Research Article
Potential C and N Mineralisation of Shoot and Root Residues from Ten Grain Legume Species As Related to their Biochemical Characteristics
Maé Guinet*, Anne-Sophie Voisin and Bernard Nicolardot
Agroécologie, INRAE, Institut Agro, Univ. Bourgogne, Univ. Bourgogne Franche Comté, F-21000 Dijon, France
Corresponding AuthorMae Guinet, Institut Agro Dijon, Département 2A2E, 26 bd Dr Petitjean, BP 87999, 21079 Dijon cedex, France.
Received Date:January 17, 2023; Published Date:January 24, 2023
Abstract
Our objective was to compare potential C and N mineralisation of root and shoot residues for a wide range of grain legume species. Shoot and root residues from ten grain legume crops were collected either from a field experiment or from an outside pot experiment. Residues were incubated in soil for 112 days at 28°C under non-limiting N conditions. Biochemical characteristics of residues were determined and related to C and N residue mineralisation. Root and shoot residues decomposed rapidly after their incorporation into the soil. After 112 days of incubation, 41.8 to 71.5% of shoots-C and 33.5 to 57.9% of roots-C was mineralised. Most residues induced net N immobilisation at the beginning of incubation, while net N mineralisation occurred later during the incubation period. At the end of incubation -19.6 to 7.8% of shoots-N and -21.7 to 23.0% of roots-N was mineralised. The differences between shoots and roots residues decomposition and N mineralisation were explained by their biochemical characteristics, roots having higher N and lignin content and shoots higher soluble compounds contents, for all species. Differences between legume species in shoot and root N mineralisation were first explained by their C:N ratio and to a lesser extent by the lignin:N ratio and some biochemical fractions (i.e. cellulose, soluble compounds). Finally, these results can be used to parameterize decomposition models and help to predict C and N behaviour of the ten legume crop residues in soil.
Keywords:Soil incubation; Grain legumes; Roots; Shoots; Decomposition; C:N ratio; Biochemical characteristics
Introduction
Legumes can provide nitrogen (N) to the subsequent crop through residue N mineralisation and contribute to more sustainable agriculture, by notably allowing the reduction of N fertilisers [1,2]. Thus, legume pre-crops tend to induce higher grain yields of the following crop compared to cereal pre-crops [3]. Legume positive pre-crop effect is partially explained by N processes, as the amount of N derived from legume residues mineralisation is generally higher compared to cereals [4]. However, to avoid any yield loss due to N limitation, N fertilization of the following cereal is not always reduced by farmers. Indeed, there is a lack of robust references for a wide range of legumes species to accurately predict the amount of N derived from legume residue mineralisation and available N for the following crop [5].
After their incorporation in the soil, plant residues (i.e. shoots and roots) are decomposed by soil microflora. The rate of organic matter breakdown depends on the relative proportion of biochemical characteristics of residues. Indeed, soluble compounds and cellulose represent the rapidly decomposable fraction while hemicellulose and lignin are considered as more recalcitrant fractions to decomposition [6,7]. On the other hand, the composition of plant residues such as residue N concentration and the C:N ratio partially determine the balance between immobilisation vs. mineralisation processes by soil microbial flora [7].
In order to study the decomposition of plant material and quantify their potential N mineralisation into soil mineral N, soil incubations under controlled conditions, without limiting factors, have often been used. However, most of these incubation experiments focused on immature cover crops used as green manure or on a very limited number of mature residues of grain legume species, with quantifications mainly focused on pea (Pisum sativum L.) and soybean (Glycine max (L.) Merr.) [8,9]. The biochemical characteristics of plant tissues tend to change during crop growth [10]. As the plant matures, straw N concentration tends to decrease while the hemicellulose and lignin fractions increase [11]. Therefore, results obtained from legume cover crops N mineralisation can’t be transposed to grain legume residues harvested at maturity. As mature shoot residues biochemical characteristics may vary widely according to the leaf:stem ratio for example [12], differences in N mineralisation dynamics should be expected when considering a wide range of legume species.
