Research Article
New Trends of the Polysaccharides as a Drug
Mohsen S Asker, Ebtsam M El Kady* and Mamal G Mahmoud
Microbial Biotechnology Department National Research Centre, Dokki, Cairo, Egypt
Ebtsam M El Kady, Microbial Biotechnology Department National Research Centre, Dokki, Cairo, Egypt.
Received Date: October 21, 2019; Published Date: November 07, 2019
Abstract
Polysaccharides (PSs) may be regarded as key ingredients for the production of bio-based materials in life sciences (medical devices, pharmaceutics, food and cosmetics). There are an enormous variety of polysaccharides that can be synthesized and/or released by marine algae. Both these marine organisms are excellent sources of PSs, most of them sulfated (S-PSs). Although some similarities may be found between the PSs from each group of organisms, they can be very heterogeneous and structurally different. The biological source and biodegradability of these biopolymers, coupled to the large variety of functionalities they encompass, make them promising compounds for the application in pharmaceuticals, therapeutics, and regenerative medicine. Some of the beneficial bioactivities demonstrated by the crude PSs and their derivatives, either in-vitro or in-vivo, include anticoagulant and/or antithrombotic properties, immunomodulatory ability, anti-tumor and cancer preventive activity. They are also good antilipidemic and hypoglycemic agents, and can be powerful antioxidants, antibiotics and anti-inflammatory. Other biomedical properties of PSs have been discussed, such as antinociceptive, angiogenic, cardioprotective, gastroprotective and hepatoprotective activities. The biomedical applications and potentialities of PSs in this area were listed, such as healing wound agents, mucobioadhesives for bone and soft tissue closure, bio lubricants to mitigate joint disorders caused by arthritis, vaccines for cancer immunotherapy, or in a new generation of bio textiles and medical fibers, in drug delivery systems, and scaffolds in regenerative medicine. From the extensive list above, the importance of this type of PSs from algae for medical use is quite obvious. However, despite all the interesting properties and potentialities for human health, the use of these PSs, especially those from algae need to be further explored. In particular, the toxicity and bioavailability of some of these polymers are yet to be studied on humans.
Background
Polysaccharides (PSs) are a high molecular weight polymer, consisting of at least ten monosaccharides mutually joined by glyosidic linkages. The glycosyl moiety of hemiacetal or hemiketal, together with the OH group of another sugar unit, formed the glyosidic linkages [1]. Unlike protein and nucleic acid, the structure of PSs is far more complicated based on the differences in (i) composition of monosaccharide residues, (ii) glyosidic linkages, (iii) sequence of sugar units, (iv) degrees of polymerization, and (v) branching point. Apart from those, other factors, such as differences of cultivars, origins, and batches, or even extraction methods and fraction procedures are evidenced to have significant influence on the physicochemical and structural properties of PSs. Owing to the rapid development of modern analytical techniques; the identification of PSs structures is becoming more and more feasible and convenient [1]. In recent years, researches have confirmed that PSs from natural products possess wide-ranging beneficial therapeutic effects and health-promoting properties. Specifically, seaweed derived PSs, such as alginate, fucoidan, carrageenan, laminaran, and agar [2], are widely distributed in biomedical and biological applications [3-7], for example, tissue engineering, drug delivery, wound healing, and biosensor due to their biocompatibility and availability.
Fungal PSs, derived from Grifola frondosa, Lentinula edodes, oyster mushroom, as well as Ganoderma, Flammulina, Cordyceps, Coriolus, and Pleurotus, and so forth, are demonstrated to have multiple bioactivities [8-11], including immunomodulating, anticancer, antimicrobial, hypocholesterolemic, and hypoglycemic effects. Bacterial extracellular PSs, loosely associated with bacterium, capsular PSs, tightly bound to bacteria surface, and lipopolysaccharides, always anchored to cell surface by lipid, are nontoxic natural biopolymers and provide extensive applications in areas such as pharmacology, nutraceutical, functional food, cosmeceutical, herbicides, and insecticides [12].
If PSs contains only one kind of monosaccharide molecule, it is known as a homopolysaccharide, or homoglycan, whereas those containing more than one kind of monosaccharide are heteropolysaccharides. The most common constituent of PSs is glucose, but fructose, galactose, galactose, mannose, arabinose, and xylose are also frequent [13,14]. PSs are structurally diverse classes of macromolecules able to offer the highest capacity for carrying biological information due to a high potential for structural variability [15]. Whereas the nucleotides and amino acids in nucleic acids and proteins effectively, interconnect in only one way, the monosaccharide units in PSs can interconnect at several points to form a wide variety of branched or linear structures [16]. This high potential for structural variability in PSs gives the necessary flexibility to the precise regulatory mechanisms of various cell-cell interactions in higher organisms. The PSs of mushrooms occurs mostly as glucans. Some of which are linked by β-(1---3), (1---6) glycosidic bonds and α-(1---3)-glycosidic bonds but many are true heteroglycans. Most often there is a main chain, which is either β-(1---3), β-(1---4) or mixed β-(1---3), β-(1---4) with β-(1---6) side chains. Hetero-β-D-glucans, which are linear polymers of glucose with other D-monosaccharides, can have anticancer activity but α-D-glucans from mushroom usually lack anticancer activity [15]. Heteroglucan side chains contain glucuronic acid, galactose, mannose, arabinose or xylose as a main component or in different combinations
The number of potential PSs structures is almost limitless but in practice many such polymers are unlikely to possess useful physical properties. Even now it is difficult to relate the chemical structure elucidated for any specific PSs to its physical functionality [17]. Currently only a small number of biopolymers are produced commercially on a large scale. However, this limited group of products exhibits an extensive range of physical properties and also provides several models for study by microbiologists, carbohydrate and physical chemists and molecular biologists.
PSs widely exist in the animals, plants, algae and microorganism. Together with proteins and polynucleotides, they are essential biomacromolecules in the life activities and play important roles in cell–cell communication, cell adhesion, and molecular recognition in the immune system [18]. In recent years, some bioactive PSs isolated from natural sources have attracted much attention in the field of biochemistry and pharmacology. They exhibit various biological activities affected by different chemical structures. Suarez et al. [19] reported that the immunostimulatory activity of arabinogalactans extracted from Chlorella pyrenoidosa cells depended on their molecular weights. The higher molecular weight arabinogalactans exhibited immunostimulatory activity, but the lower molecular weight fractions did not. Further researches show that the activities of PSs are not only dependent on their chemical structures, but also are related to their chain conformations [20]. It is known that the anti-tumor activities may be related to the triple helical conformation of the β-D-(1---3)-glucan backbone chain for some PSs, such as lentinan from Lentinus edodes [19,21] and schphylizophyllan from Schizophyllum commune [22,23]. In view of the fact that, Sakurai and Shinkai [24] were the first to find that schizophyllan may form a helical complex with single stranded homopolynucleotides, many works about preparing a complex of schizophyllan and DNA or RNA for a nontoxic gene delivery system have been developed [25-32]. Generally, it is interesting and important to elucidate the relation among chemical structures, chain conformations of PSs and their biological activities. However, PSs are usually composed of various monosaccharides linked with different glucoside bonds. Some PSs has hyperbranched structures. Moreover, PSs often has high molecular weights, and tends to form aggregates in solution that can mask the behavior of individual macromolecules. In consequence, to characterize the chemical structures and chain conformations of PSs is not an easy task. This article attempts to review the current structural and conformational characterization of some importantly bioactive PS isolated from natural sources (Figure 1).

The chemical structures were analyzed by FTIR, NMR, GC, GC– Mass and HPLC. The chain conformations of PSs in solutions were investigated using static and dynamic light scattering.
PSs already proved to have several important properties [33- 43]. However, the attempts to establish a relationship between the structures of the PS and their bioactivities/actions have been a challenge due to the complexity of this type of polymers. In fact, aside from the homogalactan from Gyrodinium impudicum [44], the β-glucan from Chlorella vulgaris [45] and the PSs from a few species of algae, most of these carbohydrates are highly branched hetero polymers with different substituents in the various carbons of their backbone and side sugar components. Additionally, the monosaccharide composition and distribution within the molecule, and the glyosidic bonds between monosaccharides can be very heterogeneous, which is a real impairment for the study of their structures. Moreover, this heterogeneity also depends on the species, between strains of the same species, and on the time and place of harvest. Nevertheless, there are always some similarities between the PSs from each group of seaweeds: often, fucoidans are extracted from brown algal species, agaroids and carrageenans come from red algae, and ulvans are obtained from green algae. Regarding cyanobacteria and as far as we know, there are not common names for their PSs, to the exception of spirulan from Arthrospira platensis. There are species that, besides producing large amounts of these useful polymers, they secrete them out into the culture medium and these polymers are easily extracted [38]. Both algae are cyanobacteria excellent sources of PSs, most of them being sulfated (S-PSs). They are associated with several biological activities and potential health benefits, making them interesting compounds for the application in pharmaceuticals, therapeutics, and regenerative medicine. Some of the beneficial bioactivities demonstrated by the crude PSs and their derivatives, either in-vitro or in-viv, upon various kinds of cell-lines and animal models, include anticoagulant and/or antithrombotic properties, immunomodulatory ability, anti-tumor and cancer preventive activity. They are also good antidislipidaemic and hypoglycaemic agents, and can be powerful antioxidants, antibiotics and antiinflammatory. The S-PSs from Enteromorpha and Porphyridium have demonstrated strong antitumor and immunomodulating properties [46-48] those from Caulerpa cupressoides and Dyctiota menstrualis are good antinociceptive agents [49,50], and the S-PSs from Cladosiphon okaramanus showed angiogenic, gastro- and cardioprotective bioactivities [33,51,52].
Some Structural Characteristics of Polysaccharides from Algae
The chemical structure of PSs from algae may significantly determine their properties, namely physico-chemical and biochemical, and reflect their physical behavior and biological activities.
Macroalgae
Macroalgae who’s PSs have been studied more often; belong to the group’s brown algae (Phaeophyceae) green algae (Chlorophyta) and red macroalgae (Rhodophyta). Brown algae usually contain fucoidans; the oligosaccharides obtained from the hydrolysis of fucoidans may often contain galactose, glucose, uronic acids, and/or other monosaccharides, linked together and to the main chain by different types of glycosidic bonds. This is the case, for example, for the laminaran from E. bicyclis, or the galactofucan from Sargassum sp., and the fucan from P. tetrastromatica. However, the structure complexity of these fucoidans makes difficult to establish a relationship between the PS-chains/composition and their biological actions, and/or some kind of protocols to design universal pharmaceuticals or other drug-like substances to prevent and/ or cure specific diseases [53]. The monosaccharide composition, the linkage types, the overall structure of fucoidans, and some of their di- and oligosaccharides were well explored by Li et al. [54], Ale et al. [55] and Fedorov et al. [35]. Ale et al. [55] showed the difference between S-PSs from three species of Fucus by focusing on the various substituents at C-2 and C-4 carbons, despite the similarities of their backbones; they also highlighted the possible structures of fucoidans from two species of Sargassum [56,57]. Among them are the schemes for the components of the main chain showing either the (1---3)-, and (1---3)- and (1---4)-linked fucose residues or some di- and trisaccharide repeating units for A. nodosum, C. okamuranus, L. saccharina and some species of Fucus. On the other hand, Fedorov et al. [35] focused on the structures and bioactivities of different S-PSs, such as galactofucan from Laminaria and laminarans from E. bicyclis. Red algae contain large amounts of S-PSs, mostly agaroids and carrageenans, with alternating repeating units of α-(1---3)-galactose and β-D-(1---4)-galactose [58], and/or (3---6)-anhydrogalactose [59]. Substituents can be other monosaccharides (mannose, xylose), sulfate, methoxy and/or pyruvate groups and the pattern of sulfation dividing carrageenans into different families, for example, in C-4 for κ-carrageenan, and in C-2 for λ-carrageenan. In addition, the rotation of galactose in 1,3-linked residues divides agaroids from carrageenans [60]. Apart from agarans [60], found in species of Porphyra, Polysiphonia, Acanthophora, Goiopeltis, Bostrychia or Cryptopleura are also good sources of κ-carrageenan (E. spinosa and K. alvarezii), λ-carrageenan (Chondrus sp, G. skottsbergii and Phillophora) [61], I-carrageenan (E. spinosa) [62], and other heterogalactans with mannose and/ or xylose building up their backbones. Among these, we may find xylogalactans in N. fastigiata and xylomannans in S. polydactyla [63,64].
Regarding green algae, the information on their structures and applications is scarce. Wangs et al. [41] has made an excellent overview on those properties for the S-PSs from several genera of green algae. These S-PSs are very diverse and complex, with various types of glycosidic bonds between monomers, and include galactans (Caulerpa spp.), rhamnans (C. fulvescens and Enteromorpha), arabino- and pyruvylated galactans (Codium spp.), and the most known ulvans from Ulva spp and E. prolifera. Wang et al. [41] also included some repeating aldobiuronic di-units for the backbone of ulvans, containing aldobiouronic acid or glucouronic acid (U. armoricana and U. rigida, respectively), disaccharides sulfated xylose-sulfated-rhamnose, and a trisaccharide unit composed by 1,4-linked glucouronic acid, glucouronic acid and sulfatedrhamnose. The backbone of rhamnans seems to be somewhat simpler, but other types of glycosidic bonds can also appear. Four repeating disaccharide units were indicated for the homo polymer of M. latissimum [65]. Species from Codium are very interesting: their S-PSs may include different percentages of arabinose and galactose, giving place to arabinans (C. adhaerens [66], galactans (C. yezoense) [67], arabinogalactans [41]. Pyruvylated galactans were also identified in C. yezoense [67], C. isthmocladium [68] and C. fragile [69]. Some other species of Codium present other PS-types such as β-D-(1---4)-mannans in C. vermilara [70], or the rare β-D- (1---3)-mannans in C. fragile [71], with various sulfation patterns
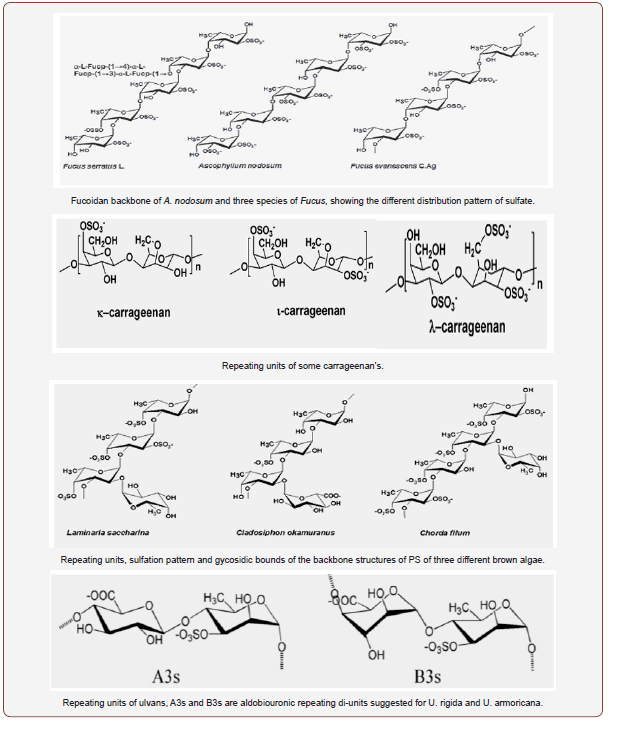
Cyanobacteria
The characteristics of the various PS produced by cyanobacteria, including their composition and structure, were recently discussed [72]. Some particular aspects about these polymers came to light. For example, it seems that concerning cyanobacteria only G. impudicum and C. vulgaris contain homopolysaccharides of galactose and glucose [44,45], respectively, while the PS from the other species are hetero polymers of galactose, xylose and glucose in different proportions. Rhamnose, fucose and fructose can also appear, and some of the PS presents uronic acids as well. The glycosidic bonds are described for only a few PS, such as the one from Aphanothece halophytica, whose monosaccharides are mainly 1,3-linked, but linkages of type 1 also appear for glucose and glucouronic acid [73], which suggests that these two last molecules are terminal, and some multiple bonds, such as 1,2,4-linked and 1,3,6-linked mannose residues [73], are present as well, suggesting some branches coming out from the backbone of the PS. Further, there are some special features of PS, as it is the case of acofriose 3-O-methyl-rhamnose in the polymers of Chlorella [74], Botryococcus braunii and calcium-spirulan (Ca-Sp) of Arthrospira platensis [75]. In Porphyridium cruentum, an aldobiuronic acid [3-O-(α-D-glucopyranosyluronic acid)-L-galactopyranose], and two hetero-oligosaccharides were also identified [76], and so did two other aldobiuronic acids [77] which were also found in other species of Porphyridium and Rhodella [19]. Furthermore, other repeating disaccharide units [78,79] and some oligosaccharides were also highlighted [79]. In addition, Ford and Percival [80] found that the structure of the S-PS from Phaeodactylum tricornutum was a ramified sulfated glucoronomannan, with a backbone composed by β-(1---3)-linked mannose; a triuronic acid, an aldobiuronic acid and a glucan made of β-(1---3)-linked glucose were also identified as being constituents of the side chains of that polymer

Potential Pharmaceutical Applications of Polysaccharides from Algae
The PSs are complex and heterogeneous macromolecules, coming from different genera belonging to the larger groups of algae, and species and strains of the same genus. Repeatedly, difficulties are found in identifying their chemical structure and therefore, their biological activities not being thoroughly understood. Few researchers have focused on such a challenging task as the exploitation of possible relation chemical structure activity of PSs. One approach to look for structure biological activity relationships has been to make inferences based on information obtained from studies of invertebrate sulfated polysaccharides that have a regular structure and, thus, could be more easily studied [60,81]. The types of glycosidic linkages and the contents and positions of the sulfate groups may be significantly different in the various S-PS, depending on species, region of the thallus, growing conditions, extraction procedures, and analytical methods [68,82]. The biological and pharmacological activities of S-PSs normally result from a complex interaction of several structural features, including the sulfation level, distribution of sulfate groups along the polysaccharide backbone, molecular weight, sugar residue composition, and stereochemistry [83,84]. For instance, the general structural features of fucans that are important in their anticoagulation activity include the sugar composition, molecular weight, sulfation level and the position of sulfate groups on the sugar backbone [85- 88]. Furthermore, it has been observed that the antiviral activity of S-PSs increases with the molecular weight [89]. Galactans, fucans and galactofucans are representative polysaccharides from brown and red algae that differ in structure, sulfation level and molecular weight, and yet all were shown to inhibit HSV-1 and HSV-2 infection [90]. Newly, by using NMR, it was found that branched fucoidan oligosaccharides might present higher imunoinflammatory activity than linear structures, because they were better at inhibiting the complement system [91]. Usov [58] compared two sulfated galactans from Botryocladia occidentalis and Gelidium crinale. He concluded that the interaction of the S-PSs with different compounds participating in the coagulation process depends on the differences in the structural features; unfortunately, data on the configuration of galactose in the galactan from G. crinale are not sufficient to fully understand the relationship.
