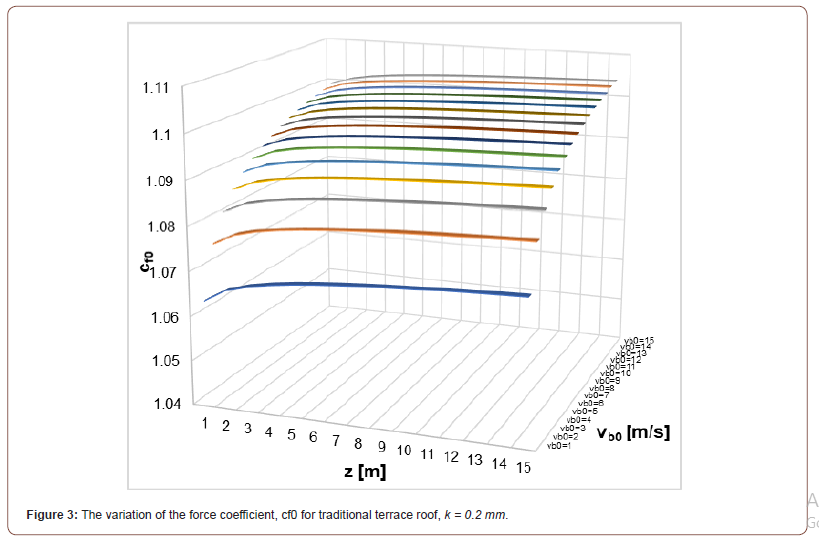

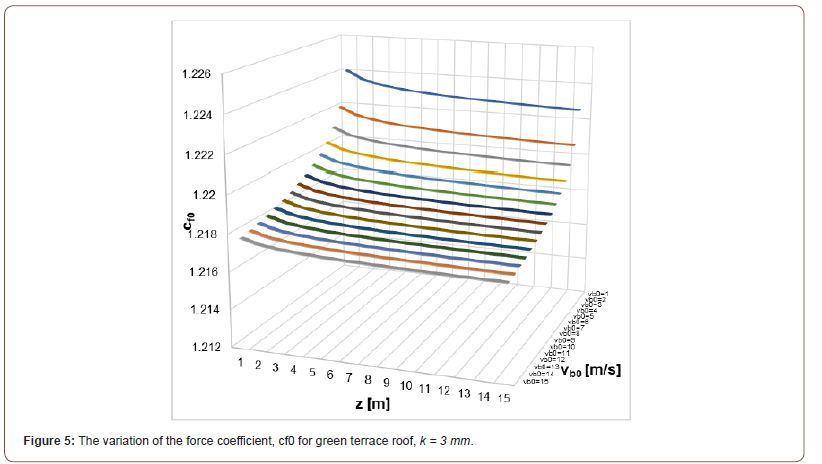

Discussions
From the results obtained in the case study analysed, the following observations can be formulated:
• The value of the equivalent surface roughness, k, influences differently the force coefficient, cf0, when the fundamental value of basic wind velocity, vb0, increases, so for k = 0.2 mm the value of the force coefficient increases with increasing wind velocity (Figure 3), while for k = 3 mm the value of the force coefficient decreases when the wind velocity increases (Figure 5);
• The same phenomenon occurs when the reference height, z, increases, so for k = 0.2 mm the value of the force coefficient increases with the increase of the reference height (Figure 3), while for k = 3 mm the value of the force coefficient decreases when the reference height increases (Figure 5);
• The wind force, Fw,roof, at the roof level increases with the increase of the reference height and the increase of the fundamental value of basic wind velocity, Figure 4 and Figure 6;
• The wind force for green terrace roof increased by approximately 20% when the equivalent surface roughness of k = 3 mm, Figure 6, compared to the value obtained for the case of k = 0.2 mm, Figure 4;
• For values of the fundamental basic wind velocity lower than 5 m/s, even if the reference height increases, there are non-essential differences between the wind force evaluated for traditional terrace roof and for green terrace roof;
• In the case when the fundamental value of basic wind velocity is higher than 5 m/s and the reference height increases, the wind force values grow exponentially (Figure 7).