In addition, several authors emphasised the importance of taking into account the contribution of the belowground compartment to better estimate N mineralisation of plant residues [e.g. 4,13,14] or contribution to C sequestration in soil [e.g. 15,16]. A few studies have measured the amount of N left over by roots after legume harvesting and estimated that 8 to 25% of total plant N was present in roots at maturity [16,17]. Moreover, root decomposition has received little attention compared to aboveground residues [18,19]. Most authors have studied root decomposition in field conditions [20,21] but few studies were carried out on mature legume root residues [9] while others focused on C or N mineralisation only [12,22]. Elsewhere very few studies have characterised potential C or N potential mineralisation of root legume residues using soil incubation in controlled conditions [9,20,23] and analysed the relationships with their composition [9,12]. Indeed, legume root decomposition could contribute to the release of significant amounts of N in the soil but with differences among species in root N mineralisation according to their biochemical characteristics.
Thus, our objectives were: i) to compare root and shoot residue C and N decomposition of a large range of grain legume species during soil incubations without limiting factors, and ii) to link the decomposition of root and shoot residues of those ten species to their biochemical characteristics.
Materials and Methods
Soils
The soil used for the incubation of legume shoots and roots was sampled (0-30 cm soil layer) in 2014 and 2016, respectively, at the INRAE experimental site of Bretenière close to Dijon (Eastern France; 47.241 N, 5.115 E, altitude = 206 m). The soil is classified as a Cambisol (Eutric) (WRB, 2006) with a clayey surface layer (depth = 0.65 ± 0.15 m) developed on an alluvial coarse layer. Soil characteristics were given previously [24]. Fresh soil was sieved at 6 mm and stored for a few days at ambient temperature before use.
Legume residues
The ten grain legume species studied were: chickpea (Cicer arietinum), common bean (Phaseolus vulgaris), common vetch (Vicia sativa), faba bean (Vicia faba), fenugreek (Trigolia foenum-graecum), lentil (Lens culinaris), lupin (Lupinus albus), pea (Pisium sativum), soybean (Glycine max) and Narbonne vetch (Vicia narbonensis). Legume shoot residues were obtained from field experiments in 2014 [24]. Shoots were harvested at plant maturity and separated from grain. Grain was not harvested for chickpea, fenugreek and Narbonne vetch, due to climatic conditions which were unsuitable for grain production. In these cases, the whole aerial part was considered as shoot residues. Due to the difficulty to collect roots in the field experiment (high clay soil) legume roots were obtained using outside pots experiment carried out in 2016. Legumes were grown using the same cultivars and Rhizobia strains as those used in the 2014 field experiment [24]. Seeds were calibrated to ensure homogenous weight. They were sown in 12 L pots filled with 60% attapulgite and 40% clay pebbles. Each pot contained four seeds of one legume species, with four replicates (for pots) per species. Pots were watered twice a day with a nutrient solution to avoid any water stress. The nutrient solution (0.16 mM KN03, 0.80 mM K2HPO 4, 0.24 mM Ca(NO3)2 + 4H2O, 1.00 mM MgSO4 + 7H2O, 2.27 mM CaCl2, 0.62 mM K2SO4, 0.20 mM NaCl) contained small amounts of N (0.625 mM) to reduce the risk of N deficiency during the early stages of plant growth, before the establishment of symbiotic N2 fixation. Legume plants were harvested at physiological maturity and roots were gently removed from the substrate and washed with water. Roots were not collected for fenugreek because it presented disease symptoms on shoots which greatly limited plant growth.
Legume residues characterisation
Table 1:Characteristics of root and shoot residues.
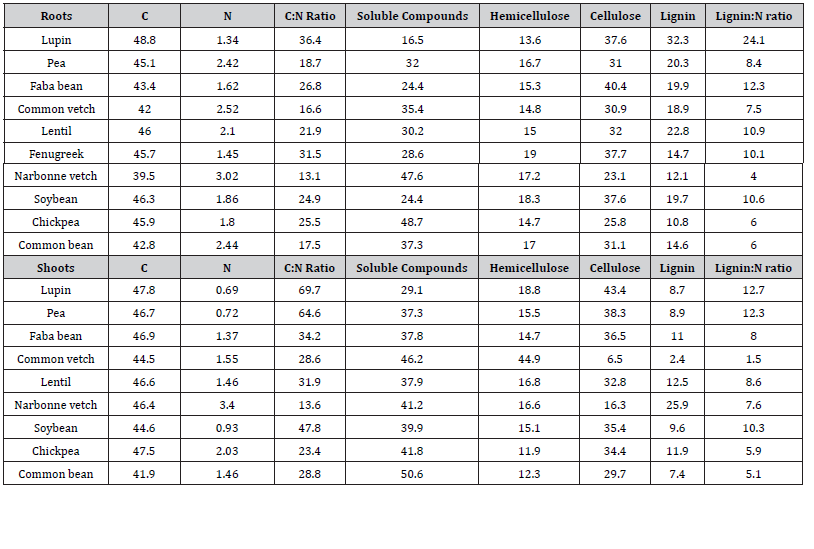
C, N, soluble compounds, hemicellulose, cellulose and lignin are expressed in % dry matter.