Antiviral and antimicrobial activities
During the last decade, the number of antivirals approved for clinical use has been increased from 5 to more than 30 drugs [92]. However, as these drugs are not always efficacious or well tolerated and drug resistant virus strains are rapidly emerging, there is still a great demand for further drug development including novel modes of action. A very promising approach is the antiviral screening of products derived from natural sources, such as marine flora and fauna, bacteria, fungi, and higher plants. Among them, marine algae represent one of the richest sources of bioactive compounds, and algae-derived products are increasingly used in medical and biochemical research [93]. HIV-1 infection is a multistep process beginning with the attachment of the version to cell surfaces and entry into lymphoid target cells. In several cell types, including CD4+ helper T cells, macrophages, and dendritic cells [94,95], the virus can establish productive or persistent infection. During virus spread, HSV-1 virons are attached via their envelope gp120 glycoprotein to the CD4 surface protein of T-lymphocytes in conjunction with a chemokine (CXCR4 or CCR5) co receptor (Figure 2).
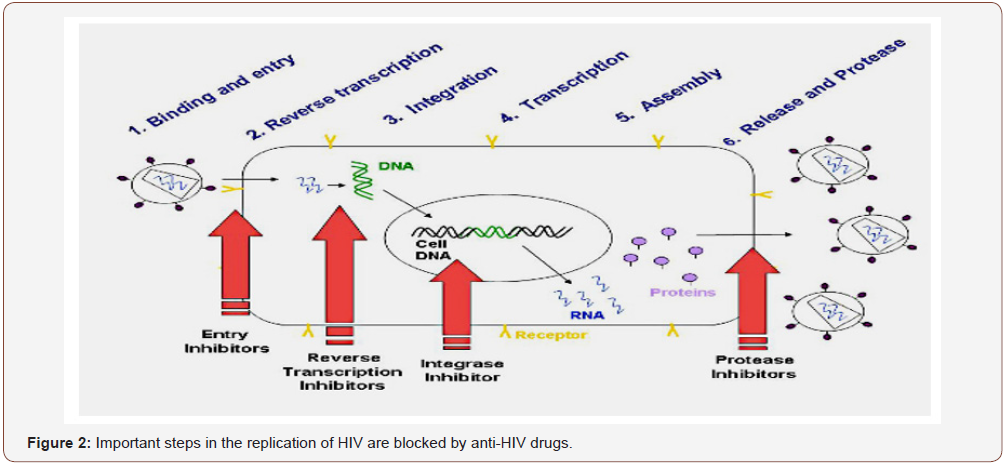
An overview on the antiviral activity against several kinds of virus and retrovirus, enveloped or naked was well documented by Carlucci et al. [96] & Wijesekara et al. [2]. These reviews focused on the HIV type 1 and type 2, the human papilloma virus (HPV), the encephalo-myocarditis virus, the hepatitis virus type A and type B and the dengue and yellow fever virus. The inhibition of infection by most of these viruses was explained by the action of S-PSs, which might block the attachment of visions to the host cell surfaces [97,98]. Another way of exerting their activity is by inhibiting the replication of the enveloped virus, such as the HIV, the human cytomegalovirus (HCMV) and the respiratory syncytial virus (RSV) [60,66,99], either by inhibiting the virus adsorption or the entry into the host cells. Some of the S-PSs are effective only if applied simultaneously with the virus or immediately after infection [60]. Another mechanism of action of fucoidans and other S-PSs is through the inhibition of the syncytium formation induced by viruses [2,100]. Some sulfated-xylomannans were reported to present antiviral sulfate-dependent activity, as it was the case of PSs from S. polydactyla and S. latifolium, which inhibited the multiplication of HSV-1 in Vero-cells [1,101]. Additionally, the molecular weight (MW) seems to play an important role in the antiviral properties of the S-PSs, the effect increasing with the molecular weight [60]. However, other structural features can be co-responsible for the reinforcement of the antiviral effectiveness, like sulfation patterns, composition and distribution of sugar residues along the backbone, and the complexity of the polymers [60,64,83,90]. Further, the fucoidans from L. japonica already proved their effectiveness in fighting both RNA and DNA viruses [54], such as poliovirus III, adenovirus III, ECHO6 virus, coxsackie B3 and A16 viruses. Moreover, these S-PSs can protect host cells by inhibiting the cytopathic activity of those viruses [102].
In addition to their virucidal activity against HIV and other viruses associated to sexually transmitted diseases (STD) [103], including HPV, some carrageenans might find application as vaginal lubricant gels and coatings of condoms, with microbicidal activity, for they do not present any significant anticoagulant properties or cytotoxicity [104,105]. Furthermore, some fucoidans, apart from inhibiting attachment of virus particles to host cells, were able to inhibit the attachment of human spermatozoids to the zona pellucida of oocytes [106]; this property could be used for the development of a contraceptive gel with microbicidal characteristics [40]. The PSs from some algae, and which may be released into the culture medium, showed antiviral activity against different kinds of viruses, such as the HIV-1, HSV-1 and HSV-2, VACV and Flu-A, as described by Raposo et al. [72] S-PSs, in particular, proved to increase the antiviral capacity [107]. In fact, the antiviral activity of the PSs may depend on the culture medium, algal strain and cell line used for testing, but also on the methodology, and the degree of sulfation, as is the case of PSs from P. cruentum [108,72]. Despite the slight toxicity that some PSs may present, they could be safely applied in in vivo experiments, decreasing the replication of the virus VACV, for instance [109]. The mechanisms involved in the antiviral activity of S-PSs may be understood analyzing what happens when cells are infected by a virus. Just before infection, viruses have to interact with some glycosaminoglycan receptors (GAG), such as heparin sulphate (HS) [110]. The GAG to which a protein can be covalently bound are part of the target cell surface and can also be found in the intracellular matrix of various connective and muscle tissues. S-PSs may impair the attachment of the virus particles by competing for those GAG-receptors, as they are chemically similar to HS [96,111], most of them having a covalently linked core protein [112,113].
Besides, as it happens with GAG, S-PSs are negatively charged and highly sulfated polymers [96,114,115], whose monosaccharide distribution pattern might influence the specificity of the bound protein, determining several biological functions [110]. For viruses to attach to the host cell surface, the linkage between the basic groups of the glycoproteins of the virus and the anionic components of the PSs (sulfate) at the cell surface must be established [83]. In fact, whichever the algal PSs are, either from algae, by mimicking this GAG, they may induce the formation of a virus-algal PSs complex, thus, impairing the cell infection by blocking the interaction virus-host cell receptor. Hidari et al. [114], for instance, showed that dengue virus (DENV) establishes an exclusive complex with fucoidan, and viral infection is, therefore, inhibited. They suggested that arginine-323 had a high influence on the interaction between the DENV-2 virus and the fucoidan, in an in-vitro experiment with BHK-21 cells. These researchers also found that glucuronic acid seems to be crucial since no antiviral activity was observed when this compound was reduced to glucose. Sulfated polysaccharides from algae, such as alginates, fucoidans and laminaran appear to have antibacterial activity against E. coli and species from Staphylococcus. A fucoidan from L. japonica and sodium alginate were found to inhibit E. coli [116], for example, by adhering to bacteria and killing those microorganisms [103], thus showing bactericidal properties. This type of PS is also a good antibacterial agent against Helicobacter pylori, eradicating their colonies, restoring the stomach mucosa, in clinical trial studies, and regenerating biocenosis in the intestines [117]. Laminaran from Fucus, Laminaria, A. nodosum and U. pinnatifida demonstrated to have an effect on pathogenic bacteria [118] as well, with the advantage of being unable to promote blood coagulation [119]. In contrast, the carrageenans from some seaweeds [120] and the S-PSs from the red algae Porphyridium cruentum, despite the higher concentration used [72], showed a significant inhibitory activity against S. enteritidis. In fact, some PSs from microalgae, such as A. platensis, may present antibacterial properties against some specific bacteria, the activity depending on the solvent used to extract the polymer [38]. By stimulating the production and/or expression of ILs, dectin-1 and toll-like receptors-2 on macrophages and dendritic cells, respectively, (1---3)-β-glucans from C. vulgaris, and laminarans, also induced antifungal and antibacterial responses in rats [121], and some resistance to mammal organisms towards infections by E. coli [122]. Therefore, these types of PSs promise to be good antimicrobial agents.
Anti-Inflammatory and immunomodulatory activities
PSs from algae have long demonstrated to have biological and pharmaceutical properties, such as anti-inflammatory and immunomodulation [81,123,72]. Nevertheless, the antiinflammatory properties may be shown in several ways, depending on the PSs, its source and type/site of inflammation. There is growing evidence that S-PSs are able to interfere with the migration of leukocytes to the sites of inflammation. For example, the heterofucan from D. menstrualis decreases inflammation by directly binding to the cell surface of leukocytes, especially polymorphonuclear cells (PMNs). It completely inhibits the migration of the leukocytes into the peritoneal cavity of mice where the injured tissue was after being submitted to simulated pain and inflammation, without the production of pro-inflammatory cytokines [49]. Every so often, the recruitment of these PMNs shows to be dependent on P- and/or L-selectins, as it was demonstrated for fucoidans of some brown algae [33,124]. Some other studies refer the association of the anti-inflammatory activity with the immunomodulatory ability. This seems to be the case in the work by Kang et al. [125] who simulated an inflammation process in RAW 264.7 cells induced by lipopolysaccharides (LPS). They found that the fucoidan from E. cava inhibited, in a dose-dependent manner, the enzyme nitric oxide synthase induced by LPS (iNOS) and the gene expression for the enzyme cyclooxygenase-2 (COX-2) and, as a consequence, the production of nitric oxide (NO) and prostaglandin E2 (PGL2). Li et al. [65] confirmed the anti-inflammation mechanism in vivo via the immunomodulatory system in-vivo, since the fucoidan from L. japonica reduced the inflammation of rats’ myocardium damaged cells, by inactivating the cytokines HMG B1 and NF-κB, two groups of proteins secreted by the immune cells during inflammatory diseases. These protective and regenerative effects of fucoidans, via the immunomodulatory system, were also verified in the destruction/proteolysis of connective tissue by Senni et al. [126]. These researchers referred to the fact that severe inflammation and the subsequent excessive release of cytokines and matrix proteinases could result in rheumatoid arthritis or chronic wounds and leg ulcers, which could be treated with fucoidans [126].
In addition to the SPs from Ulva rigida, green algae [127], the S-PSs p-KG03 from the marine dinoflagellate G. impudicum, also activates the production of nitric oxide and immunostimulates the production of cytokines in macrophages [128]. The enhancement of the immunomodulatory system by some S-PSs from marine algae is also a way for S-PSs to suppress tumour cells growth and their proliferation, and to be natural neoplastic-cell killers. Studies with arabinogalactan and other fucoidans revealed them to be immunostimulators by activating macrophages and lymphocytes, which suggests their effectiveness in the immuno-prevention of cancer [43,129]. The PSs from U. pinnatifida was also suggested to treat/relieve the symptoms of pulmonary allergic inflammation as it suppresses the activity of Th2 immune responses [130]. On the other hand, fucoidan activated macrophages and splenocytes to produce cytokines and chemokines [131]. PSs from algae, such as Porphyridium, Phaeodactylum, and C. stigmatophora, showed pharmacological properties, such as anti-inflammatory activity and as immunomodulatory agents, as reported by Raposo et al. [72]. Some of these S-PSs, for example, the ones from C. stigmatophora and P. tricornutum, have revealed anti-inflammatory efficacy in vivo and in vitro [132]. The mechanisms underlying the anti-inflammatory and immunomodulatory activities may be understood by making some considerations at the molecular level. On one side, the protein moiety that is covalently bound to most PSs seems to play a critical role in the activation of NF-κB and MAPK pathways involved in the macrophage stimulation [133,113]. This was evidenced in an in vitro experiment performed by Tabarsa et al. [113]. They showed that the PSs from C. fragile was not able to stimulate RAW264.7 cells to produce NO and the protein alone was also unable to induce NO release, but the complex S-PS-protein did inhibit the inflammatory process. On the other side, several other researchers found that proteins were not essential or responsible for the immunostimulatory responses of the cells [134,127]. Additionally, Tabarsa et al. [135] confirmed that the sulfate content and the MW were not crucial for the stimulation of murine macrophage cells. In fact, both desulfated and LMW-PS derivatives of C. fragile produced immunomodulatory responses similar to the ones of the original PSs. In contrast, the S-PSs from U. rigida induced a strong sulfatedependent release of NO [127], thus, the sulfate content showing to be essential for the stimulation of macrophages.
