Review Article
Comparative Cognition: The Narrowing Gulf Between Humans and Other Animals
Thomas R Zentall*
Department of Psychology, University of Kentucky, Lexington, Kentucky, USA
Thomas R Zentall, Department of Psychology, University of Kentucky, Lexington, Kentucky, USA
Received Date: February 06, 2024; Published Date: March 01, 2024
Abstract
Evolutionary psychologists propose that the survival of a species depends on their ability to meet its needs in its environment. Although there are differences in those environments there are also similarities in those needs: finding food, water, shelter, a mate, and often caring for young. Comparative cognition examines the similarities and differences between humans and other animals. In the present review several of those similarities are examined. First, I describe four abilities assumed to be cognitive. That is, abilities assumed to require more than the associative learning thought to be characteristic of non-human animals. The abilities examined here are imitation, same-difference learning, equivalence formation, and directed forgetting. I also identify four behaviors thought to be governed by human experiences and culture: cognitive dissonance, the sunk cost fallacy, base-rate neglect, and unskilled gambling. The results of these lines of research with animals suggest that under appropriate conditions, nonhuman animals can show abilities thought to be uniquely human. The results of the research also suggest that several of the biases and suboptimal behavior shown by humans have their bases in general predispositions that appear under conditions that appear in our complex society as biased or even suboptimal or maladaptive behaviors. It is concluded that a careful examination of the similarities and difference between humans and other animals can inform us, not only of the mechanisms underlying those cognitive abilities, but also of the origins of human biases and suboptimal behavior.
Keywords:Comparative cognition; suboptimal behavior; biased behavior
Introduction
Evolutionary psychologists suggest that humans share certain similarities with other animals [1]. Many of our needs for survival and reproduction are quite similar. We all need food, water, shelter, to find a mate and often to care for our young. Consider human origins. Not that long ago we were hunter-gatherers who lived and behaved not that differently from our primate cousins. Given the relatively slow changes in our genes due to natural selection, most of those changes have been attributable to culture. Thus, it is tempting to ignore the genetic similarities of modern humans to our huntergatherer ancestors, a mere 10,000 years ago. But perhaps we should not. Traditionally, American learning theorists such as Thorndike [2], Hull [3], and Skinner [4] did not emphasize the differences in learning between humans and other animals. Even researchers who studied human verbal learning used list learning procedures (Ebbinghaus) [5] and paired associate learning (Postman & Keppel) [6], and based their theories on associative learning principles (Cofer) [7]. The advent of the cognitive revolution encouraged a model of human learning that allowed for greater agency (e.g., subjective organization of list learning, e.g., Johns) [8]. With the exception of a small number of researchers, such as Tolman [9] and others (e.g., Lashley & Wade) [10], to a great extent, research on learning with other species remained focused on how associations were established, lost, and recovered. Although slower to become part of the mainstream of American learning theory, research with other species on behavior, thought to be beyond the scope of associative relations, has become an accepted field of study (Wasserman & Zentall; Zentall & Wasserman) [11,12].
Research in the field of comparative cognition has been challenged by the difficulty in ensuring that an animal understands what it is that the experimenter is asking. Verbal instructions are generally given to human subjects with some assurance that the instructions have been understood. With nonverbal animals, the instructions must be part of the task and any failure to demonstrate a particular cognitive ability could be an indication of a “failure of instructions” (Zentall) [13]. Thus, an animal’s failure to demonstrate a particular cognitive behavior may represent a failure on the part of the experimenter to provide adequate instructions. Thus, in assessing the cognitive abilities of animals it is important to use procedures that bring out those abilities, while at the same time preventing the animal from learning the task associatively. Much recent research on cognition with animals has focused on distinguishing between associative learning, together with primary stimulus generalization (the generalization from training with one set of stimuli to transfer to physically similar stimuli) and learning that approaches the kind of cognitive behavior often showed by humans (e.g., forming categories, making inferences, and active memory processes). Interestingly, while animal researchers have been focusing on the study of comparative cognition, the study of human learning has returned to explore the extent to which humans learn like other animals. This area of research has been stimulated in part by the distinction researchers have made between implicit learning (learning without awareness; Seger) [14] and explicit learning (the intentional learning of information).