Shoot and root residues from the field and the outside pot experiments were oven-drying for 48 h at 80 °C. Residues were ground (< 1 mm) N and C concentrations were measured using an elemental analyser (ANCA-GLS, Sercon Ldt., Crewe, UK). Biochemical analyses were performed using a standard procedure [25] derived from the Van Soest’s method [26] to determine soluble compounds (SC), hemicellulose (HEM), cellulose (CEL) and lignin (LIG) fractions. Legume residue characteristics are given in Table 1.
Soil incubations
Shoot and root residues were incubated using the methods previously described [27] to assess potential decomposition using non-limiting conditions. Shoot and root residues (ground < 1 mm) were incorporated in soil collected in 2014 and 2016, respectively, at a rate of 8 g dry matter kg-1 dry soil. Soil samples (25 g equivalent dry soil) and crop residues were thoroughly mixed and placed in polyethylene pots before being incubated in 2 L glass jars to study soil N dynamics (4 replicates per treatment and sampling date; 10 polyethylene pots per jar) or in 250 mL plasma vials (4 replicates per treatment) to study C mineralisation. A treatment without any residue (control soil) was also included to measure C and N mineralisation from native soil organic matter (4 replicates for each sampling date for N; 4 replicates for C). To avoid any limitation in C decomposition and N immobilisation by microbial biomass due to soil inorganic N deficiency [11,27], a sufficient amount of inorganic N (> 20 mg N kg-1 dry soil) was added to the soil in KNO3 form, before the start of incubation. Soil moisture content was monitored to ensure a constant value during the whole period of incubation, corresponding to field capacity (34 g 100 g-1 dry sol). In plasma vials, CO2 produced during incubation was trapped by 10 mL of 0.25 M NaOH, traps being regularly replaced to renew the atmosphere in the vials and prevent saturation of the NaOH. In the 2 L glass jars, CO2 was trapped by 30 mL NaOH 1 M; traps being equally regularly renewed. The incubations were carried out for 112 days at a constant temperature of 28 °C.
CO2 determination
The CO2 produced by the soil and trapped as inorganic C by the 10 mL NaOH traps in the plasma vials was determined by a UV-persulfate TOC analyzer (Phoenix 8000, Tekmar-Dorman, Mason, Ohio, USA). Carbon decomposition from legume residues was calculated by subtracting the amount of CO2 produced by the control soil from the amount of CO2 produced by the soil + legume residue mixture and expressed in percentage of organic C added to the soil by the legume residues.
Soil inorganic N analysis
Inorganic N from the control soil and from the soil + crop residue mixtures were extracted after 0, 7, 14, 28, 58, 84, and 112 days of incubation using 1 M KCl (1 h shaking at ambient temperature, 25 g equivalent dry soil in presence of 100 mL KCl). After 1 h of sedimentation, 10 mL of supernatant solution were sampled and stored at -20 °C until analysis. Inorganic N (NH4+ and NO3-) from the extracted solution was measured by colorimetry using a Global 240 analyzer (BPC Biosed, Italy), using methods described previously [27]. Nitrogen dynamics of legume residues was calculated by differences between mineral N dynamics in control soil and soil + legume residue mixture and expressed in percentage of total N added to the soil by the legume residues.
Data analysis
Data analyses were performed using the R software version 4.0.2. [28]. ANOVA were performed to test the effects of species and plant organ, on C and N mineralisation after 112 days of incubation. For each plant organ, where species effect was significant, differences between pairs of species were tested using post-hoc pairwise multiple comparison with the {emmeans} package. Homoscedasticity and normality of residuals were tested using a Bartlett and Shapiro-Wilk, respectively, to reject the null hypothesis.