These researchers mentioned the possibility of the sulfate interfering in the interaction PS-cell surface receptors. The interaction of algal S-PSs with the complement system suggests that they might influence the innate immunity to reduce the proinflammatory state [91,81]. In addition, algal polysaccharides have been shown to regulate the innate immune response directly by binding to pattern recognition receptors (PRRs) [136]. For example, λ-carrageenan stimulated mouse T cell cultures in a tolllike receptor-4 (TLR4) [138]. Different effects were observed in other types of S-PSs: Zhou et al. [137] proved that carrageenans from Chondrus with LMW s better stimulated the immune system. The same trend was verified for the S-PSs from the red algae Porphyridium [139], a 6.53 kDa LMW-fragment at 100 μg/mL presenting the strongest immunostimulating activity. It is worth remarking that carrageenans from red seaweeds are recognized for triggering potent inflammatory and carcinogenic effects either in rats or mice cells [111]. However, while some carrageenans stimulate the activity of macrophages, others inhibit macrophage activities [2]. While PSs from various algae do not show anticoagulant and/or antithrombotic activities, attention should be paid to the anticoagulant properties of some PSs, since their use could cause severe bleeding complications.
Anti-proliferative, tumour suppressor, apoptotic and cytotoxicity activities
The current understanding of the anti-cancer and immunomodulating effects of PSs are as follows: (i) prevention of onset of cancer by oral consumption of mushrooms or their preparations; (ii) direct inhibition of growth of various types of cancer cells; (iii) immunostimulating activity against cancers in combination with chemotherapy; (iv) preventive effect on spreading or migration of cancer cells in the body [15]. On the whole, the indirect anti-cancer as well as immunostimulatory effects of lentinan is attributed to the activation of many immune cells. Lentinan can activate them to modulate the release cell signal messengers such as cytokines. The increases in cytokine production in immune cells have been studied in mice and in humans [140,141].
Because of the growing number of individuals suffering from different types of cancer and the secondary effects of synthetic chemicals and other types of treatment used against tumour damages, research was driven towards demand for natural therapeutics with bioactive compounds. In this context, S-PSs from both macro algae and micro algae already proved to have antitumor biological activities. A sulfated-fucoidan from C. okamuranus exhibited anti-proliferative activity in U937 cells by inducing cell apoptosis following a pathway dependent of Caspases-3 and -7 [142]. In another study, conducted by Heneji et al. [143], a similar fucoidan induced apoptosis in two different leukaemia cell lines. These results indicate that fucoidans might be good candidates for alternative therapeutics in treating adult T-cell leukaemia [43]. Sulfated-fucoidans from E. cava also seem to be promising to treat other types of human leukaemia cell-lines [144]. There was some evidence that the fucoidan from L. guryanovae inactivated the epidermal growth factor (tyrosine kinase) receptor (EGFR), which is greatly involved in cell transformation, differentiation and proliferation [145,146]. Therefore, this kind of S-PSs could be used as anti-tumor and anti-metastatic therapeutical/preventing agent, which might act either on tumour cells or by stimulating the immune response [147]. Further, the S-PSs from E. bicyclis and several other algae have demonstrated their potent bioactivity against different kinds of tumours, including lung and skin, both invitro and in-vivo [55,148-150] causing apoptosis in various tumour cell-lines [151,55,152].

The mechanisms involved in this antitumor activity might be associated again with the production of pro-inflammatory interleukins IL-2 and IL-12 and cytokine interferon-gamma (INF-γ) by the immune-stimulated macrophages, together with the increase of the activity of the natural killer cells (NK cells) and the induction of apoptosis [55,21]. NK cells can also upregulate the secretion of IFN-γ, which can activate either the T-cells for the production of IL-2 or the macrophages, which, after being activated, keep on producing IL-12 and activating NK cells [153,154]. The enhancement of the cytotoxicity of these NK cells (lymphocytes and macrophages) can be stimulated by other S-PSs such as fucoidans and carrageenans from other algae [129,137]. PSs can also activate some signaling receptors in the membranes of macrophages, such as Toll-like receptor-4 (TLR-4), cluster of differentiation 14 (CD14), competent receptor-3 (CR-3) and scavenging receptor (SR) [155]; these are also activated by other intracellular pathways, involving several other protein-kinases, that enhance the production of NO, which, in turn, plays an important role in causing tumour apoptosis [155]. These immunomodulation properties of S-fucoidans could be used for the protection of the damaged gastric mucosa as it was already demonstrated by using rat-models [156]. More information on the pathways and mechanisms responsible for the immune-inflammatory activities, including the involvement of the complementary system, may be found [60]. The anti-adhesive properties of some S-PSs, especially fucoidans might also explain their anti-metastatic activity, both in-vitro and in-vivo, in various animal models [157,33], as they can inhibit the adhesion of tumour cells to platelets, thus decreasing the possibilities of proliferation of neoplastic cells. The mechanisms by which fucoidans and other S-PSs exert their anti-adhesive ability were well documented by Li et al. [54]. Some researchers also highlighted the mitogenic properties and the cytotoxicity and tumoricidal activity of some arabinogalactans and fucoidans as well [129,158], either in different cell-lines or various animal models.
The anti-adhesive properties of algal S-PSs may also be relevant as these polymers can block the adhesion of tumour cells to the basal membrane, thus demonstrating to impair implantation of tumour cells and metastatic activity by binding to the extracellular matrix [159]. For example, the S-PSs from Cladosiphon were shown to prevent gastric cancer in-vivo, since it inhibited the adhesion of H. pylori to the stomach mucosa of gerbils [160]. Metastasis appearance could also be reduced in vivo by sulfated-laminaran, a (1---3): (1---6)-β-D-glucan, because this compound inhibited the activity of heparanase, an endo-β-D-glucuronidase involved in the degradation of the main PSs component in the basal membrane and the extracellular matrix. The expression of this enzyme is known to be associated with tumour metastasis [161]. These anti-tumor properties may also be found in some PSs from platensis, which are inhibitors of cell proliferation [78]. Other S-PSs, such as S-PSs p-KG03 from G. impudicum, has also anti-proliferative activity in cancer cell lines and inhibitory activity against tumour growth [128,162,163]. Other PSs from algae, such as C. vulgaris, and S-PS or LMW-derivatives of S-PS from P. cruentum, for example, are described as having similar properties [38]. In some research work, the immunomodulatory activity was associated to the ability of inhibiting carcinogenesis. Jiao et al. [47] found that a sulfated-rhamnan and some derivatives from the green seaweed E. intestinalis suppressed tumour cell growth in-vivo, but they did not show any toxicity against tumour cells in-vitro.

The oral administration of the S-PSs to mice enhanced the spleen and thymus indexes, and also induced the production of TNF-α and NO in macrophages, increased lymphocyte proliferation, and enhanced TNF-α release into serum. The degree of sulfation may play some role in the carcinogenesis process, although the action of the S-PSs may also depend on the type of tumour. In fact, an over S-PSs demonstrated the capacity of inhibiting the growth of L-1210 leukaemia tumour in mice, but, on the other hand, it was unable to inhibit the growth of Sarcoma-180 tumour in mice [149,123]. In addition to the sulfation level, MW may also influence the anticancer activity. For instance, LMW-PS derivatives showed to enhance anti-tumor activity [164]. On the other hand, the increment in the anticancer activity greatly depends on the conditions of the PSs depolymerisation [165]. Kaeffer et al. [82] suggested that the in-vitro anti-tumor activity of LMW-PS sulfated or not, against cancerous colonic epithelial cells might be associated with the inhibition of tumour cells proliferation and/or differentiation.
Lentinan can also increase engulfing ability of certain immune cells to search and destroy migratory cancer cells in the human body [166,167]. Treatment with lentinan can also enhance production of chemical messenger such as nitric oxide to stimulate the immune system [140,168]. In addition, the immune-activating ability of lentinan may be linked with its modulation of hormonal factors, which are known to play a role in cancer growth. The anticancer activity of lentinan is strongly reduced by administration of hormones such as thyroxin orhydrocortisone [169]. Moreover, lentinan can also enhance the immune response to the presence of cancer cells in the body by triggering cancer-specific reactions to fight against them. The mechanism of anti-cancer activity of lentinan is summarized in Figure (1) [170]. Overall, lentinan can suppress the growth and even kill cancer cells directly via multiple pathways involving activation of human immune system by different mechanisms such as stimulation of various immune cells and production of cell signal messengers [171].
Anticoagulant and antithrombotic activities
There are several studies on the anticoagulant properties of PSs isolated from algae, presented in a recent review [72] by different researchers: Cumashi et al. [33], Athukorala et al. [172], Costa et al., [68], Wijesekara et al., [42] and Wang et al. [41]. The main sources of the S-PSs from green algae with anticoagulant properties are Codium and Monostroma [143,144]. Some of the PSs, such as S-rhamnans, showed their action by extending the clotting time via the intrinsic and extrinsic pathways [174]. In fact, Codium spp present strong anticoagulant effects [175,176], but other species from Chlorophyta also contain S-PSs (native, LMW or otherwise modified) with anticoagulant properties. The mechanism of action of the referred PSs is mostly attributed to either a direct inhibition of thrombin or by enhancing the power of antithrombin III [177,178]. Some other PSs from green seaweeds also showed potent anticoagulant properties but their mechanisms of action are associated not only to a direct increase in the clotting time (APTT assays) by inhibiting the contact activation pathway, but also by inhibiting the heparin cofactor II-mediated action of thrombin [179,180] thus showing a potent antithrombotic bioactivity. In addition to their anticoagulant properties demonstrated in-vitro by APTT and TT tests, several S-PSs from algae of different groups present antithrombotic qualities in-vivo [181,50] by increasing the time of clot formation. In fact, Wang et al. [41] published an exhaustive work on this issue by including a summary table with 24 references about both the anticoagulant, and anti-and prothrombotic activities of several S-PS from various green algae.
In two other studies, Costa et al. [68] & Wijesekara et al. [42] also included the S-PS from brown and red algae that present effects on the blood clotting time. Wijesekara et al. [42] referred to the fact that there are few reports on the interference of PSs from algae on the PT (prothrombin) pathway, meaning that most of the marine S-PSs may not affect the extrinsic pathway of coagulation [42]. As a matter of fact, Costa et al. [68] did not detect any inhibition in the extrinsic coagulation pathway (PT test), for the concentrations used; only C. cupressoides increased the clotting time. Also, they found no anticoagulant properties (APTT and PT assays) in the S-PS from S. filipendula (brown algae) and G. caudate (red algae). Additional, in our laboratory we found no anticoagulant properties in the S-PSs from different strains of the red algae P. cruentum, despite the high content in sulfate and molecular weight. As Costa et al. [68] observed this could be due to the absence of sulfate groups in the monosaccharides at the non-reducing ends of the branches, which impaired the interaction between target proteases and coagulation factors. Nishino et al. [87] & Dobashi et al. [182] defended that there might be no effect above an upper limit for the content in sulfate, since the difference in the anticoagulant and antithrombotic activities decreased with the increase of the sulfate content. It seems that some of the chemical and structural features of the S-PSs may have some influence on their anticoagulant and/ or antithrombotic activities. The degree and distribution pattern of sulfate, the nature and distribution of monosaccharides, their glycosidic bonds and also the molecular weight showed to play some role on the coagulation and platelet aggregation processes induced by sulfated-galactans and sulfated-fucoidans [68,183,184]. In fact, at least for some fucoidans, the anticoagulant properties are related to the content in C-2 and C-2, 3 di-sulfates, this last feature being usually common in these PSs [185,186,131]. Several other studies documented the anticoagulant activity and inhibition of platelet aggregation [54,111,43], supplying more information on the mechanisms of different S-PSs for these biological activities. HMW-PS usually presents stronger anticoagulant activity [187] and if PSs has a more linear backbone, a longer polymer is required to accomplish the same anticoagulant effects [88]. On the other hand, both the native PSs and LMW-derivatives of M. latissimum presented strong anticoagulant activities [188]. Nishino and colleagues also observed that HMW fucans (27 and 58 kDa) showed greater anticoagulant activity than the ones with LMW (~10 kDa) [189].
They found that a higher content of fucose and sulfate groups coincided with higher anticoagulant activities of fractions from E. kurome [189]. However, despite its high sulfation level, the galactofucan from U. pinnatifida lacks significant anticoagulation activity [159]. In addition, a sulfated-galactofucan from schröederi did not present any anticoagulant properties in-vitro but demonstrated a strong antithrombotic activity when administered to an animal model during an experimental induced venous thrombosis, this effect disappearing with the desulfation of the polymer [190]. As for other PSs, the anticoagulant properties of the PSs from marine algae may not only depend on the percentage of sulfate residues, but rather on the distribution/position of sulfate groups and, probably, on the configuration of the polymer chains [72]. Spirulan from A. platensis is one of the PSs from microalgae that strongly interfere with the blood coagulation-fibrinolytic system and exhibits antithrombogenic properties [97], then, promising to be an anti-thrombotic agent in clots’ breakdown, although care should be taken regarding hemorrhagic strokes [38].
Figure (4) summarizes the preponderant target sites for the S-PSs from marine organisms on the coagulation system. Blue and red arrows indicate anticoagulant and pro-coagulant effects, respectively. (+) indicates activation and (−) indicates inhibitory effects. Anticoagulant effect: SG and FCS inhibit the intrinsic tenase and prothrombinase complexes [202,203]. It is still unclear if sulfated fucans (SF) have similar effects. These PS also potentiate the inhibitory effect of antithrombin (AT) and/or heparin cofactor II (HCII) on thrombin [199,204]. Their effects on factor Xa are very modest. The serpin-independent action preponderates on the plasma system. Pro-coagulant effect: SG and FCS activate factor XII [205,206]. This effect may result in severe hypotension (due to bradykinin release) and pro-coagulant (pro-thrombotic) action. It is unclear if SF activates factor XII. SF inhibits Tissue Factor Protease Inhibitor (TFPI), a specific inhibitor of the extrinsic tenase complex. So, SF has a pro-coagulant effect [207,208]. Of course, further studies are necessary to investigate whether this distinct mechanism of action may confer favorable effects to the PSs for the prevention and treatment of thromboembolic events. In particular, it is necessary to clarify which one of the two mechanisms (serpindependent or serpin-independent) is more favorable for an antithrombotic therapy.
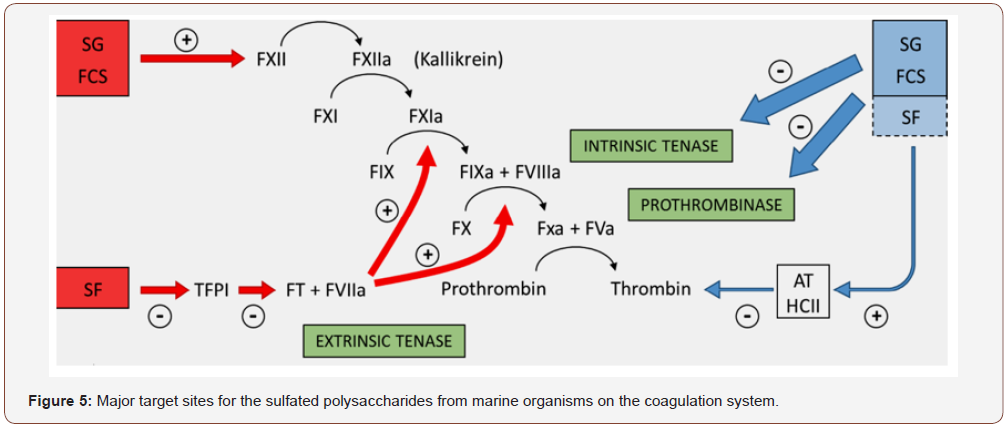
Antilipidaemic, hypoglycemic and hypotensive activities
S-PSs from algae are potent inhibitors of human pancreatic cholesterol esterase, an enzyme that promotes its absorption at the intestinal level; this inhibitory effect is enhanced by higher molecular weights and degree of sulfation [2]. A sulfated-ulvan from U. pertusa in an in-vivo study using mice models regulated the ratio HDL/LDL-cholesterol and reduced the levels of triglycerides (TG) in serum [209]. On the other hand, in another experiment with rats and mice, using native ulvans from the same species, the animals experienced a hypocholesterolaemic effect but no reduction in the TG profile [210]. An opposite reaction was observed when the PSs was acetylated and over sulfated, as TG levels were normalized. It seems that the ability to sequester bile extracts may be involved [210]. The contents in sulfate and acetylate groups play important roles during the dislipidaemia process [211,212]. Ulvans from Ulva spp also showed antiperoxidative properties, preventing liver tissues from hyperlipidaemia, including that induced by toxic chemicals and protecting the injured tissue from the oxidative stress [213], and improving antioxidant performance of the animal models. In fact, these S-PSs regulated superoxide dismutase (SOD) and catalase, increased vitamins E and C, and reduced glutathione, and had some role in reducing the levels of aspartate and alanine transaminases in the rats’ liver [214,209]. Additionally, the S-PSs from M. nitidum also demonstrated hepatoprotective activity by increasing the expression of liver detoxifying enzymes, and, therefore, showed to be good agents for chemoprevention medicine [215].
