Research Article
Sustainable Mortars with Slag for Green Facades
Todorka Samardzioska1*, Milica Jovanoska Mitrevska2 and Vesna Grujoska3
1Full Professor, Faculty of Civil Engineering, Ss. Cyril and Methodius University in Skopje, Macedonia
2Assist. Professor, Faculty of Civil Engineering, Ss. Cyril and Methodius University in Skopje, Macedonia
3PhD Student, Faculty of Civil Engineering, Ss. Cyril and Methodius University in Skopje, Macedonia
Todorka Samardzioska, Professor, Ss. Cyril and Methodius University in Skopje, Faculty of Civil Engineering, 24 Partizanski odredi blvd, 1000 Skopje, Macedonia
Received Date:February 15, 2024; Published Date:February 21, 2024
Abstract
The energy crisis in the world is intensifying again in the last years. Since buildings consume about 42% of total energy, much more than industry and transport, it is logical that research in the world should be directed towards energy-efficient buildings and materials. They reduce the workload of heating and cooling systems, thereby contributing to saving energy and money. At the same time, a comfortable and pleasant temperature and the necessary thermal comfort are provided in the buildings and facilities. In this context, research in recent years has been reduced to new light composite materials, which replace part of the aggregate or cement with vegetable or polymer materials. Also, various waste materials from the industry, such as slag or ash, as well as natural materials such as perlite, vermiculite, etc. can be used. All of them contribute to obtain lighter composites, with a lower density, and a much more favorable thermal conductivity, which makes them suitable for use in new sustainable energy efficient buildings. For the purposes of this research, different types of thermal mortar samples with slag were examined, in order to prove the physical-mechanical and thermal properties of the mortar. The actual Macedonian standards were used for the tests. Compressive strength of the thermal mortar is 1.45 N/mm2, which includes it in the CS I class of mortars, whose compressive strength ranges from 0.4 – 2.5 N/ mm2. The absorption of water leads to the conclusion that the thermal-mortar can be favorably used in external atmospheric conditions, i.e. for facades. The thermal conductivity of the tested samples is λ = 0.16 W/m·K. This makes it a better thermal insulator than classic cement mortar by almost 11 times, and by 3.6 times than gypsum mortar. By increasing the volume mass (density) of the thermal mortar, the thermal conductivity increases. Tests showed that with an increase in density by 5.56%, the thermal conductivity increased by 9.5%. Similarly, for an increase of 9.26% in the density of the thermal mortar, its thermal conductivity increased by 20.1%. Furthermore, based on the obtained results, the paper points out the possibilities of applying this type of innovative thermal insulation material, as well as the method, details and technique in construction of new and reconstruction of existing buildings.
Keywords:Thermal mortar; Slag; Thermal conductivity; Compressive strength; Energy efficient buildings
Introduction
The performance of buildings in terms of energy consumption depends on the thermal efficiency of their envelopes. If the components and materials in the envelope are not properly designed and installed, heat leakage through them will compromise the overall energy consumption. Mortar is a building material that has been traditionally used over the years and is still an important component in every building. A lot of research has been devoted to improving the properties of mortar, especially for environmental reasons, since lately a lot of attention has been paid to climate change and energy efficiency. Thermal mortars can significantly contribute to solving this deficiency and represent a promising solution [1].
The development of thermal insulation mortars is a recent trend in the market, which aims to reduce the U-value of the building envelope with an upgraded thermal resistance of its components. Usually, thermal insulation mortars are used on existing substrates, such as: bricks, brick and stone walls or walls that are already mortared. The workability of thermal mortars is very similar to traditional ones, they can be used on uneven, varied or even curved surfaces. Thus, thermal insulation mortars are flexible and can be suitable for all kinds of architectural and design solutions. It is common to use materials with low density and high porosity as a substitute for natural fine aggregate in mortar mixtures, in order to achieve better thermal properties. Cork, expanded clay, expanded polystyrene, silica aerogel, as well as fly ash, slag, waste rubber and waste glass are some of the materials that can be used to improve the thermal insulation properties of mortars.