A Principal Component Analyses (PCA) was performed to test whether the biochemical characteristics of residues could account for the C and N mineralisation rates among species and plant organ (shoot and root). Samples were ordered through a Principal Component Analysis (PCA) using the Canoco 5.0 program [29]. Variables were standardized to obtain equal weights in the PCA.
Results
Shoot and root residue C mineralisation
Considering our range of ten legume species, CO2-C derived from residue mineralisation during soil incubation was on average higher for shoots (62.7%) compared to root residues (47.8%) (F(1,74)=49.4, p < 0.001) (Figure 1). Legume species had a significant effect on shoot residues C mineralisation at the end of incubation (i.e.112 days) (F(9,30)=12.2, p < 0.001) with values ranging from 41.8 to 77% for Narbonne vetch and pea, respectively (Figure 1A). At the end of incubation, root C mineralisation ranged from 33.5to 59.2% with a significant effect of species (F(8,27)=22.0, p < 0.001). Chickpea, faba bean and soybean had the highest root residues C mineralisation while lentil and Narbonne vetch had the lowest (Figure 1B).
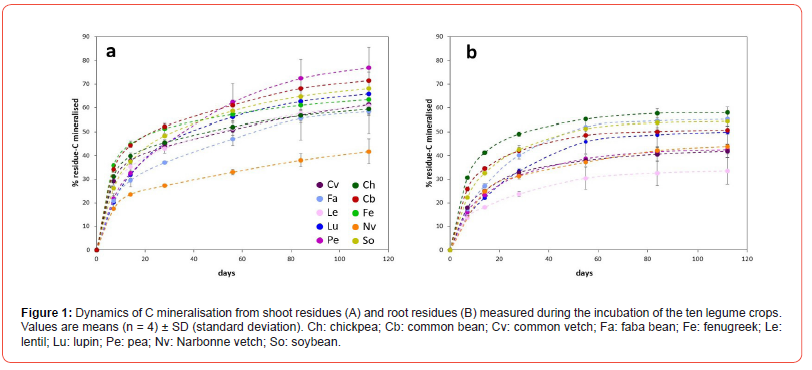
Shoot and root residue N mineralisation

Inorganic N derived from residue mineralisation during soil incubation widely varied among residues with contrasting dynamics over time (Figure 2). At the end of the incubation, residue N was on average slightly higher for roots (4%) compared to shoot residues (-2%,) (F(1,74)=5.2, p < 0.05). For shoot residues, the highest net N immobilisation was measured at day 14 for most species with values ranging from -1.1 to -64.6% of shoot-N for Narbonne vetch and lupin, respectively (Figure 2A). The highest net N immobilisation across time was observed at day 28 for soybean (-55.6%) and at day 56 for faba bean (-13%) and pea (-36%). Thereafter, net N mineralisation occurred during the remainder of the incubation period. At the end of incubation, legume species had a significant effect on shoot N mineralisation (F(9,30)=13.9, p < 0.001). Net N mineralisation values were obtained for chickpea, common bean, common vetch, fenugreek, lentil and Narbonne vetch while net N immobilisation still occurred for faba bean, lupin, pea and soybean. A similar trend was observed for root residues (Figure 2B) with an initial period of net N immobilisation followed by N mineralisation lasting later during the incubation. At the end of incubation, legume species had significant effect on root N mineralisation (F(8,27)=53.0, p < 0.001) with values ranging from -21% to 23% for lupin and Narbonne vetch, respectively.
Shoot and root decomposition as related to their composition
A PCA analysis was performed to assess the relationship between residue characteristics and their C and N mineralisation after 112 days of incubation in soil. First and second axis of the PCA accounting respectively for 53.8 and 27.8% of the total variance (Figure 3). The residue C:N ratio (C:N), the residue N content (%N) and the residue N mineralisation rate after 112 days (%Nmin) contributed most to the first axis. Nitrogen mineralisation rates of residues were negatively correlated with the residue C:N ratio and positively correlated with the residue N content. Four variables contributed most to the second axis with the residue N mineralisation rate after 112 days (%Cmin) negatively correlated with the residue lignin fraction (LIG) and the residue lignin: N ratio (LIG:N) and positively related with the residue soluble compound fraction(SC). A clear separation between root and shoot residues appeared, with a distinction mostly on axis 2 (Figure 3). Root residues presented higher lignin fractions and lower soluble compound fractions in comparison to shoot residues, except for Narbonne vetch and chickpea.