The activity of these PSs may be related to their uronic acid and sulfate content, which are able to sequester and bind to bile acids [216], reducing their levels. Other S-PSs from green algae also revealed hypolipidaemic properties, such as that from E. prolifera. These PSs regulated the lipidic profile both in plasma and liver, increasing HDL-cholesterol, in rats [217]. Fucoidans from L. japonica, the native or LMW-derivate, have hypolipidaemic effects, decreasing total and LDL-cholesterol in the serum and TG in rats [218], and they prevented hyperchole-sterolaemia in mice [133]. Another mechanism to reduce blood cholesterol in humans by S-PSs is associated to their high capacity to inhibit pancreatic cholesterol esterase, which is responsible for the absorption of cholesterol and fatty acids at the intestine [2]. It seems that the presence of sulfate at the C-3 position of the sugar residues greatly enhances that inhibition [2]. Porphyran from P. yezoensis has antihyperlipidaemic properties [219,220] by reducing the release of apolipoprotein-B100 (apoB100) and decreasing the synthesis of lipids in human liver cultured cells [221]. By reducing the secretion of apoB100, porphyran has the potential to be used as a therapeutic agent to treat CVD. Additionally, some types of carrageenans have already proved to decrease blood cholesterol in humans [222] and in rats fed on a diet enriched with a mixture of κ/λ- carrageenans from G. radula [223]. Most of the PSs from marine algae are naturally highly sulfated, with high molecular weights, making them not easily absorbable and thus enabling them to be used as anticholesterolaemic agents. Few studies were carried out in this area, namely focusing on Porphyridium, P. cruentum, R. reticulata [224-227], but these suggest a strong potential of S-PSs from unicellular algae to be used as hypolipidaemic and hypoglycaemic agents, and as promising agents for reducing coronary heart disease, due to their hypocholesterolaemic effects [72]. As far as we know, scarce research was performed on the mechanisms underlying the antihyper-lipidaemic activity. However, the sequestration and disruption of the enterophatic circulation of the bile acids may be involved [209,228,229]. Ulvans and their LMW-derivatives, and also the S-PSs from Porphyridium showed to increase the excretion of bile [230,210]. Another explanation for the antihyperlipidaemic activity of S-PSs may be associated to the fact that they can effectively increase the anionic charges on the cell surface, which improve the removal of cholesterol excess from the blood, thus, resulting in a decrease of serum cholesterol [54]. In addition, most PSs have ion exchange capacity, such as those from Porphyridium and Rhodella [231], and they can function as dietary fibres. This could also explain the ability to lower down cholesterol [232]. PSs may act as dietary fibres, immunostimulating the goblet cells in the intestine to increase the release and effects of mucin [233]. Moreover, the administration of PSs may increase the viscosity of the intestinal contents, interfering with the formation of micelles and nutrient absorption, thus, lowering lipid absorption, and reducing gastrointestinal transit time (GTT) [230,57]. Other PSs have the ability to inhibit the enzyme α-glucosidase, thus improving the postprandial hyperglycaemia [234], and another can also reduce the blood pressure by inhibiting the release of plasma angiotensin II [235].
Wound healing and wound dressing
Due to their inherent biocompatibility, low toxicity, and pharmaceutical biomedical activity, various PSs, suchas chitin, chitosan, cellulose, hyaluronan, and alginate, have been widely used to prepare wound healing materials [236,142,237]. Hyaluronan, a major extracellular component with unique hygroscopic, rheological, and viscoelastic properties, has been extensively developed for tissue repair purposes due to its physicochemical properties and specific interactions with cells and extracellular matrix. It is generally accepted that hyaluronan plays multifaceted roles in the mediation of the tissue repair process and is involved in all the stages of wound healing, i.e. inflammation, granulation tissue formation, reepithelialization, and remodeling. Derivatives of hyaluronan, such as cross-linked, esterified or other chemically modified products have also been developed for tissue repair or wound healing purposes [238,239]. Remarkably, wound healing promoting activity of the materials is also important in the designing of materials for tissue engineering. All-natural composite wound dressing films prepared by dispersion and encapsulation of essential oils in sodium alginate matrices have been reported to show remarkable antimicrobial and antifungal properties and may find applications disposable wound dressings [240].
Chitosan/silk fibroin blending membranes crosslinked with dialdehyde alginate have been developed for wound dressing and the membranes were found to promote the cell attachment and proliferation, which suggests a promising candidate for wound healing applications [241]. Blending aqueous dispersions of sodium alginate and povidone iodine (PVPI) complex was prepared as free standing NaAlg films oras Ca2+ cross-linked alginate beads. These products were demonstrated to show antibacterial and antifungal activity and controlled release of PVP Iinto open wounds when the composite films and beads were brought into direct contact with water or with moist media [240]. This proved that they could be suitable for therapeutic applications such as wound dressings. In situ injectable nano-composite hydrogels composed of curcumin, N, O-carboxymethyl chitosan, and oxidized alginate as a novel wound dressing was successfully developed for dermal wound repair application [139]. In-vitro release, in-vivo wound healing, and histological studies all suggested that the developed nanocurcumin/ N, O-carboxymethyl chitosan/ oxidized alginate hydrogel as apromising wound dressing might have a potential application in the wound healing. Silver nanoparticles containing polyvinyl pyrrolidone and alginate hydrogels were synthesized using gamma radiation and showed the ability of preventing fluid accumulation in exudating wound [242]. The incorporation of nanosilver particles provided as trong antimicrobial effect and therefore made such polyvinyl pyrrolidone/alginate hydrogels suitable for use as wound dressing. Except the alginate and its various derivatives, other natural PSs such as cellulose, chitin, chitosan, and hyaluronic acid have also been explored for wound dressingor wound healing applications [243-245].
Antioxidant activity
Oxidation is an essential process for all living organisms for the production of energy necessary for biological processes [246]. In addition, oxygen-centered free radicals are involved in development of a variety of diseases, including cellular aging, mutagenesis, carcinogenesis, coronary heart disease, diabetes and neurodegeneration [247]. Though almost all organisms possess antioxidant defense and repair systems to protect against oxidative damage, these systems are often insufficient to prevent the damage entirely [98]. Recently, much attention was paid to screening natural biomaterials in the case of several clinical situations since use of synthetic antioxidants is restricted due to their carcinogenicity [135]. Among various natural antioxidants, PSs in general has strong antioxidant activities and can be explored as novel potential antioxidants [248,249]. Recently, PSs isolated from fungal, bacterial and plant sources were found to exhibit antioxidant activity and were proposed as useful therapeutic agents [250,251].
essential process for all living organisms for the production of energy necessary for biological processes [246]. In addition, oxygen-centered free radicals are involved in development of a variety of diseases, including cellular aging, mutagenesis, carcinogenesis, coronary heart disease, diabetes and neurodegeneration [247]. Though almost all organisms possess antioxidant defense and repair systems to protect against oxidative damage, these systems are often insufficient to prevent the damage entirely [98]. Recently, much attention was paid to screening natural biomaterials in the case of several clinical situations since use of synthetic antioxidants is restricted due to their carcinogenicity [135]. Among various natural antioxidants, PSs in general has strong antioxidant activities and can be explored as novel potential antioxidants [248,249]. Recently, PSs isolated from fungal, bacterial and plant sources were found to exhibit antioxidant activity and were proposed as useful therapeutic agents [250,251]. The main mechanism by which S-PSs from green algae exert their primary antioxidant action is by scavenging free-radicals (DPPH-radicals) or by inhibiting their appearance [251]. They also demonstrated to have total antioxidant capacity, and a strong ability as reducing agents and as ferrous chelators [251]. However, some S-PSs, such as sulfated-heterogalactan from C. cupressoides do not show a good scavenging power, but they are rather powerful against reactive oxygen species (ROS) [252]. It is interesting to note that fucoidans from brown algae seem to exert a reducing power bigger than the S-PSs from other groups [68]; the PSs from S. filipendula has an effect even stronger than vitamin C. Moreover, the fucoidan from L. japonica has a great potential to be used in medicine in order to prevent free-radical mediated diseases, as it successfully prevented peroxidation of lipids in plasma, liver and spleen in-vivo, despite showing no effects in-vitro
The S-PSs from S. fulvellum has shown a NO scavenging activity higher than some commercial antioxidants [253]. In addition, the S-PSs from the red algae P. haitanensishas demonstrated to decrease antioxidant damages in aging mice [254]. It seems that LMWS- PS may present higher antioxidant activity than the native polymers, as it was verified with the PSs from U. pertusa and E. prolifera [255,256]. It is probably related with the ability of PSs to be incorporated in the cells and to donate protons [42]. As noted by Raposo et al. [72], S-PSs produced and secreted out by marine algae have shown the capacity to prevent the accumulation and the activity of free radicals and reactive chemical species. Hence, S-PSs might act as protecting systems against these oxidative and radical stress agents. The S-PSs from Porphyridium and Rhodella reticulata exhibited antioxidant activity [257,258], although some research revealed no scavenging activity and no ability to inhibit the oxidative damage in cells and tissues for the crude S-PSs with high molecular weight from P. cruentum, while the PS-derived products after microwave treatment showed antioxidant activity [259].
In all cases, the antioxidant activity was dose dependent. PSs from A. platensis also exhibit a very high antioxidant capacity [260]. Due to their strong antioxidant properties, most of the S-PSs from marine algae are promising since they may protect human health from injuries induced by ROS, which can result in cancer, diabetes, some inflammatory and neurodegenerative diseases, and some other aging-related disorders, such as Alzheimer and CVD. The influence of sulfate content on the antioxidant activity depends rather on the origin of the PSs. For example, the PS from U. fasciata and other algae with lower sulfate content demonstrated a strong antioxidative power [261,262,258,259], while the antioxidant activity observed in PSs from E. linza and other seaweeds showed to be sulfate-dependent [263,264]. Furthermore, high sulfated PSs were shown to have an enhanced scavenging power [251,265], this property being also dependent on the sulfate distribution pattern [68]. It seems, in addition, that the protein moiety of PSs may play some role on the antioxidative power. For example, Tannin-Spitz et al. [258] reported a stronger antioxidant activity for the crude PSs of Porphyridium than for the denatured PSs. Zhao et al. [266] found that the antioxidant activity of S-PSs was apparently related, not only to MW and sulfated ester content, but also to glucuronic acid and fructose content. This antioxidant activity seems to be attributable to metal chelating, free radical and hydroxyl radical scavenging activities of the S-PSs.
Toxicity of polysaccharides
The toxicity of polysaccharide is very crucial to the development of any product for the medical treatments. An animal experiment was conducted to evaluate the toxicity of polysaccharide and the results found that no toxicity was exhibited to the liver, kidney, heart, thymus or spleen of the mice which were fed with the polysaccharide conjugate and none of the mice died throughout the period of the experiment. There was no significant difference between the thymus index, spleen index and liver index of the mice from the test and control groups. It might be a candidate of dietary supplements besides the bioactivities as a polysaccharide [267- 293].
Acknowledgement
None.
Conflict of Interest
No conflict of interest.
References
- Cui SW (2005) Food Carbohydrates: Chemistry, Physical Properties, and Applications, CRC Press.
- Laurienzo P (2010) Marine polysaccharides in pharmaceutical applications: An overview. Marine Drugs 8(9): 2435-2465.
- Giavasis I (2014) Bioactive fungal polysaccharides as potential functional ingredients in food and nutraceuticals. Curr Opin Biotechnol 26: 162-173.
- Li L, Ni R, Shao Y, Mao S (2014) Carrageenan and its applications in drug delivery. Carbohydr Polym 103(1): 1-11.
- Popa EG, Reis RL, Gomes ME (2015) Seaweed polysaccharide-based hydrogels used for the regeneration of particular cartilage. Critical Rev in Biotechnol 35(3): 410-424.
- Srivastava A, Srivastava A, Srivastava A, Chandra P (2015) Marine biomaterials in therapeutics and diagnostic. Springer Handbook of Marine Biotechnology, Springer, Berlin, Germany, pp. 1247-1263.
- Venkatesan J, Lowe B, Anil S, Manivasagan P, Al Kheraif AA, et al. (2015) Seaweed polysaccharides and their potential biomedical applications, Starch-Stärke 67(5-6): 381-390.
- Giavasis I (2013) Production of microbial polysaccharides for use in food. In: Microbial Production of Food Ingredients. Enzymes and Nutraceuticals, Wood head Publishing, UK, Pp. 413-468.
- Nie S, Zhang H, Li W, Xie M (2013) Current development of polysaccharides from Ganoderma: isolation, structure and bioactivities. Bioactive Carbohydrates and Dietary Fibre 1(1): 10-20.
- Nie S, Cui SW, Xie M, Phillips AO, Phillips GO (2013) Bioactive polysaccharides from Cordyceps sinensis: isolation, structure features and bioactivities, Bioactive Carbohydrates and Dietary Fibre 1(1): 38-52.
- Wu C, Wang X, Wang H, Shen B, He X, et al. (2014) Extraction optimization, isolation, preliminary structural characterization and antioxidant activities of the cell wall polysaccharides in the petioles and pedicels of Chinese herbal medicine Qian (Euryale ferox Salisb.), Inter Jl of Biological Macromol 64: 458-467.
- Freitas F, Alves VD, Reis MAM (2011) Advances in bacterial exopolysaccharides: from production to biotechnological applications. Trends Biotechnol 29(8): 388-398.
- Anglès MN, Dufresne A (2001) Plasticized starch/tunicin whiskers nanocomposite materials Mechanical behaviour. Macromolecules 34: 2921-2931.
- Cima F, Ballarin L, Bressa G, Martinucci G, Burighel P (1996) Toxicity of organotin compounds on embryos of a marine invertebrate. Ecotoxicology Environmental Safety 35: 174-182.
- Wasser SP (2002) Medicinal mushrooms as a source of antitumor and immunomodulating polysaccharides. Applied Microb and Biotechno 60(3): 258-274.
- Sharon N, Lis H (1993) Carbohydrates in cell recognition. Sci Am 268: 82-89.
- Sutherland IW (1994) Structure function relationships in microbial exopolysaccharides. Biotechnology Adv 12(2): 393-448.
- Dwek RA (1996) Glycobiology: Toward understanding the function of sugars. Chemical Rev 96: 683-720.
- Surenjav U, Zhang L, Xu X, Zhang X, Zeng F (2006) Effects of molecular structure on antitumor activities of (1-3)- β -D-glucans from different Lentinus Edodes. Carbohydr Polym 63: 97-104.