Significant number of researchers around the world investigated in this field. Since sustainable development is of vital importance, the focus of research is especially on waste materials as an integral part of the mortar mix. Sikora et al. [2] experimentally investigated the thermal properties of three types of mortars with complete replacement of the fine aggregate with waste glass with granulation up to a maximum of 2 mm and with nanosilicate, respectively 0%, 1% or 3% of the weight. The water-cement factor (w/c) was 0.5. The mortar containing waste glass showed about 2.5 times lower thermal conductivity compared to the mortar with river sand. Fadiel et al. [3] investigated the impacts of using shredded waste rubber in three different sizes (mesh 30 with an aggregate fraction of 0 ÷ 0.6 mm and mesh 10 ÷ 20 with 0.84 ÷ 2 mm). The aggregate was replaced with chopped waste rubber with 10, 20, 30 and 40% of its quantity. The results of the heat flow measurement showed that the higher percentage of chopped rubber reduces the thermal conductivity value. The value is 0.593 ÷ 0.492 W/ m·K or a reduction of 28% compared to traditional mortar. Afonso [4] presented results for four different thermal mortar formulations with different aggregate proportions: 60% aerogel + 40% expanded clay, 60% aerogel + 40% cork, 100% expanded clay and 100% cork. The attached results showed that λ = 0.08 W/m·K for the first, second and fourth formulation, and for the third formulation λ = 0.07 W/m·K. Improvement of the thermal properties is also shown by the experiment of Saha et al. [5] with iron and nickel slag. Namely, the thermal conductivity of the classic mortar decreased from 2.34 W/m·K to 1.65 W/m·K and 1.16 W/m·K when replacing 50% or 100% of the fine aggregate with slag, respectively. All these results show an important improvement in the properties of thermal mortars compared to traditional mortar with sand aggregate.
Slag, on the other hand, is waste from the metal industry. It is obtained during the processing of metals, i.e. when melting metals in special furnaces. During smelting, when the ore is exposed to high temperatures, these compounds separate from the molten metal and are thus removed. Slag is a collection of compounds that are removed from metals. It is produced in large quantities in pyro metallurgical processes and represents a huge source of waste if not recycled and used properly. Republic of Macedonia is also struggling with this problem. There are whole hills of unusable slag in the plants from “Zhelezara”, “Skopski leguri”, “Topilnica Veles” etc., which has to be transported to landfills, and is a nondegradable material.
One of the safety steps that can be taken is the repurposing of this waste material in the thermal mortar manufacturing industry. The most important aspect to consider is that slag thermal mortars use slag as a substitute for mineral aggregate. By increasing the use of slag in mortars, a large part of the industrial waste would be solved. Indian scientists Humam & Siddique [6] conducted significant research on the properties of mortar containing slag. Ordinary Portland cement was used in this research. They concluded that the compressive strength and the splitting tensile strength of the mortar increase with an increase in the amount of slag. Nataraja and Rajeeth [7] performed research on the possibility of using granulated copper slag as a substitute in cement mortar. The strength, durability and abrasion resistance of mortar under different water-cement factors have been studied and discussed. Results showed that the use of copper slag as a fine aggregate in cement mortar is appropriate and technically feasible. Borhan and Janna [8] studied the possibility of using aggregate from waste aluminum (waste from the production of structural elements) as a partial replacement of natural sand in cement mortar and found that the thermal conductivity decreases with an increase in the quantity of aluminum waste. Bae et al. [9] in their recent research investigated cement mortars with blast furnace slag powder (BFSP) and mixed steel fine aggregates, to reduce the environmental damage caused by extraction of natural aggregate and to increase the recycling rate of by- products of steel in the construction industry. Hasan El-Chabib [10] presents results of experimental research of the mechanical properties of mortar, in which the fine aggregate (sand) was partially replaced by ground granulated slag from blast furnaces by seven different percentages (10%, 20%, 30%, 40%, 50%, 60% and 70%) of slag by mass. Furthermore, according to Cerulli et al. [11] mortars containing a higher amount of iron slag have higher durability and capacity.
Materials and Methods
In this paper, the thermal properties of mortars with addition of slag, as a partial replacement of fine natural aggregate, are examined. Slag is a residue from the processing of metals and its use in mortars and concrete is increasingly common. Slag used in this research is waste from the production process in metal industry in Macedonia. It usually ends up in a landfill where it is destroyed, which results in a release of many harmful gases that have a negative impact on the environment.