The strongest relationship was established between crop residue C:N ratio and their N mineralization at the end of the incubation (Figure 4). Net N mineralization occurred for residues with a C:N <25 while net immobilization was measured for residues with a C/N> 25. Moreover, for residues with C/N> 25, net N immobilization was higher for root residues compared to shoot residues, especially for faba bean and lupin.


Discussion
The originality of this work was to study the C and N potential mineralisation of roots and shoots for a wide range of legume species, for which data are still partial. For shoot and root residues, C and N mineralisation kinetics were similar to those of studies that conducted incubations of residues under controlled conditions. [9,11,12,27,30-32]. Nevertheless, in our study, differences between organs and legume species were observed. Consistent with studies that have compared roots and shoot residues [9,31-36], our results showed that C decomposition of roots was lower than shoots. The difference of C mineralisation between root and shoot residues of legumes can be explained by higher lignin content and lower soluble compounds and hemicellulose contents of roots compared to shoots. Several authors also emphasized the link between carbon decomposition and the lignin content of residues [9,30,31,34] or easily degradable compounds contents (i.e. soluble compounds, hemicellulose, cellulose) [12,27].
Shoot and root residue decomposition induced initial inorganic N net immobilisation during the first 28 days of incubation, with intensity widely varying among species. After this initial phase of N immobilisation, the mineralisation processes took over. In agreement with several authors [7,8,30,32], nitrogen mineralization of shoot and root residues at the end of incubation was positively correlated with residue N content and negatively correlated with the C:N ratio and to a lesser extent with the lignin:N ratio of residues.
Curiously, legume roots generally had higher N contents than shoot residues. The presence of nodules on the roots, even if not functional at the end of the legume cycle, may explain these high N contents that was associated on average with a slightly higher N mineralization compared to shoot residues. Many authors showed that the balance between net immobilisation and net mineralisation is related to residue C:N ratio, with net mineralisation occurring for C:N < 25 and net immobilisation for C:N > 25. In this study, a similar threshold was found for both roots and shoot residue at the end of the incubation. However, for residues with C/N> 25, net N immobilization was higher for root residues compared to shoot residues, probably due to higher lignin content and lower contents of decomposable compounds. Several studies have shown that roots of grain legumes have higher lignin contents than cereals, due to their tap root system with generally thicker roots [9,12]. This is particularly the case for faba bean and lupin, which have large tap roots rich in lignin and low N mineralisation compared to other legume species. In addition, incubation of residues under non-limiting N conditions may limit lignin-degrading enzymatic activity and thus reduce the decomposition of lignified residues [38]. Finally, shoot residues of legumes generally have lower C:N ratios than cereals. Nevertheless, in our study, the C:N ratios of aerial residues were highly variable among species with the lowest values measured for Narbonne vetch, fenugreek and chickpea and the highest values measured for lupin, pea and soybean. These differences may be due to the proportion of total nitrogen in the aerial parts that is exported to the seeds. Indeed, in the 2014 field experiment, lupin, pea, and soybean exported an average of 87% of aerial N to harvested grain (data not shown), compared to 68% for the other harvested grain legumes. Conversely, non-grain producing legumes (chickpea, fenugreek, and Narbonne vetch) had the lowest C:N ratios in shoot residues, with values close to those typically measured for non-mature legume residues [39,40].