- Tao Y, Zhang L, Yan F, Wu X (2007) Chain conformation of waterinsoluble hyperbranched polysaccharide from fungus. Biomacromolecules 8(7): 2321-2328.
- Zhang L, Li X, Xu X, Zeng F (2005) Correlation between antitumor activity, molecular weight, and conformation of Lentinan. Carbohydr Res 340(8): 1515-1521.
- Okamura K, Suzuki M, Chihara T, Fujiwara A, Fukuda T, et al. (1986) Clinical evaluation of Schizophyllan combined with irradiation in patients with cervical cancer. Cancer 58: 865-872.
- Tsuzuki A, Ohno N, Adachi Y, Yadomae T (1999) Interleukin 8 production of human leukocytes stimulated by triple or single helical conformer of an antitumor (1-3) - β -D-glucan preparation, sonifilan. Drug Development Res 48: 17-25.
- Kazuo Sakurai, Seiji Shinkai (2000) Molecular recognition of adenine, cytosine, and uracil in a single-stranded RNA by a natural polysaccharide: schizophyllan. J of Amer Chemical Soci 122: 4520-4521.
- Anada T, Karinaga R, Koumoto K, Mizu M, Nagasaki T, Katom Y, et al. (2005) Linear double-stranded DNA that mimics an infective tail of virus genome to enhance transfection. J Control Release 108: 529-539.
- Koumoto K, Karinaga R, Mizu M, Anada T, Sakurai K, et al. (2004). Protection of polynucleotides against nuclease-mediated hydrolysis by complexation with schizophyllan. Biomaterials 25(15): 3109-3116.
- Koumoto K, Karinaga R, Mizu M, Anada T, Sakurai K, et al. (2004) Removal of the side-chain glucose groups from schizophyllan improves the thermal stability of the polycytidylic acid complexes under the physiological conditions. Biopolymers 75(5): 403-411.
- Koumoto K, Mizu M, Sakurai K, Kunitake T, Shinkai S (2004) Complementary-strand-induced release of single-stranded DNA bound in the Schizophyllan complex. Chem Biodivers 1(3): 520-529.
- Mizu M, Koumoto K, Anada T, Matsumoto T, Numata M, et al. (2004) A polysaccharide carrier for immunostimulatory CpG DNAs to enhance cytokine secretion. J of Amer Chemical Soci 126(27): 8372-8373.
- Sakurai K, Iguchi R, Koumoto K, Kimura T, Mizu M, et al. (2002) Polysaccharide-polynucleotide complexes VIII. Cation-induced complex formation between polyuridylic acid and Schizophyllan. Biopolymers 65: 1-9.
- Sakurai K, Mizu M, Shinkai S (2001) Polysaccharide-polynucleotide complexes. 2. complementary polynucleotide mimic behavior of the natural polysaccharide schizophyllan in the macromolecular complex with singlestranded RNA and DNA. Biomacromolecules 2: 641-650.
- Shimada N, Coban C, Takeda Y, Mizu M, Minari J, et al. (2007) A polysaccharide carrier to effectively deliver native phosphodiester CpG DNA to antigen-presenting cells. Bioconjugate Chemistry 18: 1280-1286.
- Cumashi A, Ushakova NA, Preobrazhenskaya ME, D Incecco A, Piccoli A, et al. (2007) A comparative study of the anti-inflammatory, anticoagulant, antiangiogenic, and antiadhesive activities of nine different fucoidans from brown seaweeds. Glycobiology 5: 541-552.
- Ermakova S, Sokolova R, Kim SM, Um BH, Isakov V, et al. (2011) Fucoidans from Brown seaweeds Sargassum hornery, Ecklonia cava, Costaria costata: Structural characteristics and anticancer activity. Appl Biochem Biotechnol 164: 841-850.
- Fedorov SN, Ermakova SP, Zvyagintseva TN, Stonik VA (2013) Anticancer and cancer preventive properties of marine polysaccharides: Some results and prospects. Mar Drugs 11: 4876-4901.
- Fujitani N, Sakari S, Yamagushi Y, Takenaka H (2001) Inhibitory effects of microalgae on activation of hyaluronidase. Journal of Applied Phycology 13(6): 489-492.
- Kawamoto H, Miki Y, Kimura T, Tanaka K, Nakagawa T, et al. (2006) Effects of fucoidan from Mozuku on human stomach cell lines. Food Science and Technology Research 12(3): 218-222.
- Raposo MF, de Morais AM, de Morais RM (2014) Influence of sulphate on the composition and antibacterial and antiviral properties of the exopolysaccharide from Porphyridium cruentum. Life Sci 101: 56-63.
- Karim Senni, Jessica Pereira, Farida Gueniche, Christine Delbarre-Ladrat, Corinne Sinquin, et al. (2011) Marine polysaccharides: A source of bioactive molecules for cell therapy and tissue engineering. Mar Drugs 9: 1664-1681.
- Smitt AJ (2004) Medicinal and pharmaceutical uses of seaweed natural products: A review. J Appl Phycol 16: 245-262.
- Wang L, Wang X, Wu H, Liu R (2014) Overview on biological activities and molecular characteristics of sulfated polysaccharides from marine green algae in recent years. Mar Drugs 12(9): 4984-5020.
- Wijesekara I, Pangestuti R, Kim SK (2011) Biological activities and potential health benefits of sulfated polysaccharides derived from marine algae. Carbohydr. Polym 84(1): 14-21.
- Wijesinghe WAJP, Jeon YJ (2012) Biological activities and potential industrial applications of fucose rich sulphated polysaccharides and fucoidans from brown seaweeds: A review. Carbohydr Polym 88(1): 13-20.
- Yim JH, Kim SJ, Ahn SH, Lee HK (2007) Characterization of a novel bioflocculant, p-KG03, from a marine dinoflagellate, Gyrodinium impudicum KG03. Bioresour Technol 98(2): 361-367.
- Nomoto K, Yokokura T, Satoh H, Mutai M (1983) Anti-tumor effect by oral administration of Chlorella extract, PCM-4 by oral admission. Gan To Kagaku Zasshi 10(3): 781-785.
- Chen X, Song L, Wang H, Liu S, Yu H, et al. (2019) Partial Characterization, the Immune Modulation and Anticancer Activities of Sulfated Polysaccharides from Filamentous Microalgae Tribonema sp. Molecules 24: 322.
- Jiao L, Li X, Li T, Jiang P, Zhang L, et al. (2009) Characterization and anti-tumor activity of alkali-extracted polysaccharide from Enteromorpha intestinalis. International Immunopharmacology 9(3): 324-329.
- Matsui SM, Muizzudin N, Arad SM, Marenus K (2003) Sulfated polysaccharides from red microalgae anti-inflammatory properties in vitro and in vivo. Appl Biochem Biotechnol 104(1): 13-22.
- Albuquerque IRL, Cordeiro SL, Gomes DL, Dreyfuss, JL, Filgueira LGA, et al. (2013) Evaluation of anti-nociceptive and anti-inflammatory activities of a heterofucan from Dictyota menstrualis. Mar Drugs 11: 2722-2740.
- Rodrigues JA, Vanderlei ES, Silva LM, Araújo IW, Queiroz IN (2012) Antinociceptive and anti-inflammatory activities of a sulfated polysaccharide isolated from the green seaweed Caulerpa cupressoides. Pharmacol Rep 64: 282-292.
- Shibata H, Iimuro M, Uchiya N, Kawamori T, Nagaoka M, et al. (2003) Preventive effects of Cladosiphon fucoidan against Helicobacter pylori infection in Mongolian gerbils. Helicobacter 8: 59-65.
- Shibata H, Kimura-Takagi I, Nagaoka M, Hashimoto S, Aiyama R, et al. (2000) Properties of fucoidan from Cladosiphon okamuranus Tokida in gastric mucosal protection. Biofactors 11: 235-245.
- Raposo MF, de Morais AM (2015) Microalgae for the prevention of cardiovascular disease and stroke. Life Sci 125: 32-41.
- Li B, Lu F, Wei X, Zhao R (2008) Fucoidan: Structure and bioactivity. Molecules 13(8): 1671-1695.
- Ale MT, Mikkelsen JD, Meyer AS (2011) Important determinants for fucoidan bioactivity: A critical review of structure-function relations and extraction methods for fucose-containing sulfated polysaccharides from brown seaweeds. Mar Drugs 9: 2106-2130.
- Li B, Wei XJ, Sun JL, Xu SY (2006) Structural investigation of a fucoidan containing a fucose-free core from the brown seaweed, Hizikia fusiforme. Carbohydr Res 341(9): 1135-1146.
- Oakenfull D (2001) Physicochemical properties of dietary fiber: Overview. In Handbook of Dietary Fibers; Cho SS, Dreher MD, Eds. Marcel Dekker: New York, NY, USA, pp. 195-206.
- Usov AI (2011) Polysaccharides of the red algae. Adv Carbohydr Chem Biochem 65: 115-217.
- Pomin VH (2012) Fucanomics and Galactanomics: Marine Distribution, Medicinal Impact, Conceptions, and Challenges. Mar Drugs 10: 793-811.
- Jiao G, Yu G, Zhang J, Ewart HS (2011) Chemical structures and bioactivities of sulphated polysaccharides from marine algae. Mar Drugs 9(2): 196-223.
- Anderson NS, Dolan TCS, Rees DA (1973) Carrageenans Part VII. Polysaccharides from Eucheuma spinosum and Eucheuma cottonii. The covalent structure of L-carrageenan. J Chem Soc Perkin 19: 2173-2176.
- Funami T, Hiroe M, Noda S, Asai I, Ikeda S, Nishinari K (2007) Influence of molecular structure imaged with atomic force microscopy on the rheological behavior of carrageenan aqueous systems in the presence or absence of cations. Food Hydrocolloids 21(4): 617-629.
- Damonte E, Neyts J, Pujol CA, Snoeck R, Andrei G, et al. (1994) Antiviral activity of a sulphated polysaccharide from the red seaweed Nothogenia fastigiata. Biochem Pharmacol 47: 2187-2192.
- Ghosh T, Chattopadhyay K, Marschall M, Karmakar, P, Mandal P, et al. (2009) Focus on antivirally active sulfated polysaccharides: From structure-activity analysis to clinical evaluation. Glycobiology 19(1): 2-15.
- Li H, Mao W, Zhang X, Qi X, Chen Y, et al. (2011) Structural characterization of an anticoagulant-active sulfated polysaccharide isolated from green alga Monostroma latissimum. Carbohydr Polym 85(2): 394-400.
- Lee JB, Hayashi K, Maeda M, Hayashi T (2004) Antiherpetic activities of sulfated polysaccharides from green algae. Planta Med 70(9): 813-817.
- Bilan MI, Vinogradova EV, Shashkov AS, Usov AI (2007) Structure of a highly pyruvylated galactan sulfate from the Pacific green alga Codium yezoense (Bryopsidales, Chlorophyta). Carbohydr Res 342: 586-596.
- Costa LS, Fidelis GP, Cordeiro SL, Oliveira RM, Sabry DA, et al. (2010) Biological activities of sulfated polysaccharides from tropical seaweeds. Biomed Pharmacother 64: 21-28.
- Ohta Y, Lee JB, Hayashi K, Hayashi T (2009) Isolation of sulfated galactan from Codium fragile and its antiviral effect. Biol Pharm Bull 32: 892-898.
- Fernández PV, Ciancia M, Miravalles AB, Estevez JM (2010) Cell wall polymer mapping in the coenocytic macroalga Codium vermilara. J Phycol 46: 456-465.
- Tabarsa M, Karnjanapratum S, Cho M, Kim JK, You S (2013) Molecular characteristics and biological activities of anionic macromolecules from Codium fragile. Int J Biol Macromol 59: 1-12.
- Raposo DMF, de Morais, AMMB, de Morais RMSC (2014) Bioactivity and Applications of polysaccharides from marine microalgae. In Polysaccharides: Bioactivity and Biotechnology Merillon JM, Ramawat KG, Eds. Springer: Cham, Switzerland.
- Li P, Liu Z, Xu R (2001) Chemical characterization of the released polysaccharides from the cyanobacterium Aphanothece halophytica GR02. J Appl Phycol 13(1): 71-77.
- Liu F, Ooi VE, Chang ST (1997) Free radical scavenging activities of mushroom polysaccharide extracts. Life Sci 60(10): 763-771.
- Lee JB, Hayashi T, Hayashi K, Sankawa U, Maeda M, et al. (1998) Further purification and structural analysis of calcium spirulan from Spirulina platensis. J Nat Prod 61(9): 1101-1104.
- Pignolet O, Jubeau S, Vaca-Garcia C, Michaud P (2013) Highly valuable microalgae: Biochemical and topological aspects. J Ind Microbiol Biotechnol 40: 781-796.
- Heaney Kieras JH, Chapman D (1976) Structural studies on the extracellular polysaccharide of the red alga Porphyridium cruentum. Carbohydr Res 52(1): 169-177.
- Kaji T, Okabe M, Shimada S, Yamamoto C, Fujiwara Y, et al. (2004) Sodium spirulan as a potent inhibitor of arterial smooth muscle cell proliferation in vitro. Life Sci 74(9): 1-9.
- Lee JB, Hayashi T, Hayashi K, Sankawa U (2000) Structural analysis of calcium spirulan (Ca-SP)-derived oligosaccharides using electrospray ionization mass spectrometry. J Nat Prod 63(1): 136-138.
- Ford CW, Percival E (1965) The carbohydrates of Phaeodactylum tricornutum. Part I. Preliminary examination of the organism and characterization of low molecular weight material and of glucan. Journal of the Chemical Society 1298: 7035-7041.
- Kashif SA, Hwang YJ, Park JK (2018) Potent biomedical applications of isolated polysaccharides from marine microalgae Tetraselmis species. Bioprocess Biosyst Eng 41(11): 1611-1620.
- Kaeffer B, Benard C, Lahaye M, Blottiere HM, Cherbut C (1999) Biological properties of ulvan, a new source of green seaweed sulfated polysaccharides, on cultured normal and cancerous colonic epithelial tells. Planta Med 65(6): 527-531.
- Damonte EB, Matulewicz MC, Cerezo AS (2004) Sulfated seaweed polysaccharides as antiviral agents. Curr Med Chem 11: 2399-2419.
- Ghosh T, Pujol CA, Damonte EB, Sinha S, Ray B (2009) Sulfated xylomannans from the red seaweed Sebdenia polydactyla: Structural features, chemical modification and antiviral activity. Antivir Chem Chemother 19(6): 235-242.
- Nishino T, Kiyohara H, Yamada H, Nagumo T (1991) An anticoagulant fucoidan from the brown seaweed Ecklonia kurome. Phytochemistry 30(2): 535-539.
- Nishino T, Nagumo T (1991) The sulfate-content dependence of the anticoagulant activity of a fucan sulfate from the brown seaweed Ecklonia kurome. Carbohydr Res 214(1): 193-197.
- Nishino T, Yokoyama G, Dobahi K (1989) Isolation, purification and characterization of fucose-containing sulfated polysaccharides from the brown seaweed Ecklonia kurome and their blood-anticoagulant activities. Carbohydr Res 186(1): 119-129.
- Pomin VH, Pereira MS, Valente AP, Tollefsen DM, Pavão MS, et al. (2005) Selective cleavage and anticoagulant activity of a sulfated fucan: Stereospecific removal of a 2-sulfate ester from the polysaccharide by mild acid hydrolysis, preparation of oligosaccharides, and heparin cofactor II-dependent anticoagulant activity. Glycobiology 15: 369-381.
- Witvrouw M, de Clercq E (1997) Sulfated polysaccharides extracted from sea algae as potential antiviral drugs. Gen Pharmacol 29: 497-511.
- Harden EA, Falshaw R, Carnachan SM, Kern ER, Prichard MN (2009) Virucidal activity of polysaccharide extracts from four algal species against herpes simplex virus. Antiviral Res 83(3): 282-289.