Thermal propertied of mortars
Thermal conductivity can be defined as a property of the material to conduct heat through itself at a certain temperature difference between its two opposite surfaces. This property is characterized by the thermal conductivity λ. The lower the value of the coefficient λ, the better thermal insulation of the material. Testing of the thermal conductivity was performed in the Laboratory of insulation materials and building physics at the Faculty of Civil Engineering in Skopje, using the instrument for measuring thermal conductivity Heat Flow Meter (HFM) 436/3, presented in Fig. 1 and the appropriate Q-LAB software, [12]. The instrument configuration and the testing procedure is described in detail in [13]. The heat flow measurement method is a standardized test technique. The application of thermal insulation materials is related to the following standards: MKS ISO 8301:2016 [14], MKS EN 12667:2009 [15] and MKS EN 12939:2009 [16] (Figure 1).
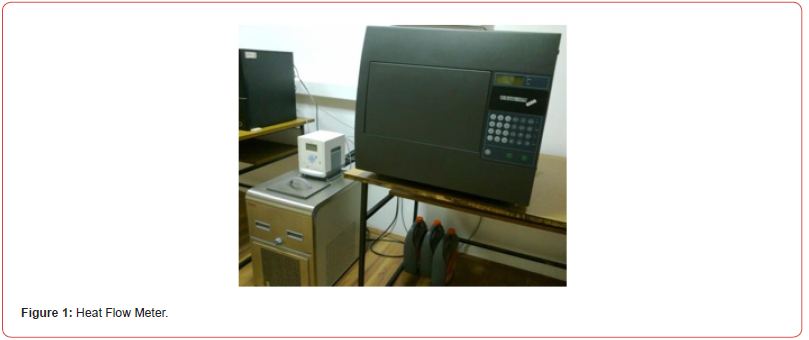
Table 1:Percentage share of the ingredients in the thermal mortar.

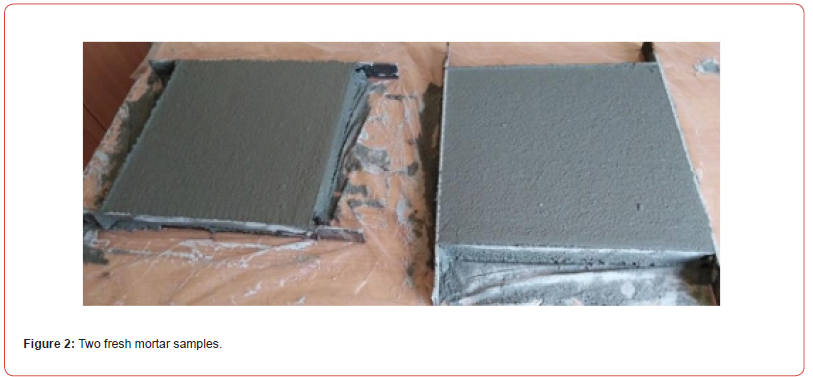
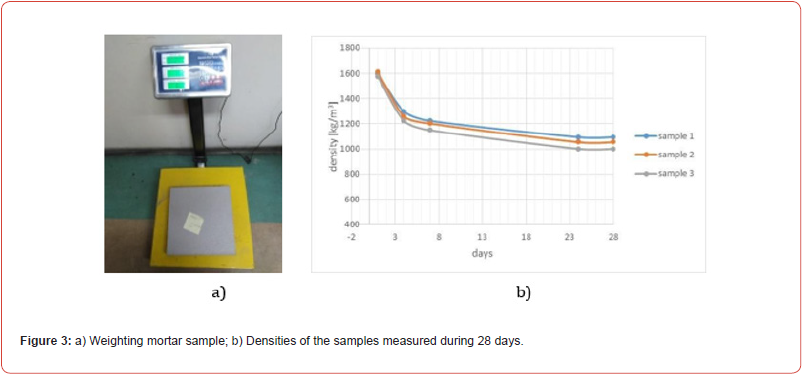
Preparation of samples
The thermal mortar was prepared with the ingredients according to the recipe given in Table 1. Three samples with dimensions of 300x300x30 mm were prepared, for which drying a standard procedure was applied. Volume and density were calculated for all samples, based on the measured masses and dimensions (Figure 2). Achieving a constant mass of the samples is done by drying them at a temperature of 70°C ± 5°C. The sample is determined to have a constant mass if two consecutive measurements within a period of 2 hours during drying do not differ by more than 0.2% of the mass of the dry sample. The mass of the sample is measured with an accuracy of 0.1%, Figure 3a. Densities are calculated as a quotient of the mass and the volume of each of the samples. These three samples have densities as follows: 1092.6 kg/m3, 1055.6 kg/ m3 and 1000 kg/m3. The average density of the dry samples is = 1049.4 kg/m3.