Incubation of crop residues under controlled conditions allows to characterize the C and N potential mineralization without limiting factors. These results can be used to parameterize the residue decomposition modules in crop models to estimate the amount of N from residue mineralization in the field [8,11]. Moreover, the amount of N potentially mineralized in the field can be estimated by multiplying the amounts of N in residues measured in field experiments by the N mineralization rates measured under controlled conditions. In our study, the total amount of N in shoot residues accounted for 20-112 kg N ha-1, as described in [41], with corresponding values for roots ranging from 2-23 kg ha-1 taking into account the root-N:shoot-N ratio measured in the 2016 pot experiment (unpublished data). Based on these estimates, N mineralization would provide an average of -18 to 4 kg N ha-1 of N for aboveground residues and -5.9 to 3 kg N ha-1 for root residues after 28 days at 28 °C (i.e. 198 days at 11 °C for field conditions using the concept of normalized days) [42]. These amounts of potentially mineralizable N should however be considered with caution, because the soil incubations were conducted under non-limiting conditions. To translate these results to field conditions, the effect of (i) soil temperature and moisture on residue decomposition [42], (ii) mineral N availability in the soil [43], and (iii) residue-soil contact, which depends on residue particle size and soil porosity [44], should be considered. Although the decomposition of legume residues releases little or no short-term mineral N available for the following crop, residue N is incorporated into soil organic matter and can contribute to long-term soil N supply [45,46].
Conclusion
This study extended our knowledge of C and N mineralization of aerial and root residues for a wide range of seed legume species with contrasting biochemical characteristics. The high lignin content of roots compared to aerial residues induced a lower carbon mineralization of roots compared to aerial parts. Residue-N mineralization was negatively correlated with the C:N ratio and the lignin: N ratio of the residues. Unlike cover crops used as green manure, mature grain legume residues have a higher C:N ratio that may induce net N immobilization followed by net N mineralization. These results can be used to parameterize crop models to more accurately estimate the potential N supplied to the soil and then available to the following crop. This will help maximizing the synchronization between residue N mineralization and N uptake by the following crop through the implementation of adapted agricultural practices.
Acknowledgement
M. Guinet received a grant from the INRAE and the Ministry of Agriculture. This experimental work was supported by the ANR LEGITIMES, INRA Bourgogne-Franche-Comté Region (PSDR project ProSys), the Europeen Union’s Horizon 2020 research and innovation program under agreement No. 727247 (SolACE project) and Terres Inovia. We thank C. Caule and S. A. Augustin for their work on the incubation experiments during their internship of master’s degree. We also thank C. Ducourtieux, V. Durey and F. Lombard for their excellent technical assistance.
Conflict of Interest
The authors declare that they have no conflict of interest.
References
- Peoples MB, Hauggaard-Nielsen H, Jensen ES (2009) The potential environmental benefits and risks derived from legumes in rotations. In Emerich DW, Krishnan HB, Nitrogen fixation in crop production, Agronomy monograph 52. American Society of Agronomy, Madison, Wisconsin, USA. pp. 349-385.
- Voisin AS, Guéguen J, Huyghe C, Jeuffroy MH, Magrini MB, et al. (2014) Legumes for feed, food, biomaterials and bioenergy in Europe: a review. Agron Sustain Dev 34: 361-380.
- Cernay C, Makowski D, Pelzer E (2018) Preceding cultivation of grain legumes increases cereal yields under low nitrogen input conditions. Environ Chem Lett 16: 1-6.
- Evans J, McNeill AM, Unkovich MJ, Fettell NA, Heenan DP (2001) Net nitrogen balances for cool-season grain legume crops and contributions to wheat nitrogen uptake: a review. Aust J Exp Agric 41: 347-359.
- Preissel S, Reckling M, Schläfke N, Zander P (2015) Magnitude and farm-economic value of grain legume pre-crop benefits in Europe: A review. Field Crop Res 175: 64-79.
- Fox RH, Myers RJK, Vallis I (1990) The nitrogen mineralization rate of legume residues in soil as influenced by their polyphenol, lignin, and nitrogen contents. Plant Soil 129: 251-259.
- Kumar K, Goh KM (2003) Nitrogen release from crop residues and organic amendments as affected by biochemical composition. Commun Soil Sci Plant Anal 34: 2441-2460.
- Justes E, Mary B, Nicolardot B (2009) Quantifying and modelling C and N mineralization kinetics of catch crop residues in soil: parameterization of the residue decomposition module of STICS model for mature and non mature residues. Plant Soil 325: 171-185.
- Abiven S, Recous S, Reyes V, Oliver R (2005) Mineralisation of C and N from root, stem and leaf residues in soil and role of their biochemical quality. Biol Fertil Soils 42: 119-128.
- Luna-Orea P, Wagger MG, Gumpertz ML (1996) Decomposition and nutrient release dynamics of two tropical legume cover crops. Agron J 88: 758-764.