Conclusion
According to the methodology of evaluating the wind force at the roof level used in the case study, the following conclusions can be formulated:
• For buildings with up to four levels above the ground floor, at wind velocity of up to 5 m/s the traditional terrace roofs can be replaced with green terrace roofs without the need to evaluate the wind force at the level of the green terrace roof;
• The wind force at the roof level is influenced by the type of terrace roof, so for a reference height of 15 m and a speed of 15 m/s, the force increases compared to the initial value by approximately 20% when the roof is a green terrace;
• Considering the exponential way of increasing the wind force at reference heights of over 15 m, it is recommended to check the behaviour of the building with a green terrace roof to the action of the wind when building height is higher than 15 m;
• By the method presented synthetically in equation (2) it is not possible to identify at the roof level if it is suction or pressure, this detailing can be identified by the analytical model presented in equation (1). It is important to know the direction of peak velocity pressure qρ(z) at reference height, to design the execution details to meet the requirements of conforming behaviour of the building in operation, to ensure the strength and stability of the structure, and its parts;
• Considering the 20% increase of the wind force and implicitly of the peak velocity pressure for high buildings, in the areas with negative values of suction, they can cause major damages in the stability of the vegetal layer from the green roof structure, thus to change the roof solution from a traditional terrace roof with a green terrace roof must be analysed before the implementation.
Acknowledgement
None.
Conflict of Interest
No conflict of interest.
References
- Baciu IR, Isopescu DN, Țăranu N, Dascălu DM, Maxineasa SG (2019) Implementation of the systems for greening the building’s structure. Buletinul Institutului Politehnic din Iași, Fasc 2: 31-45.
- Baciu IR, Lupu ML, Maxineasa SG (2019) Principles of green roofs design. The TUIASI Doctoral International Conference, LXV 3: 63-76.
- Antrop M (2004) Landscape change and the urbanization process in Europe. Landscape and Urban Planning 67(1-4): 9-26.
- Ewing L (2008) Coastal megacities and hazards: challenges and opportunities. Journal of the American Shore and Beach Preservation Association 76(4): 36-41.
- Zakaria El Adli K, Abd El Aziz NA (2021) Greening the Urban Environment: An Integrated Approach to Planning Sustainable Cities-The Case of Greater Cairo. The Importance of Greenery in Sustainable Buildings, pp. 47-72.
- Brilhante O, Klaas J (2018) Green city concept, a method to measure green city performance over time applied to fifty cities globally: Influence of GDP, population, size and energy efficiency. Sustainability Journal 10: 1-23.
- Pérez G, Coma J, Martorell I, Cabeza LF (2014) Vertical Greenery Systems (VGS) for Energy Saving in Buildings: A Review. Renewable Sustain.able Energy Reviews Journal 39: 139-165.
- de Jesus MP, Lourenço JM, Arce RM, Macias M (2017) Green Façades and in Situ Measurements of Outdoor Building Thermal Behaviour. Building Environmental 119: 11-19.
- Sproul J, Pun Wan M, Mandel BH, Rosenfeld AH (2014) Economic comparison of white, green, and black flat roofs in the United States. Energy and Buildings 71: 20-27.
- Bryant DA, Frigaard NU (2006) Prokaryotic photosynthesis and phototrophy illuminated. Trends in Microbiology 14(11): 488-496.
- Photosynthesis (2007) McGraw-Hill Encyclopedia of Science & Technology. McGraw-Hill, ISBN 978-0-07-144143-8.
- Bailey PHJ, Currey JD, Fitter AH (2002) The role of root system architecture and root hairs in promoting anchorage against uprooting forces in Allium cepa and root mutants of Arabidopsis thaliana. Journal of Experimental Botany 53(367): 333-340.
- Getter KL, Rowe DB, Cregg BM (2009) Solar radiation intensity influences extensive green roof plant communities. Urban Forestry and Urban Greening 8(4): 269-281.
- Tuan D (2013) Full-scale wind loading on green roof systems. Thesis presented to the graduate School of the University of Florida.
- (2020) Wind Load.
- (2007) SR EN 1991-1-4: Eurocode 1: Actions on structures. Wind action.
-
Isopescu Dorina Nicolina, Maxineasa Sebastian George, Baciu Ioana Roxana. Influence of Green Terraces in Evaluating the Action of Wind on Buildings. Glob J Eng Sci. 8(3): 2021. GJES.MS.ID.000688.
-
Green terrace, Wind actions, Sustainability, Building stability, Building durability
-
This work is licensed under a Creative Commons Attribution-NonCommercial 4.0 International License.