Light mortars usually have a density in the hardened state up to 1500 kg/m3, while, for comparison, the volume mass of ordinary mortars ranges from 1800 - 2200 kg/m3. Therefore, this thermal mortar is 1.7 - 2.1 times lighter than ordinary mortar, while it is 1.5 times lighter than usual light mortars. The diagram in Figure 3b shows the drying speed of the three samples of mortar during 28 days, until a constant specific mass was reached. The thermal conductivity for all three samples was tested at 28 days of age in the same thermo-hygrometric conditions in the laboratory of the Faculty of Civil Engineering in Skopje, Figure 4.
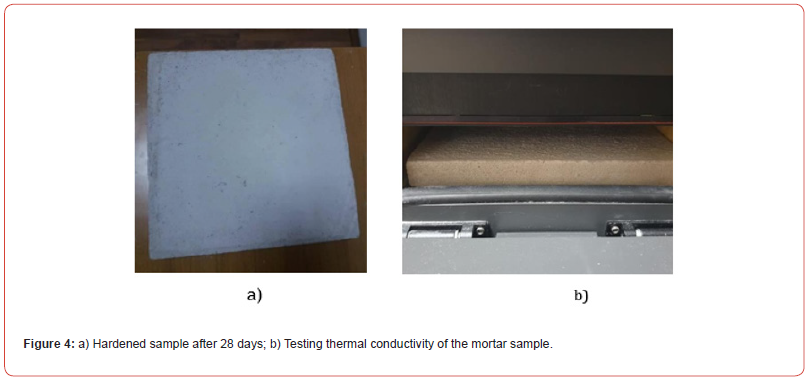
Porosity of the mortars
The amount of entrained air in the mortar depends on several factors – the percentage of aggregate dosage, the type of concrete or mortar, the type and amount of cement, the maximum fraction of aggregate, the consistency of the mortar. Through the entrained air, it is determined how porous the material is, and many other characteristics arise from this property. The tested samples of the thermal mortar with slag gave a result of 42% entrained air, while the normal mortars show results of 25-35% entrained air. Through this test, it can be concluded that the considered mortar has a higher porosity, which results in a higher thermal insulation ability.
Mechanical properties
In order to determine the bending strength of thermal mortar, test samples of hardened mortar in the form of prisms with dimensions of 40/40/160 mm and an age of 28 days were examined. The test result is obtained as the mean value of at least three samples, following the guidelines in the standard MKS EN 1015- 11 [17] and its value is fs = 0.73 N/mm2. Samples according to the MKS EN 1015-11 standard were used to test the compressive strength, [17]. They were tested in a press. The result of the compressive strength test is fc=1.45 N/mm2. The compressive strength of class CS1 mortar in the hardened state ranges from 0.4 - 2.5 N/mm2, which means that the considered mortar has a compressive strength within the limits of class CS1 mortar.
Water absorption
The standard MKS EN 1015-18: Test methods for masonry mortar - Part 18: Determination of water absorption coefficient as a result of capillary action of hardened mortar, [18], is used to test the water absorption of thermal mortar. After the samples were prepared, they were placed in a container of water with a height of 5 – 10 mm, in order for the water to rise by capillary action in them, keeping the water level constant. The samples were covered to prevent possible evaporation of water from them. By measuring the mass of the examined sample after 10 minutes (M1) and after 90 minutes (M2), the absorption of water was determined, which is C = 0.39 kg/m ∙ min 0.5. This result shows that this type of thermal mortar belongs to class W1 < 0.4 kg/m2 ∙ min 0.5, which means that the mortar can be used for external use. With this experiment, water penetration was measured after 10 minutes and after 90 minutes, and they were 33 mm and 40 mm, respectively.
Thermal conductivity
Results for the density, thermal conductivity, thermal resistance, as well as the test time for all three samples, with dimensions of 300× 300 × 30 mm, are presented in Table 2. The duration of the examination of each of the samples depends on the achievement of thermal equilibrium.
The results, shown in the Table 2, are compared in the following Figure 5. The first sample shows a 20.1% higher thermal conductivity than the third sample, while the second has a higher value than the third by 9.5%. During observation, it was noticed that the first sample has several pronounced depressions and unevenness on the surfaces on which the plates of the thermal conductivity instrument rest, so its higher lambda value may result due to that anomaly. The average value for the thermal conductivity of the thermal mortar was calculated as λaverage= 0,15956 ≈ 𝟎, 𝟏𝟔 W/m · K, as shown with the red column in Fig. 5.