- Nicolardot B, Recous S, Mary B (2001) Simulation of C and N mineralisation during crop residue decomposition: a simple dynamic model based on the C: N ratio of the residues. Plant Soil 228: 83-103.
- Redin M, Guénon R, Recous S, Schmatz R, De Freitas LL, et al. (2014) Carbon mineralization in soil of roots from twenty crop species, as affected by their chemical composition and botanical family. Plant Soil 378: 205-214.
- Peoples MB, Bowman AM, Gault RR, Herridge DF, McCallum MH, et al (2001) Factors regulating the contributions of fixed nitrogen by pasture and crop legumes to different farming systems of eastern Australia. Plant Soil 228: 29-41.
- Walley FL, Clayton GW, Miller PR, Carr PM, Lafond GP (2007) Nitrogen economy of pulse crop production in the Northern Great Plains. Agron J 99: 1710-1718.
- De Notaris C, Olesen JE, Sorensen P, Rasmussen J (2020) Input and mineralization of carbon and nitrogen in soil from legume-based cover crops. Nutr Cycl Agroecosys 116: 1-18.
- Frasier I, Noellemeyer E, Figuerola E, Erijman L, Permingeat H, et al. (2016) A High quality residues from cover crops favor changes in microbial community and enhance C and N sequestration. Global Ecol Conserv 6: 242-256.
- Izaurralde RC, McGill WB, Juma NG (1992) Nitrogen fixation efficiency, interspecies N transfer, and root growth in barley-field pea intercrop on a Black Chernozemic soil. Biol Fertil Soils 13: 11-16.
- McNeill AM, Fillery IRP (2008) Field measurement of lupin belowground nitrogen accumulation and recovery in the subsequent cereal-soil system in a semi-arid Mediterranean-type climate. Plant Soil 302: 297-316.
- Zhang D, Hui D, Luo Y, Zhou G (2008) Rates of litter decomposition in terrestrial ecosystems: global patterns and controlling factors. J Plant Ecol 1: 85-93.
- Jani AD, Grossman J, Smyth TJ, Hu S (2016) Winter legume cover-crop root decomposition and N release dynamics under disking and roller-crimping termination approaches. Renew Agr Food Syst 31: 214-229.
- Palm CA, Gachengo CN, Delve RJ, Cadisch G, Giller KE (2001) Organic inputs for soil fertility management in tropical agroecosystems: application of an organic resource database. Agric Ecosyst Environ 83: 27-42.
- Jani AD, Grossman JM, Smyth TJ, Hu S (2015) Influence of soil inorganic nitrogen and root diameter size on legume cover crop root decomposition and nitrogen release. Plant Soil 393: 57-68.
- Yang XM, Drury CF, Reynolds WD, Phillips LA (2020) Nitrogen release from shoots and roots of crimson clover hairy vetch and red clover. Can J Soil Sci 100: 179–188.
- Guinet M, Nicolardot B, Voisin AS (2020) Nitrogen benefits of ten legume pre-crops for wheat assessed by field measurements and modelling. Eur J Agron 120: 126151.
- Afnor (2013) Norme NF V18-122 Aliments des animaux - Détermination séquentielle des constituants pariétaux - Méthode par traitement aux détergents neutre et acide et à l'acide sulfurique. Afnor éditions Paris France.
- Van Soest PJ (1963) Use of detergents in the analysis of fibrous feeds. 2. A rapid method for the determination of fiber and lignin. J Ass Off Agric Chem 46: 829-835.
- Trinsoutrot I, Recous S, Bentz B, Lineres M, Cheneby D, et al. (2000) Biochemical quality of crop residues and carbon and nitrogen mineralization kinetics under non-limiting nitrogen conditions. Soil Sci Soc Am J 64: 918-926.
- R Core Team (2020) A Language and Environment for Statistical Computing. R Foundation for Statistical Computing, Vienna.
- Braak, P SMILAUER, C. TER BRAAK, P Šmilauer, CJF ter Braak, et al. (2012) Canoco reference manual and user's guide: software for ordination, version 5.0. Microcomputer Power, Ithaca, USA, p. 496.