- Clement MJ, Tissot B, Chevolot L, Adjadj E, Du Y, et al. (2010) NMR characterization and molecular modeling of fucoidan showing the importance of oligosaccharide branching in its anticomplementary activity. Glycobiology 20: 883-894.
- De Clercq E (2004) Antiviral drugs in current chemical reviews. J Clin Virol 30: 115-133.
- Mayer AMS, Lehmann VKB (2000) Marine pharmacology in 1998: Marine compounds with antibacterial, anticoagulant, antifungal, anti-inflammatory, anthelmintic, antiplatelet, antiprotozoal and antiviral activities; with actions on the cardiovascular, endocrine, immune and nervous systems: and other miscellaneous mechanisms of action. Pharmacologist 42(2): 62-69.
- Balzarini J, Van Damme L (2007) Microbicide drug candidates to prevent HIV infection. Lancet 369: 787-797.
- McReynolds KD, Garvey-Hague J (2007) Chemotherapeutic interventions targeting HIV interactions with host-associated carbohydrates. Chem Rev 107(5): 1533-1552.
- Carlucci MJ, Mateu CG, Artuso MC, Scolaro LA (2012) Polysaccharides from red algae: Genesis of a renaissance. In: The Complex World of Polysaccharides; Karunaratne DN (Edt.), InTech: Rijeka, Croatia 20: 535-554.
- Mazumder S, Ghosal PK, Pujol CA, Carlucci MJ, Damonte EB, et al. (2002) Isolation, chemical investigation and antiviral activity of polysaccharides from Gracilaria corticata (Gracilariaceae, Rhodophyta). Int J Biol Macromol 31(1-3): 87-95.
- Simic MG (1988) Mechanisms of inhibition of free-radical processes in mutagenesis and carcinogenesis. Mutat Res 202: 377-386.
- Nakashima H, Kido Y, Kobayashi N, Motoki Y, Neushul M, et al. (1987) Purification and characterization of an avian myeloblastosis and human immunodeficiency virus reverse transcriptase inhibitor, sulfated polysaccharides extracted from sea algae. Antimicrob Agents Chemother 31(10): 1524-1528.
- Mandal P, Mateu CG, Chattopadhyay K, Pujol CA, Damonte EB, et al. (2007) Structural features and antiviral activity of sulphated fucans from the brown seaweed Cystoseira indica. Antivir Chem Chemother 18(3): 153-162.
- Asker MS, Mohamed SF, Ali FM, El Sayed OH (2007) Chemical Structure and Antiviral Activity of Water-soluble Sulfated Polysaccharides from Sargassum latifolium. J Appl Sci Res 3: 1178-1185.
- Li F, Tian TC, Shi YC (1995) Study on antivirus effect of fucoidan in vitro. J N Bethune Univ Med Sci 21(3): 255-257.
- Kraan S (2012) Algal polysaccharides, novel applications and outlook. Carbohydrates-Comprehensive Studies on Glycobiology and Glycotechnology. Europe, Ppt. 489-524.
- Buck CB, Thompson CD, Roberts JN, Muller M, Lowy DR, et al. (2006) Carrageenan is a potent inhibitor of papillomavirus infection. PLoS Pathog 2(7): e69.
- Zeitlin L, Whaley KJ, Hegarty TA, Moench TR, Cone RA (1997) Tests of vaginal microbicides in the mouse genital herpes model. Contraception 56(5): 329-335.
- Oehninger S, Clark GF, Acosta AA, Hodgen GD (1991) Nature of the inhibitory effect of complex saccharide moieties on the tight binding of human spermatozoa to the human zona pellucida. Fertil Steril 55: 165-169.
- Hayashi T, Hayashi K, Maeda M, Kojima I (1996) Calcium spirulan, an inhibitor of enveloped virus replication, from a blue-green alga Spirulina platensis. J Nat Prod 59(1): 83-87.
- Huleihel M, Ishanu V, Tal J, Arad SM (2001) Antiviral effect of the red microalgal polysaccharides on Herpes simplex and Varicella zoster viruses. Journal of Applied Phycology 13(2): 127-134.
- Radonić, Aleksandar, Thulke, Stefanie, Achenbach, John, Kurth, Andreas, et al. (2010) Anionic polysaccharides from phototrophic microorganisms exhibit antiviral activities to Vaccinia virus. J Antivir Antiretrovir 2: 51-55.
- Esko JD, Selleck SB (2002) Order out of chaos: Assembly of ligand binding sites in heparin sulfate. Annu Rev Biochem 71: 435-471.
- Prajapati VD, Maheriya PM, Jani GK, Solanki HK (2014) Carrageenan: A natural seaweed polysaccharide and its applications. Carbohydr Polym 105: 97-112.
- Heaney Kieras J, Roden L, Chapman DJ (1977) The covalent linkage of protein to carbohydrate in the extracellular protein-polysaccharide from the red alga Porphyridium cruentum. Biochem J 165(1): 1-9.
- Tabarsa M, Park GM, Shin IS, Lee E, Kim JK, You S (2015) Structure-activity relationships of sulphated glycoproteins from Codium fragile on nitric oxide releasing capacity from RAW264.7 cells. Mar Biotechnol 17(3): 266-276.
- Hidari KIPJ, Takahashi N, Arihara M, Nagaoka M, Morita K, et al. (2008) Structure and anti-dengue virus activity of sulfated polysaccharide from a marine alga. Biochem. Biochem Biophys Res Commun 376(1): 91-95.
- Huleihel M, Ishanu V, Tal J, Arad SM (2002) Activity of Porphyridium sp. polysaccharide against Herpes simplex viruses in vitro and in vivo. J Biochem Biophys Methods 50(2-3): 189-200.
- Miroshnichenko VA, Yansons TY, Polushin OG (1998) Differentiated approach to the treatment of gastroduodenal pathology with the use of bioactive substances of marine hydrocele. In New Biomedical Technologies to Use of Dietary Supplements; Ivanov EM, Ed. IMKVL Siberian Branch, Ross. Akad. Med. Nank.: Vladivostok, Russia, pp. 146-150.
- Hoffman R, Paper DH, Donaldson J, Alban S, Franz G (1995) Characterization of a laminarin sulfate which inhibits basic fibroblast growth-factor binding and endothelial-cell proliferation. J Cell Sci 108(Ppt 11): 3591-3598.
- Shanmugam M, Mody KH (2000) Heparinoid-active sulfated polysaccharides from marine algae as potential blood anticoagulant agents. Curr Sci 79: 1672-1683.
- Yamashita S, Sugita-Konishi Y, Shimizu M (2001) In vitro bacteriostatic effects of dietary polysaccharides. Food Sci Technol Res 7: 262-264.
- Rice PJ, Adams EL, Ozment-Skelton T, Gonzalez AJ, Goldman MP, et al. (2005) Oral delivery and gastrointestinal absorption of soluble glucans stimulate increased resistance to infectious challenge. J Pharmacol Exper Ther 314: 1079-1086.
- Verma MS, Gu FX (2012) 1,3-β-Glucans drug delivery and pharmacology. In The Complex World of Polysaccharides, Karunaratne DN, Ed.; InTech: Rijeka, Croatia 21: 555-572.
- Park GT, Go RE, Lee HM, Lee GA, Kim CW, et al. (2017) Potential anti-proliferative and immunomodulatory effects of marine microalgal exopolysaccharide on various human cancer cells and lymphocytes in vitro. Mar Biotechnol 19: 136-146.
- Preobrazhenskaya ME, Berman AE, Mikhailov VI, Ushakova NA, Mazurov AV (1997) Fucoidan inhibits leukocyte recruitment in a model peritoneal inflammation in rat and blocks interaction of P-selectin with its carbohydrate ligand. Biochem Mol Biol Int 43: 443-451.
- Kang SM, Kim KN, Lee SH, Ahn G, Cha SH, et al. (2011) Anti-inflammatory activity of polysaccharide purified from AMG-assistant extract of Ecklonia cava in LPS-stimulated RAW264.7 macrophages. Carbohydrate Polymers 85(1): 80-85.
- Senni K, Gueniche F, Foucault-Bertaud A, Igondjo-Tchen S, Fioretti F, et al. (2006) Fucoidan a sulfated polysaccharide from brown algae is a potent modulator of connective tissue proteolysis. Arch Biochem Biophys 445: 56-64.
- Leiro JM, Castro R, Arranz JA, Lamas J (2007) Immunomodulating activities of acidic sulphated polysaccharides obtained from the seaweed Ulva rigida C Agardh. Int Immunopharmacol 7(7): 879-888.
- Bae SY, Yim JH, Lee HK, Pyo S (2006) Activation of murine peritoneal macrophages by sulphated exopolysaccharide from marine microalga Gyrodinium impudicum (strain KG03): Involvement of the NF-kappa B and JNK pathway. Int Immunopharmacol 6: 473-484.
- Choi EM, Kim AJ, Kim YO, Hwang JK (2005) Immunomodulating activity of arabinogalactan and fucoidan in vitro. J Med Food 8: 446-453.
- Maruyamaa H, Tamauchib H, Hashimotoc M, Nakano T (2005) Suppression of Th2 immune responses by Mekabu fucoidan from Undaria pinnatifida sporophylls. Int Arch Allergy Immunol 137(4): 289-294.
- Yoon SJ, Pyun YR, Hwang JK, Mourão PAS (2007) A sulfated fucan from the brown alga Laminaria cichorioides has mainly heparin cofactor II-dependent anticoagulant activity. Carbohydr Res 342(15): 2326-2330.
- Guzman S, Gato A, Lamela M, Freire Garabal M, Calleja JM (2003) Anti-Inflammatory and immunomodulatory activities of polysaccharide from Chlorella stigmatophora and Phaeodactylum tricornutum. Phytother Res 17(6): 665-670.
- Chen Z, Soo MY, Srinivasan N, Tan BKH, Chan SH (2009) Activation of macrophages by polysaccharide-protein complex from Licium barbarum. Phytother Res 23: 1116-1122.
- Karnjanapratum S, Tabarsa M, Cho M, You SG (2012) Characterization and immunomodulatory activities of sulfated polysaccharides from Capsosiphon fulvescens. International Journal of Biological Macromolecules 51(5): 720-729.
- Kim IG, Jung IL, Oh TJ, Kim KC, Shim HW (2002) Polysaccharide-enriched fraction isolated from Duchesnea chrysantha protects against oxidative damage. Biotechnology Letters 24(16): 1299-1305.
- Chen D, Wu XZ, Wen ZY (2008) Sulfated polysaccharides and immune response: Promoter or inhibitor? Panminerva Med 50: 177-183.
- Zhou G, Sun Y, Xin H, Zhang Y, Li Z, et al. (2004) In vivo antitumor and immunomodulation activities of different molecular weight lambda-carrageenans from Chondrus ocellatus. Pharmacol Res 50(1): 47-53.
- Tsuji RF, Hoshino K, Noro Y, Tsuji NM, Kurokawa T, et al. (2003) Suppression of allergic reaction by lambda-carrageenan: Toll-like receptor 4/MyD88-dependent and -independent modulation of immunity. Clin Exp Allergy 33: 249-258.
- Sun L, Wang L, Zhou Y (2012) Immunomodulation and antitumor activities of different molecular weight polysaccharides from Porphyridium cruentum. Carbohydr Polym 87(2): 1206-1210.
- Shin KH, Lim SS, Lee S, Lee YS, Jung SH, et al. (2003) Anti-tumour and immuno-stimulating activities of the fruiting bodies of Paecilomyces japonica, a new type of Cordyceps spp. Phytotherapy Res,17: 830-833.
- Czaja WK, Young DJ, Kawecki M, Brown RM (2007) The future prospects of microbial cellulose in biomedical applications. Biomacromolecules 8(1): 1-12.
- Heneji K, Matsuda T, Tomita M, Kawakami H, Ohshiro K, et al. (2005) Fucoidan extracted from Cladosiphon okamuranus Tokida induces apoptosis of human T-cell leukemia virus type 1-infected T-cell lines and primary adult T-cell leukemia cells. Nutr Cancer 52(2): 189-201.
- Athukorala Y, Ahn GN, Jee YH, Kim GY, Kim SH, et al. (2009) Antiproliferative activity of sulfated polysaccharide isolated from an enzymatic digest of Ecklonia cava on the U-937 cell line. J Appl Phycol 21: 307-314.
- Chen WS, Lazar CS, Poenie M, Tsien RY, Gill GN, et al. (1987) Requirement for intrinsic protein tyrosine kinase in the immediate and late actions of the EGF receptor. Nature 328: 820-823.
- Lee NY, Ermakova SP, Choi HK, Kusaykin MI, Shevchenko NM, et al. (2008) Fucoidan from Laminaria cichorioides inhibits AP-1 transactivation and cell transformation in the mouse epidermal JB6 cells. Mol Carcinog 47(8): 629-637.
- Khotimchenko YS (2010) Antitumor properties of non-starch polysaccharides: Fucoidans and Chitosans. Rus. Russian Journal of Marine Biology 36(5): 321-330.
- Yamamoto I, Nagumo T, Takahashi M, Fujihara M, Suzuki Y, et al. (1981) Antitumor effect of seaweeds, 3. Antitumor effect of an extract from Sargassum kjellmanianum. Jpn J Exp Med 51: 187-189.
- Yamamoto I, Takahashi M, Tamura E, Maruyama H, Mori H (1984) Antitumor activity of edible marine algae: Effect of crude fucoidan fractions prepared from edible brown seaweed against L-1210 leukemia. Hydrobiology 116/117:145-148.
- Yamamoto I, Nagumo T, Yagi K, Tominaga H, Aoki M (1974) Antitumor effect of seaweeds, 1. Antitumor effect of extract from Sargassum and Laminaria. Jpn. J Exp Med 44: 543-546.
- Aisa Y, Miyakawa Y, Nakazato T, Shibata H, Saito K, et al. (2005) Fucoidan induces apoptosis of human HS-sultan cells accompanied by activation of caspase-3 and down-regulation of ERK pathways. Am J Hematol 78: 7-14.
- Yamasaki-Miyamoto Y, Yamasaki M, Tachibana H, Yamada K (2009) Fucoidan induces apoptosis through activation of caspase-8 on human breast cancer MCF-7 cells. J Agric Food Chem 57(18): 8677-8682.
- Maruyama H, Tamauchi H, Iizuka M, Nakano T (2006) The role of NK cells in antitumor activity of dietary fucoidan from Undaria pinnatifida sporophylls (Mekabu). Planta Med 72(15): 1415-1417.
- Zhang Q, Li N, Zhao T, Qi H, Xu Z, et al. (2005) Fucoidan inhibits the development of proteinuria in active Heymann nephritis. Phytother. Res 19(1): 50-53.
- Teruya T, Tatemoto H, Konishi T, Tako M (2009) Structural characteristics and in vitro macrophage activation of acetyl fucoidan from Cladosiphon okamuranus. Glycoconj J 26(8): 1019-1028.
- Raghavendran HR, Srinivasan P, Rekha S (2011) Immunomodulatory activity of fucoidan against aspirin-induced gastric mucosal damage in rats. Int Immunopharmacol 11: 157-163.
- Alekseyenko TV, Zhanayeva SY, Venediktova AA, Zvyagintseva TN, Kuznetsova TA, et al. (2007) Antitumor and antimetastatic activity of fucoidan, a sulfated polysaccharide isolated from the Okhotsk sea Fucus evanescens brown alga. Bull Exp Biol Med 143: 730-732.
- Jun Shimizu, Urara Wada-Funada, Hiroshi Mano, Yoshiharu Matahira, Mitsuaki Kawaguchi, et al. (2005) Proportion of murine cytotoxic T cells is increased by high molecular weight fucoidan extracted from Okinawa mozuku (Cladosiphon okamuranus). J Health Sci 51: 394-397.