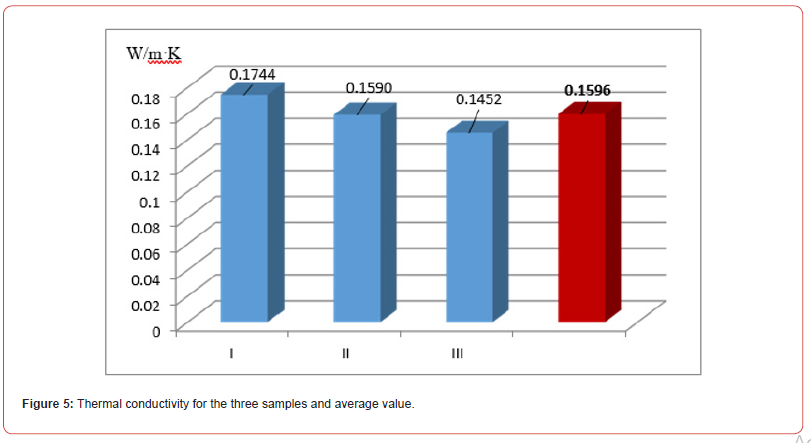
Table 2:Thermal conductivity, thermal resistance and test time of the samples.

The obtained value for thermal conductivity of 0.16 W/m·K shows that the slag mortar has significantly improved thermal insulation characteristics compared to classic mortars. Namely, since cement mortar usually has thermal conductivity λ = 1.73 W/m·K, and gypsum mortar has thermal conductivity λ = 0.57 W/m·K, with this research it is shown that thermal mortars with slag have as much as 11 times lower thermal conductivity than classic cement ones, and 3.6 times lower conductivity than gypsum mortars. But since gypsum mortars are not recommended for external use, it follows that the examined thermal mortar has a much greater potential use.
On the other hand, the value of 0.16 W/m·K is only 4 times greater than the thermal conductivity of traditionally used thermal insulation materials (nearly 0.04 W/m·K for stone wool, glass wool, expanded polystyrene etc.). That means that sufficiently thick mortar layer can substitute a part of the thermal insulation, with lower costs and lower impact on the environment.
Thermal resistance
The thermal resistance R represents a reciprocal value of the thermal conductivity of the material. The obtained values for the three examined samples are shown in Figure 6. The average value of the three samples of the thermal mortar shows that the thermal resistance is R = 0.199312 ≈ 0.2 m2K/W. The Figure 7 shows the relationship between the mortar density on the thermal conductivity. With an increase in density of 1000.0 kg/m3 for the third sample, 1055.6 kg/m3 for the second and 1092.6 kg/m3 for the third sample, it is noticeable that the thermal conductivity increases. Namely, it is common for the majority of construction materials, that with the increase in density, the porosity of the materials decreases. With this, the definition that the thermal conductivity is inversely proportional to the porosity is also confirmed for this type of material, i.e. the decrease in porosity influences an increase in thermal conductivity.