- Jensen LS, Salo T, Palmason F, Breland TA, Henriksen TM, Stenberg B, et al. (2005) Influence of biochemical quality on C and N mineralisation from a broad variety of plant materials in soil. Plant Soil 273: 307-326.
- Li F, Sørensen P, Li X, Olesen JE (2020) Carbon and nitrogen mineralization differ between incorporated shoots and roots of legume versus non-legume based cover crops. Plant Soil 446:243–257.
- Jensen ES (1997) Nitrogen immobilization and mineralization during initial decomposition of 15N-labelled pea and barley residues. Biol Fertil Soils 24: 39-44.
- De Notaris C, Olesen JE, Sørensen P, Rasmussen J (2020) Input and mineralization of carbon and nitrogen in soilfrom legume-based cover crops. Nutr Cycl Agroecosyst 116: 1–18.
- Vanlauwe B, Nwoke OC, Sanginga N, Merckx R (1996) Impact of residue quality on the C and N mineralization of leaf and root residues of three agroforestry species. Plant Soil 183: 221-231.
- Yang XM, Drury CF, Reynolds WD, Phillips LA (2020) Nitrogen release from shoots and roots of crimson clover,hairy vetch, and red clover. Can J Soil Sci 100: 179–188.
- Urquiaga S, Cadisch G, Alves BJR, Boddey RM, Giller KE (1998) Influence of decomposition of roots of tropical forage species on the availability of soil nitrogen. Soil Biol Biochem 30: 2099-2106.
- Sievers T, Cook RL (2018) Aboveground and root decomposition of cereal rye and hairy vetch cover crops. Soil Sci Soc Am J 82: 147-155.
- Rinkes ZL, Bertrand I, Amin BAZ, Grandy AS, Wickings K, et al. (2016) Nitrogen alters microbial enzyme dynamics but not lignin chemistry during maize decomposition. Biogeochemistry 128: 171-186.
- Couëdel A, Alletto L, Tribouillois H, Justes E (2018) Cover crop crucifer-legume mixtures provide effective nitrate catch crop and nitrogen green manure ecosystem services. Agric Ecosyst Environ 254: 50-59.
- Tribouillois H, Cohan JP, Justes E (2015) Cover crop mixtures including legume produce ecosystem services of nitrate capture and green manuring: assessment combining experimentation and modelling. Plant Soil 401: 347-364.
- Guinet M, Nicolardot B, Revellin C, Durey D, Carlsson G, et al. (2018) Comparative effect of inorganic N on plant growth and N2 fixation of ten legume crops: towards a better understanding of the differential response among species Plant Soil 432: 207–227.
- Recous S, Aita C, Mary B (1998) In situ changes in gross N transformations in bare soil after addition of straw. Soil Biol Biochem 31: 119-133.
- Recous S, Robin D, Darwis D, Mary B (1995) Soil inorganic N availability: effect on maize residue decomposition. Soil Biol Biochem 27: 1529-1538.
- Fruit L, Recous S, Richard G (1999) Plant Residue Decomposition: Effect of Soil Porosity and Particle Size. In: Berthelin J, Huang PM, Bollag JM, Andreux F, Effect of Mineral-Organic-Microorganism Interactions on Soil and Freshwater Environments. Springer, Boston, MA, pp 189-196.
- Jeuffroy MH, Biarnès V, Cohan JP, Corre-Hellou G, Gastal F, et al. (2015) Performances agronomiques et gestion des légumineuses dans les systèmes de productions végétales. In Scheneider A, Huyghe C, Les légumineuses pour des systèmes agricoles et alimentaires durables. Editions Quae, Versailles, France, pp. 139-223.
- Crews TE, Peoples MB (2005) Can the synchrony of nitrogen supply and crop demand be improved in legume and fertilizer-based agroecosystems? A review. Nutr Cycl Agroecosyst 72: 101-120.
-
Maé Guinet*, Anne-Sophie Voisin and Bernard Nicolardot. Potential C and N Mineralisation of Shoot and Root Residues from Ten Grain Legume Species As Related to their Biochemical Characteristics. World J Agri & Soil Sci. 8(4): 2023. WJASS.MS.ID.000691.
-
Soil incubation, Grain legumes, Roots, Shoots, Decomposition, C:N ratio, Biochemical characteristics
-
This work is licensed under a Creative Commons Attribution-NonCommercial 4.0 International License.