- Rocha HA, Franco CR, Trindade ES, Veiga SS, Leite EL, et al. (2005) Fucan inhibits Chinese hamster ovary cell (CHO) adhesion to fibronectin by binding to the extracellular matrix. Planta Med 71: 628-633.
- Miao HQ, Elkin M, Aingorn E, Ishai-Michaeli R, Stein CA, et al. (1999) Inhibition of heparanase activity and tumor metastasis by laminarin sulfate and synthetic phosphorothioate oligodeoxynucleotides. Int J Cancer 83(3): 424-431.
- Namikoshi M (1996) Bioactive compounds produced by cyanobacteria. J Int Microbiol Biotechnol 17(5-6): 373-384.
- Yim JH, Son E, Pyo S, Lee HK (2005) Novel sulfated polysaccharide derived from red-tide microalga Gyrodinium impudicum strain KG03 with immunostimulating activity in vivo. Mar Biotechnol 7(4): 331-338.
- Rioux LE, Turgeon SL, Beaulieu M (2010) Structural characterization of laminaran and galactofucan extracted from the brown seaweed Saccharina longicruris. Phytochemistry 71: 1586-1595.
- Yang C, Chung D, Shin IS, Lee HY, Kim JC, et al. (2008) Effects of molecular weight and hydrolysis conditions on anticancer activity of fucoidans from sporophyll of Undaria pinnatifida. Int J Biol Macromol 43(5): 433-437.
- Hobbs C (2000) Medicinal value of Lentinus edodes (Berk.) Sing. (Agaricomycetideae). A literature reviews. International Journal of Medicinal Mushrooms 2(4): 287-302.
- Wasser SP, Weis AL (1997) Shiitake mushrooms [Lentinus edodes (Berk.) Sing]. In E Nevo (Ed.), Medicinal mushrooms. Haifa, Israe: Peledfus.
- Ohno N, Furukawa M, Miura NN, Adachi Y, Motoi M, et al. (2001) Antitumor b-glucan from the cultured fruit body of Agaricus blazei. Biological & Pharmaceutical Bulletin 24: 820-828.
- Aoki T (1984) Lentinan. In: Fenichel RL, Chirgis MA (Eds.), Immune modulation agents and their mechanisms. Immunol Stud 25: 62-77.
- Moradali M, Mostafavi H, Ghods S, Hedjaroude G (2007) Immunomodulating and anticancer agents in the realm of macromycetes fungi (macrofungi). Inter Immunopharmaco 7(6): 701-724.
- Lotzova E, Tsubura E (1989) Effector functions of cytokines in cancer. In JW Hadden, F Spreafico, Y Yamamura & K Frank Austen (Eds), Adv in immunopharmaco 4: 245-250.
- Athukorala Y, Lee KW, Kim SK, Jeon YJ (2007) Anticoagulant activity of marine green and brown algae collected from Jeju Island in Korea. Bioresour Technol 98: 1711-1716.
- Maeda M, Uehara T, Harada N, Sekiguchi M, Hiraoka A (1991) Heparinoid-active sulfated polysaccharide from Monostroma-nitidum and their distribution in the Chlorophyta. Phytochemistry 30(11): 3611-3614.
- Mao W, Li H, Li Y, Zhang H, Qi X, et al. (2009) Chemical characteristic and anticoagulant activity of the sulfated polysaccharide isolated from Monostroma latissimum (Chlorophyta). Int J Biol Macromol 44(1): 70-74.
- Hayakawa Y, Hayashi T, Hayashi K, Osawa T, Niiya K, Sakuragawa N (2000) Activation of heparin cofactor II by calcium spirulan J Biol Chem 275(15): 11379-11382.
- Shanmugam M, Mody KH, Ramavat BK, Murthy ASK, Siddhanta AK (2002) Screening of Codiacean algae (Chlorophyta) of the Indian coasts for blood anticoagulant activity. Indian J Mar Sci 31(1): 33-38.
- Matsubara K, Matsuura Y, Bacic A, Liao ML, Hori K, et al. (2001) Anticoagulant properties of a sulfated galactan preparation from a marine green alga, Codium cylindricum. Int J Biol Macromol 28(5): 395-399.
- Matsubara K, Matsuura Y, Hori K, Miyazawa K (2000) An anticoagulant proteoglycan from the marine green alga, Codium pugniformis. J Appl Phycol 12: 9-14.
- Li H, Mao W, Hou Y, Gao Y, Qi X, et al. (2012a) Preparation, structure and anticoagulant activity of a low molecular weight fraction produced by mild acid hydrolysis of sulfated rhamnan from Monostroma latissimum. Bioresour Technol 114: 414-418.
- Mao W, Zang X, Li Y, Zhang H (2006) Sulfated polysaccharides from marine green algae Ulva conglobata and their anticoagulant activity. J Appl Phycol 18(1): 9-14.
- Rodrigues JAG, Oliveira Vanderlei EDS, Bessa EF, Magalhaes FDA, Monteiro de Paula, et al. (2011) Anticoagulant activity of a sulfated polysaccharide isolated from the green seaweed Caulerpa cupressoides. Braz Arch Biol Technol 54: 691-700.
- Dobashi K, Nishino T, Fujihara M, Nagumo T (1989) Isolation and preliminary characterization of fucose-containing sulfated polysaccharides with blood-anticoagulant activity from seaweed Hizikia fusiforme. Carbohydr Res 194: 315-320.
- Melo FR, Pereira MS, Foguel D, Mourão PAS (2004) Antithrombin-mediated anticoagulant activity of sulfated polysaccharides. J Biol Chem 279(20): 20824-20835.
- Silva FRF, Dore CMPG, Marques CT, Nascimento MS, Benevides NMB, et al. (2010) Anticoagulant activity, paw edema and pleurisy induced carrageenan: Action of major types of commercial carrageenans. Carbohydr Polym 79: 26-33.
- Chevolot L, Foucault A, Chaubet F, Kervarec N, Sinquin C, et al. (1999) Further data on the structure of brown seaweed fucans: Relationships with anticoagulant activity. Carbohydr Res 319: 154-165.
- Chevolot L, Mulloy B, Racqueline JA (2001) Disaccharide repeat unit is the structure structure in fucoidans from two species of brown algae. Carbohydr Res 330: 529-535.
- Chandía NP, Matsuhiro B (2008) Characterization of a fucoidan from Lessonia vadosa (Phaeophyta) and its anticoagulant and elicitor properties. Int J Biol Macromol 42: 235-240.
- Zhang HJ, Mao WJ, Fang F, Li HY, Sun HH, et al. (2008) Chemical characteristics and anticoagulant activities of a sulfated polysaccharide and its fragments from Monostroma latissimum. Carbohydr. Polym 71: 428-434.
- Nishino T, Aizu Y, Nagumo T (1991) The influence of sulfate content and molecular weight of a fucan sulfate from the brown seaweed Ecklonia kurome on its antithrombin activity. Thromb Res 64(6): 723-731.
- Rocha HA, Moraes FA, Trindade ES, Franco CR, Torquato RJ, et al. (2005) Structural and haemostatic activities of a sulfated galactofucan from the brown alga Spatoglossum schrö An ideal antithrombotic agent. J Biol Chem 280: 41278-41288.
- Grauffel V, Koareg B, Mabeau S, Duran P, Jozefonvicz J (1989) New natural polysaccharides with potent antithrombotic activity: Fucans from brown algae Biomaterials 10(6): 363-368.
- Kindness G, Williamson FB, Long WF (1979) Effect of polyanetholesulfonic acid and xylan sulphate on antithrombin III activity. Biochem Biophys Res Commun 88(3): 1062-1068.
- Pereira MS, Mulloy B, Mourão PA (1999) Structure and anticoagulant activity of sulfated fucans. Comparison between the regular, repetitive, and linear fucans from echinoderms with the more heterogeneous and branched polymers from brown algae. J Biol Chem 274: 7656-7667.
- Xiangdong Qiu, Ananda Amarasekara, Vasant Doctor (2006) Effect of oversulfation on the chemical and biological properties of fucoidan. Carbohydr Polym 63: 224-228.
- Jung WK, Athukorala Y, Lee YJ, Cha SH, Lee CH, et al. (2007) Sulfated polysaccharide purified from Ecklonia cava accelerates antithrombin III-mediated plasma proteinase inhibition. Journal of Applied Phycology 19(5): 425-430.
- Nishino T, Nagumo T (1992) Anticoagulant and antithrombotic activities of oversulfated fucans. Carbohydr Res 229(2): 355-362.
- Minix R, Doctor VM (1997) Interaction of fucoidan with proteases and inhibitors of coagulation and fibrinolysis. Thromb Res 87(5): 419-429.
- De Candia E, De Cristofaro R, Landolfi R (1999) Thrombin-induced platelet activation is inhibited by high-and low-molecular-weight heparin. Circulation 99: 3308-3314.
- Farias WR, Valente AP, Pereira MS, Mourão PAS (2000) Structure and anticoagulant activity of sulfated galactans. Isolation of a unique sulfated galactan from the red algae Botryocladia occidentalis and comparison of its anticoagulant action with that of sulfated galactans from invertebrates. J Biol Chem 275: 29299-29307.
- Mourão PAS, Pereira MS, Pavão MSG, Mulloy B, Tollefsen DM, et al. (1996) Structure and anticoagulant activity of a fucosylated chondroitin sulfate from echinoderm: Sulfated fucose branches on the polysaccharide account for its high anticoagulant action. J Biol Chem 271(39): 23973-23984.
- Pereira MS, Vilela-Silva AC, Valente AP, Mourão PA (2002) A 2-sulfated, 3-linked α-L-galactan is an anticoagulante polysaccharide. Carbohydr Res 337: 2231-2238.
- Glauser BF, Pereira MS, Monteiro RQ, Mourão PAS (2008) Serpin-independent anticoagulant activity of a fucosylated chondroitin sulfate. Thromb Haemost 100(3): 420-428.
- Glauser BF, Rezende RM, Melo FR, Pereira MS, Francischetti IMB, et al. (2009) Anticoagulant activity of a sulfated galactan: Serpin-independent effect and specific interaction with factor Xa. Thromb Haemost. 102(6): 1183-1193.
- Pereira MS, Melo FR, Mourão PA (2002) Is there a correlation between structure and anticoagulant action of sulfated galactans and sulfated fucans. Glycobiology 12: 573-580.
- Fonseca RJC, Oliveira SNMCG, Pomin VH, Mecawi AS, Iracema G, et al. (2010) Effects of oversulfated and fucosylated chondroitin sulfates on coagulation, Challenges for the study of anticoagulant polysaccharides. Thromb Haematol 103: 994-1004.
- Melo FR, Mourão PAS (2008) Sulfated galactan has an unusual effect on thrombosis due to activation of factor XII and inhibition of the coagulation proteases. Thromb Haemost 99(3): 531-538.
- Liu T, Scallan CD, Broze GJ, Patarroyo White S, Pierce GF, et al. (2006) Improved coagulation in bleeding disorders by non-anticoagulant sulfated polysaccharides (NASP). Thromb Haemostas 95(1): 68-76.
- Zhang Z, Till S, Jiang C, Knappe S, Reutterer S, et al. (2014) Structure-activity relationship of the pro- and anticoagulant effects of Fucus vesiculosus fucoidan. Thromb. Haemost 111(3): 429-437.
- Yu PZ, Li N, Liu XG, Zhou GF, Zhang QB, et al. (2003) Antihyperlipidemic effects of different molecular weight sulfated polysaccharides from Ulva pertusa (Chlorophyta). Pharmacol Res 48(6): 543-549.
- Yu PZ, Zhang QB, Li N, Xu ZH, Wang YM, et al. (2003) Polysaccharides from Ulva pertusa (Chlorophyta) and preliminary studies on their antihyperlipidemia activity. J Appl Phycol 15(1): 21-27.
- Qi H, Huang L, Liu X, Liu D, Zhang Q, et al. (2012) Antihyperlipidemic activity of high sulfate content derivative of polysaccharide extracted from Ulva pertusa (Chlorophyta). Carbohydr Polym 87: 1637-1640.
- Qi H, Liu X, Zhang J, Duan Y, Wang X, et al. (2012) Synthesis and antihyperlipidemic activity of acetylated derivative of ulvan from Ulva pertusa. Int J Biol Macromol 50: 270-272.
- Sathivel A, Raghavendran HR, Srinivasan P, Devaki T (2008) Anti-peroxidative and anti-hyperlipidemic nature of Ulva lactuca crude polysaccharide on D-Galactosamine induced hepatitis in rats. Food Chem Toxicol 46: 3262-3267.
- Balaji Raghavendra Rao H, Sathivel A, Devaki T (2004) Antihepatotoxic nature of Ulva reticulata (Chlorophyceae) on acetaminophen-induced hepatoxicity in experimental rats. J Med Food 7: 495-497.
- Charles AL, Chang CK, Wu ML, Huang TC (2007) Studies on the expression of liver detoxifying enzymes in rats fed seaweed (Monostroma nitidum). Food and Chemical Toxicology 45: 2390-2396.
- Lahaye M (1991) Marine algae as sources of fibres: Determination of soluble and insoluble dietary fibre contents in some “sea vegetables. J Sci Food Agric 54(4): 587-594.
- Teng Z, Qian L, Zhou Y (2013) Hypolipidemic activity of the polysaccharides from Enteromorpha prolifera. Int J Biol Macromol 62: 254-256.
- Li Y, Xu Z, Zhang SH (1999) Prevention and cure of fucoidan of L japonica on mice with hypercholesterolemia. Food Sci 20: 45-46.
- Ren D, Noda H, Amano H, Nishino T, Nishizawa K, et al. (1994) Study on antihypertensive and antihyperlipidemic effects of marine algae. Fish Sci 60: 33-40.
- Tsuge K, Okabe M, Yoshimura T, Sumi T, Tachibana H, et al. (2004) Dietary effect of porphyran from Porphyra yezoensis on growth and lipid metabolism of Sprague-Dawley rats. Food Sci Technol Res 10: 147-151.
- Inoue N, Yamano N, Sakata K, Nagao K, Hama Y, et al. (2009) The sulfated polysaccharide porphyran reduces apolipoprotein B100 secretion and lipid synthesis in HepG2 cells. Biosci Biotechnol Biochem 73(2): 447-449.
- Panlasigui LN, Baello OQ, Dimatangal JM, Dumelod BD (2003) Blood cholesterol and lipid-lowering effects of carrageenan on human volunteers. Asia Pac J Clin Nutr 12: 209-214.
- Reddy BS, Watanabe K, Sheinfil A (1980) Effect of dietary wheat bran, alfalfa, pectin and carrageenan on plasma cholesterol and fecal bile acid and neutral sterol excretion in rats. J Nutr 110: 1247-1254.
- Dvir I, Stark AH, Chayoth R, Madar Z, Arad SM (2009) Hycholesterolemic effects of nutraceuticals produced from the red microalga Porphyridium sp. in rats. Nutrients 1: 156-167.
- Dvir I, Maislos M, Arad SM (1995) Feeding rodents with red microalgae. In Dietary Fiber, Mechanisms of Action in Human Physiology and Metabolism; Cherbut C, Barry JL, Lairon D, Durand M (Eds.) John Libbey Eurotext: Paris, France, pp: 86-91.
- Ginzberg A, Cohen M, Sod Moriah UA, Shany S, Rosenshtrauch A, et al. (2000) Chickens fed with biomass of the red microalga Porphyridium sp. have reduced blood cholesterol levels and modified fatty acids composition in egg yolk. Journal of Applied Phycology 12(3-5): 325-330.
- Huang J, Liu L, Yu Y, Lin W, Chen B, et al. (2006) Reduction in the blood glucose level of exopolysaccharide of Porphyridium cruentum in alloxan-induced diabetic mice. J Fujian Norm Univ 22: 77-80.