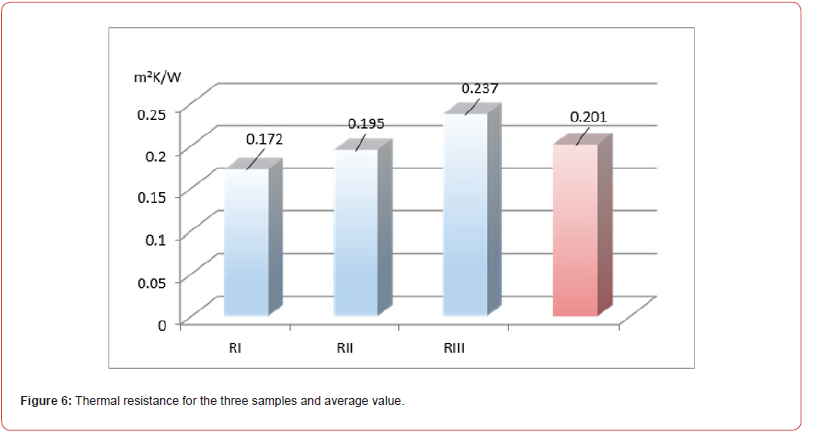
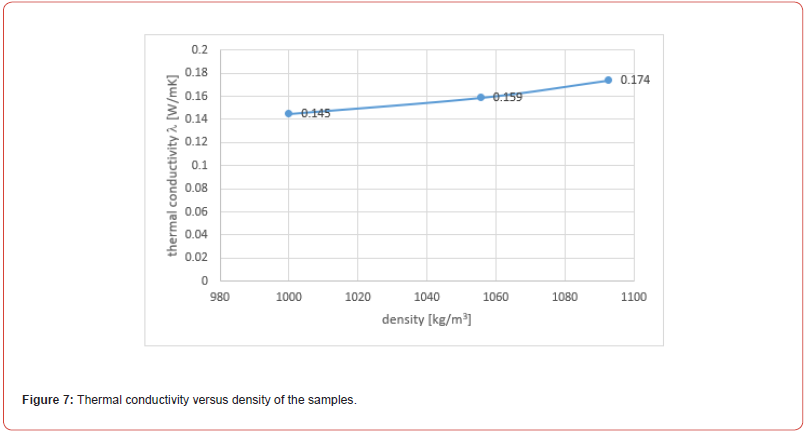
The density of the second sample is 5.56% higher than that of the third one, and the thermal conductivity increased by 9.5%. The first sample, on the other hand, has a 9.26% higher density than the third one, while the thermal conductivity increased by 20.1% compared to the conductivity of the third sample.
Application of thermal mortars in buildings
The favorable properties of these new thermal mortars offer
possibilities for the multi-purpose use for the new buildings, as
well as for reconstruction of existing buildings. Since there is no
need of selection of specially trained staff for work and no need of
specific technique and skills for performance technology, thermal
mortar with slag can be used wherever classic mortars can be used,
for instance:
a. Finishing of building facades and provision of thermal
insulation;
b. Additional insulation of external and internal walls of
buildings;
c. Insulation of hot and cold-water supply pipes;
d. Insulation of floors and ceilings, etc.
Conclusion
The amount of waste slag and its presence in the environment occupies a large percentage of the total waste. Steel slag is very difficult to dissolve in water. It represents one of the biggest environmental problems in the world because it cannot be recycled, processed, repurposed and decomposed. Most types of slag also contain hazardous elements such as As, Pb, Cd, Co, Cr and Ni. These substances can be released from the slag to a certain extent leading to environmental hazards. A large body of scientific findings and evidence is available on the pollution of water, soil and plants by waste slag. Although slag can be repurposed, it still leaves long-lasting traces in nature. In order to recycle these hazardous substances, it is necessary to take long-term safety steps in the name of the health of future generations. Repurposing of this waste material as a substitute for mineral aggregate in the thermal mortar is an elegant way to provide safety solutions for the environment.
In this research, the thermal properties of a new type of mortar
with the addition of slag were tested. It showed significant favorable
physical, mechanical and thermal characteristics:
a) In accordance with the tests of the physical and mechanical
properties of the thermal mortar, as well as according to the
obtained results of water absorption, it is established that it can
be used for internal as well as external mortaring. The obtained
compressive strength of 1.45 N/mm2 shows that it is in the
class of CS I class, with a compressive strength of 0.4 – 2.5 N/
mm2.
b) The thermal conductivity of the tested samples is λ =
0.16 W/m·K. The value obtained in this way shows that the
slag mortar has significantly improved thermal insulation
characteristics compared to classic mortars. Namely, since
cement mortar usually has thermal conductivity λ = 1.73
W/m·K, and gypsum mortar has thermal conductivity λ = 0.57
W/m·K, with this research it is shown that thermal mortars
with slag have as much as 11 times lower thermal conductivity
than classic cement ones, and 3.6 times lower conductivity
than gypsum mortars. But since gypsum mortars are not
recommended for external use, it follows that the examined
thermal mortar has a much greater potential use.
c) The thermal conductivity of the thermal mortar is directly
proportional to the bulk mass (density), i.e. by increasing
the volumetric mass of the thermal mortar, the thermal
conductivity increases. Tests showed that with an increase in
density by 5.56%, the thermal conductivity increased by 9.5%.