- Glore SR, van Treeck D, Knehans AW, Guild M (1994) Soluble fiber in serum lipids: A literature review. J Am Diet Assoc 94(4): 425-436.
- Marlett J (2001) Dietary fibre and cardiovascular disease. In Handbook of Dietary Fibers; Cho SS, Dreher MD, Eds, Marcel Dekker: New York, NY, USA, pp. 17-30.
- Dvir I, Chayoth R, Sod Moriah U, Shany S, Nyska A, et al. (2000) Soluble polysaccharide of red microalga Porphyridium spalters intestinal morphology and reduces serum cholesterol in rats. Br J Nutr 84: 469-476.
- Lupescu N, Geresh S, Arad SM, Bernstein M, Glaser R (1991) Structure of some sulfated sugars isolated after acid hydrolysis of the extracellular polysaccharide of Porphyridium sp. unicellular red alga. Carbohydr Res 210: 349-352.
- Guillon F, Champ M (2000) Structural and physical properties of dietary fibres, and consequences of processing on human physiology. Food Research International 33(3-4): 233-245.
- Barcelo A, Claustre J, Moro F, Chayvaille JA, Cuber JC, et al. (2000) Mucin secretion is modulated by luminal factors in the isolated vascularly perfused rat colon. Gut 46: 218-224.
- Ohta T, Sasaki S, Oohori T, Yoshikawa S, Kurihara H, et al. (2002) β-Glucosidase inhibitory activity of a 70% methanol extract from ezoishe (Pelvetia babingtonii de Toni) and its effect on the elevation of blood glucose level in rats. Biosci Biotechnol Biochem 66: 1552-1554.
- Fu XY, Xue CH, Ning Y, Li ZJ, Xu JC (2004) Acute antihypertensive effects of fucoidan oligosaccharides prepared from Laminaria japonica on renovascular hypertensive rat. Journal of Ocean University of China (Natural Science) 34(4): 560-564.
- Barud Hda S, De Araujo AM, Saska S, Mestieri LB, Campos JA, et al. (2013) Antimicrobial Brazilianpropolis (EPP-AF) containing biocellulose membranes as promising biomaterial forskin wound healing. Evid Based Complement Alternate Med pp: 703024.
- Hrynyk M, Martins Green M, Barron AE, Neufeld RJ (2012) Alginate-PEG sponge architecture and role in the design of insulin release dressings. Biomacromolecules 13(5): 1478-1485.
- Anilkumar TV, Muhamed J, Jose A, Jyothi A, Mohanan PV, et al. (2011) Advantages of hyaluronic acid as a component of fibrin sheet for care of acute wound. Biologicals 39(2): 81-88.
- Chen WY, Abatangelo G (1999) Functions of hyaluronan in wound repair. Wound Repairand Reg 7(2): 79-89.
- Liakos I, Rizzello L, Bayer IS, Pompa PP, Cingolani R, et al. (2013) Controlled antiseptic release by alginate polymer films and beads. Carbohydr Polym 92(1): 176-183.
- Gu Z, Xie H, Huang C, Li L, Yu X (2013) Preparation of chitosan/silk fibroin blending membrane fixed with alginate dialdehyde for wound dressing. Int J Biol Macromol 58: 121-126.
- Li X, Chen S, Zhang B, Li M, Diao K, et al. (2012b) In situ injectable nano-composite hydrogel composed of curcumin, N,O-carboxymethy lchitosan and oxidized alginate for wound healing application. Inter J of Pharmaceutics 437(1-2): 110-119.
- Singh R, Singh D (2012) Radiation synthesis of PVP/alginate hydrogel containing nanosilver as wound dressing. Journal of Materials Science Materials in Medicine 23(11): 2649-2658.
- Kondo, S, Niiyama H, Yu A, Kuroyanagi Y (2011) Evaluation of a wound dressing composed of hyaluronic acid and collagen sponge containing epidermal growth factor in diabeticmice. J Biomater Sci Polym Ed 23(13): 1729-1740.
- Matsumoto Y, Kuroyanagi Y (2010) Development of a wound dressing composed of hyaluronic acid sponge containing arginine and epidermal growth factor. J of Biomater Sci Polymer Ed 21(6-7): 715-726.
- Duan XJ, Zhang WW, Li XM, Wang BG (2006) Evaluation of antioxidant property of extract and fractions obtained from a red alga, Polysiphonia urceolata. Food Chem 95: 37-43.
- Halliwell B, Gutteridge JM (1999) Free Radicals in Biology and Medicine III. Oxford University Press: Oxford, UK.
- Jiang Y, Wang H, Lu L, Tian GY (2005) Chemistry of polysaccharide Lzps-1 from Ganoderma lucidum spore and anti-tumor activity of its total polysaccharides. Yao Xue Xue Bao 40(4): 347-350.
- Ng TB, Pi ZF, Yue H, Zhao L, Fu M, et al. (2006) A polysaccharopeptide complex and a condensed tannin with antioxidant activity from dried rose (Rosa rugosa) flowers. J Pharm Pharmacol 58(4): 529-534.
- Kodali VP, Sen R (2008) Antioxidant and free radical scavenging activities of an exopolysaccharide from a probiotic bacterium. Biotechnol J 3(2): 245-251.
- Wang J, Zhang Q, Zhang Z, Li Z (2008) Antioxidant activity of sulfated polysaccharide fractions extracted from Laminaria japonica. Int J Biol Macromol 42(2): 127-132.
- Costa MSSP, Costa LS, Cordeiro SL, Almeida Lima J, Dantas-Santos N, et al. (2012) Evaluating the possible anticoagulant and antioxidant effects of sulfated polysaccharides from the tropical green alga Caulerpa cupressoides var. flabellata. J Appl Phycol 24: 1159-1167.
- Kim SH, Choi DS, Athukorala Y, Jeon YJ, Senevirathne M, et al. (2007) Antioxidant activity of sulfated polysaccharides isolated from Sargassum fulvellum. Preventive Nutrition and Food Science 12(2): 65-73.
- Zhang QB, Li N, Zhou GF, Lu XL, Xu ZH, Li Z (2003) In-vivo antioxidant activity of polysaccharide fraction from Porphyra haitanensis (Rhodophyta) in aging mice. Pharmacol Res 48(2): 151-155.
- Cho ML, Lee HS, Kang IJ, Won MH, You SG (2011) Antioxidant properties of extract and fractions from Enteromorpha prolifera, a type of green seaweed. Food Chem 127: 999-1006.
- Qi H, Zhang Q, Zhao T, Chen R, Zhang H, et al. (2005) Antioxidant activity of different sulfate content derivatives of polysaccharide extracted from Ulva pertusa (Chlorophyta) in vitro. Int J Biol Macromol 37: 195-199.
- Chen B, You B, Huang J, Yu Y, Chen W (2010) Isolation and antioxidant property of the extracellular polysaccharide from Rhodella reticulata. World J Microbiol Biotechnol 26: 833-840.
- Tannin Spitz T, Bergman M, van Moppes D, Grossman S, Arad SM (2005) Antioxidant activity of the polysaccharide of the red microalga Porphyridium sp. J Appl Phycol 17(3): 215-222.
- Sun L, Wang C, Shi Q, Ma C (2009) Preparation of different molecular weight polysaccharides from Porphyridium cruentum and their antioxidant activities. Int J Biol Macromol 45(1): 42-47.
- Challouf R, Trabelsi L, Dhieb RB, El Abed O, Yahia A, et al. (2011) Evaluation of cytotoxicity and biological activities in extracellular polysaccharides released by cyanobacterium Arthrospira platensis. Braz Arch Biol Technol 54: 831-838.
- Qi HM, Zhao TT, Zhang QB, Li Z, Zhao ZQ, et al. (2005) Antioxidant activity of different molecular weight sulfated polysaccharides from Ulva pertusa Kjellm (Chlorophyta). J Appl Phycol 17: 527-534.
- Shao P, Chen X, Sun P (2013) In vitro antioxidant and antitumor activities of different sulfated polysaccharides isolated from three algae. Int J Biol Macromol 62: 155-161.
- Wang X, Zhang Z, Yao Z, Zhao M, Qi H (2013) Sulfation, anticoagulant and antioxidant activities of polysaccharide from green algae Enteromorpha linza. Int J Biol Macromol 58: 225-230.
- Zhang Z, Wang X, Yu S, Yin L, Zhao M, Han Z (2011) Synthesized oversulfated and acetylated derivatives of polysaccharide extracted from Enteromorpha linza and their potential antioxidant activity. Int J Biol Macromol 49(5): 1012-1015.
- Chen HX, Zhang M, Qu ZS, Xie BJ (2007) Compositional analysis and preliminaly toxicological evaluation of a tea polysaccharide conjugate. J Agric Food Chem 55: 2256-2260.
- Ale MT, Maruyama H, Tamauchi H, Mikkelsen JD, Meyer AS (2011) Fucoidan from Sargassum sp. and Fucus vesiculosus reduces cell viability of lung carcinoma and melanoma cells in vitro and activates natural killer cells in mice in vivo. Int J Biol Macromol 49: 331-336.
- Burja AM, Banaigs B, Abou-Mansor E, Burgess JG, Wright PC (2001) Marine cyanobacteria-A prolific source of natural products. Tetrahedron 57: 9347-9377.
- Fonseca RJC, Santos GRC, Mourão PAS (2009) Effects of polysaccharides enriched in 2,4-disulfated fucose units on coagulation, thrombosis and bleeding, Practical and conceptual implications. Thromb Haemost 102: 829-836.
- Freitas F, Alves VD, Reis MA (2014) Bacterial polysaccharides: production and applications in cosmetic industry. Polysaccharides Pp. 1-24.
- Geresh S, Dubinsky O, Arad SM, Christian D, Glaser R (1990) Structure of 3-O- (α-D-glucopyranosyluronic acid)-L-galactopyranose, an aldobiuronic acid isolated from the polysaccharides of various unicellular red algae. Carbohydr Res 208: 301-305.
- Hayakawa Y, Hayashi T, Hayashi K, Osawa T, Niiya K, et al. (1996) Heparin cofactor II-dependent antithrombin activity of calcium spirulan. Blood Coagul Fibrinolysis 7(5): 554-560.
- Jiao L, Jiang P, Zhang L, Wu M (2010) Antitumor and immunomodulating activity of polysaccharides from Enteromorpha intestinalis. Biotechnol. Biotechnology and Bioprocess Engineering 15(3): 421-428.
- Jiménez Escrig A, Gómez Ordóñez E, Rupérez P (2011) Seaweed as a source of novel nutraceuticals: Sulfated polysaccharides and peptides. Adv Food Nutr Res 64: 325-337.
- Kim EJ, Park SY, Lee JY, Park JH (2010) Fucoidan present in brown algae induces apoptosis of human colon cancer cells. BMC Gastroenterol 10: 96-106.
- Li DY, Xu RY, Zhou WZ, Sheng XB, Yang AY, et al. (2002) Effects of fucoidan extracted from brown seaweed on lipid peroxidation in mice. Acta Nutrim Sin 24: 389-392.
- Li LY, Li LQ, Guo CH (2010) Evaluation of in vitro antioxidant and antibacterial activities of Laminaria japonica polysaccharides. J Med Plants Res 4(21): 2194-2198.
- Li SP, Zhang GH, Zeng Q, Huang ZG, Wang YT, et al. (2006) Hypoglycemic activity of polysaccharide, with antioxidation, isolated from cultured Cordyceps mycelia. Phytomedicine 13(6): 428-433.
- Li ZJ, Xue CH, Lin H (1999) The hypolipidemic effects and antioxidative activity of sulfated fucan on the experimental hyperlipidemia in rats. Acta Nutrim Sin 21: 280-283.
- Na YS, Kim WJ, Kim SM, Park JK, Lee SM, et al. (2010) Purification, characterization and immunostimulating activity of water-soluble polysaccharide isolated from Capsosiphon fulvescens. Int Immunopharmacol 10(3): 364-370.
- Guillaume Pierre, Valérie Sopena, Camille Juin, Amira Mastouri, Marianne Graber, et al. (2011) Antibacterial activity of a sulphated galactan extracted from the marine alga Chaetomorpha aerea against Staphylococcus aureus. Biotechnol Bioproc Eng 16: 937-945.
- Pulz O, Gross W (2004) Valuable products from biotechnology of microalgae. Appl Microbiol Biotechnol 65: 635-648.
- Raposo MF, de Morais RM, Bernardo de Morais AM (2013) Bioactivity and applications of sulphated polysaccharides from marine microalgae. Mar Drugs 11: 233-252.
- de Jesus Raposo MF, de Morais RM, de Morais AM (2013) Health applications of bioactive compounds from marine microalgae. Life Sci 93: 479-486.
- de Jesus Raposo MF, de Morais AM, de Morais RM (2015) Marine Polysaccharides from Algae with Potential Biomedical Applications. Mar Drugs 13: 2967-3028.
- Ribeiro AC, Vieira RP, Mourão PA, Mulloy B (1994) A sulfated α-L-fucan from sea cucumber. Carbohydr Res 255: 225-240.
- Rodrigues JAG, Lino de Queiroz IN, Gomes Quindere AL, Vairo BC, de Souza Mourao, PA, et al. (2011) An antithrombin-dependent sulfated polysaccharide isolated from the green alga Caulerpa cupressoides has in vivo anti- and prothrombotic effects. Cienc Rural 41: 634-639.
- Suarez ER, Syvitski R, Kralovec JA, Noseda MD, et al. (2006) Immunostimulatory polysaccharides from chlorella pyrenoidosa. A new Galactofuranan. Measurement of molecular weight and molecular weight dispersion by DOSY NMR. Biomacromolecules 7(8): 2368-2376.
- Sun LQ, Chu JL, Sun ZL, Chen LH (2016) Physicochemical properties, immunomodulation and antitumor activities of polysaccharide from Pavlova viridis. Life Sci 144: 156-161.
- Teruya T, Konishi T, Uechi S, Tamaki H, Tako M (2007) Anti-proliferative activity of oversulfated fucoidan from commercially cultured Cladosiphon okamuranus Tokida in U937 cells. Int J Biol Macromol 41(3): 221-226.
- Thomes P, Rajendran M, Pasanban B, Rengasamy R (2010) Cardioprotective activity of Cladosiphon okamuranus against isoproterenol induced myocardial infraction in rats. Phytomedicine 18(1): 52-57.
- Xing RG, Liu S, Yu HH, Guo ZY, Li Z, et al. (2005) Preparation of high-molecular weight and high-sulfate content chitosans and their potential antioxidant activity in vitro. Carbohydr. Polym 61: 148-154.
- Yoo YC, Kim WJ, Kim SY, Kim SM, Chung MK, et al. (2007) Immunomodulating activity of a fucoidan isolated from Korean Undaria pinnatifida sporophyll. Algae 22: 333-338.
- Zhao X, Xue CH, Li BF (2008) Study of antioxidant activities of sulfated polysaccharides from Laminaria japonica. J Appl Phycol 20: 431-436.
- Anisha BS, Biswas R, Chennazhi KP, Jayakumar R (2013) Chitosan-hyaluronic acid/ Nano silver composite sponges for drug resistant bacteria infected diabetic wounds. Int J of Biol Macromol 62: 310-320.
- Shao P, Chen X, Sun P (2013) In vitro antioxidant and antitumor activities of different sulfated polysaccharides isolated from three algae. Int J Biol Macromol 62: 155-161.
-
Ebtsam M El Kady, Mohsen S Asker, Mamal G Mahmoud. New Trends of the Polysaccharides as a Drug. World J Agri & Soil Sci. 3(5): 2019. WJASS.MS.ID.000572.
-
Polysaccharides, Drug, Biopolymers, Medical fibers, Fucoidan, Carrageenan, Laminarin
-
This work is licensed under a Creative Commons Attribution-NonCommercial 4.0 International License.