While for an increase of 9.26% in the volumetric mass of the
thermal mortar, its thermal conductivity increased by 20.1%.
d) These properties offer possibilities for the multi-purpose
use of the new thermal mortar product for the new buildings
and, especially, for the reconstruction of existing buildings.
e) Thermal mortar with slag can be used wherever classic
mortars can be used, without selecting specially trained staff
for work and without specific technique and performance
technology.
f) This type of thermal mortar represents one of the greatest
environmental discoveries, as it gives hope for solving one of
the biggest environmental problems in the world. In this way,
slag as a waste material would be recycled and converted into
a useful product and an essential element in the construction
industry.
Acknowledgment
The research work has been carried out under the support of the Faculty of Civil Engineering, Ss. Cyril and Methodius University in Skopje. Testing materials were provided as a part of the project “Ecological mortars for green facades”, financed by FITR—Fund for Innovation and Technology Development of R. Macedonia.
Conflict of Interest
No conflict of interest.
References
- Barbero S, Dutto M, Ferrua C, Pereno A (2014) Analysis on existent thermal insulating mortars towards innovative applications: Evaluation methodology for a real cost performance, Energy and Buildings 77: 40-47.
- Sikora P, Horszczaruk E, Skoczylas K, Rucinska T (2017) Thermal properties of cement mortars containing waste glass aggregate and nanosilica; Procedia Engineering 196: 159-166.
- Fadiel A, Al Rifaie F, Abu Lbdeh T, Fini E (2014) Use of crumb rubber to improve thermal efficiency of cement-based materials, American Journal of Engineering and Applied Sciences 7(1): 1-11.
- Afonso P (2015) Acoustic behavior of mortars with improved thermal performance. Instituto Superior Tecnico, Universidade de Lisboa, Portugal.
- Saha AK, Sarker PK, Golovanevskiy V (2019) Thermal properties and residual strength after high temperature exposure of cement mortar using ferronickel slag aggregate; Construction and Building Materials 199: 601-612.
- Humam T, Siddique R (2013) Properties of Mortar Incorporating Iron Slag; Leonardo Journal of Sciences (23): 53-60.
- Nataraja MC, Rajeeth TJ (2019) Strength, Flow and Abrasion characteristics of cement mortar produced from copper slag. Journal on Structural Engineering 8(1).
- Borhan MT, Janna H (2016) Thermal properties of cement mortar containing waste aluminium fine aggregate. Journal of Kerbala University 14(2).
- Cerulli T, Pistolesi C, Maltese C, Salvioni D (2003) Durability of traditional mortars with respect to blast furnace slag-based mortar. Cement and Concrete Research 33 (9): 1375-1383.
- Bae SH, Lee JI, Choi SJ (2021) Characteristics of Mortars with Blast Furnace Slag Powder and Mixed Fine Aggregates Containing Ferronickel-Slag Aggregate. Materials 14(19): 5879.
- El Chabib H (2020) Properties of SCC with supplementary cementing materials“in Self-Compacting Concrete: Materials, Properties and Applications. Science Direct Journals and Books.
- (2008) Heat Flux Meter 436/3 Lambda, Operating Instructions, NETZSCH-Gerätebau GmbH.
- Samardzioska T, Jovanosk -Mitrevska M, (2023) Recycled Rebonded Polyurethane Foam as Sustainable Thermal Insulation. Current Trends in Civil & Structural Engineering 9(4).
- MKS ISO 8301 (2016) Thermal insulation- Determination of steady-state thermal resistance and related properties-Heat flow meter apparatus.
- MKS EN 12667 (2009) Thermal performance of building materials and products - Determination of thermal resistance by means of guarded hot plate and heat flow meter methods - Products of high and medium thermal resistance.
- MKS EN 12939 (2009) Thermal performance of building materials and products - Determination of thermal resistance by means of guarded hot plate and heat flow meter methods - thick products of high and medium thermal resistance.
- MKS EN 1015-11 (2019) Methods of test for mortar for masonry - Part 11: Determination of flexural and compressive strength of hardened mortar.
- MKS EN 1015-18 (2022) Methods of test for mortar for masonry - Part 18: Determination of water absorption coefficient due to capillary action of hardened mortar.
-
Todorka Samardzioska*, Milica Jovanoska Mitrevska and Vesna Grujoska. Sustainable Mortars with Slag for Green Facades. Cur Trends Civil & Struct Eng. 10(4): 2024. CTCSE.MS.ID.000741.
-
Thermal mortar; Slag; Thermal conductivity; Compressive strength; Energy efficient buildings; Iris Publishers, Iris Indexing Sites
-
This work is licensed under a Creative Commons Attribution-NonCommercial 4.0 International License.