The purpose of this review is to identify several selective areas of research that have been studied in both humans and other animals, and by demonstrating similarities in those behaviors, reduce some of the apparent qualitative differences that appear to distinguish between them. By adopting this interspecies approach, the goal of this review is, on the one hand, to recognize some of the cognitive abilities of other animals. On the other hand, if certain human behaviors thought to be mediated by human cultural factors and unique human experience are also true of other animals, then general behavioral factors unrelated to human culture may be responsible. I will start with four behaviors thought to be evidence for cognitive, non-associative behaviors. The first behavior, imitation, is the copying of the behavior of a conspecific (a member of the same species). At one time it was proposed to represent the ability of the observer to “take the perspective of others” (Piaget) [15]. According to this view, if one understands what one has to do to obtain the result obtained by the other, one must mentally put oneself in the place of the other.
The second behavior, same/different concept learning, is based on the development of abstract concepts that require more than associative relations. It suggests that if one learns to respond to a stimulus based on its similarity to (or difference from) another stimulus, that similarity relation will then generalize to other stimuli, physically dissimilar from the training stimuli, but that bare the same relation to the training stimuli. The third cognitive behavior, equivalence learning, is a characteristic of human language learning, in which symbols associated with common outcomes (e.g., a word and the object that the word represents) may come to have similar meaning, such that new associations learned about one (e.g., the word), appear to automatically transfer to the other (i.e., the object itself). Although such equivalences are thought by some (Sidman) [16] to be based on the sequential associative principles, of identity, symmetry, and transitivity, those principles are not readily demonstrated individually in nonhuman animals (Urcuioli) [17].
The fourth cognitive behavior examined in this review is directed forgetting. Humans can actively control information that they know they will have to remember, because if they are directed to forget, that information is not remembered as well. We have found that under the right conditions, pigeons too can show memory deficits when they are given a signal that memory for previous information will not be required. In the second part of this review, I will present the results of four programs of research concerning behavior that is thought to result from human cultural influence but because they also can be found in other animals, they are likely to be based on simpler behavioral mechanisms. The first of these behaviors is a form of cognitive dissonance. Cognitive dissonance is the mental discomfort that comes from having thoughts, beliefs, or attitudes that are inconsistent with one’s behavior (Festinger) [18]. The mental discomfort is often resolved by trying to justify or rationalize a decision that one has made or an action one has taken to which the discomfort can be attributed (Festinger) [19]. The dissonance is thought to result from the cultural admonition to avoid being inconsistent (Aronson & Carlsmith) [20]. Cognitive dissonance would be difficult to study in animals because thoughts, beliefs, and attitudes are difficult to assess in other animals. However, justification of effort, a version of cognitive dissonance, is more amenable to study in a non-verbal organism. The justification of effort effect occurs when the value of one’s effort affects the value of the outcome that follows. That is, often one finds that people value rewards that follow greater effort over those that follow less effort (Aronson & Mills) [21]. Surprisingly, there is evidence that a similar effect can be found in animals (Clement et al.,) [22].
The second biased behavior that I discuss is base rate neglect. Base rate neglect is the tendency for people to erroneously judge the likelihood of an outcome by not sufficiently considering all the relevant information. For example, the stereotype of librarians is that they are shy, but salespeople are not. One may thus conclude that a particular shy person is more likely to be a librarian than a salesperson but as there are many more salespeople than there are librarians, the probability that a given shy person is a librarian is actually less than that they are a salesperson. As we have found, non-human animals appear to show a similar bias (Zentall & Clement) [23]. The third behavior thought to involve cultural norms is the sunk cost fallacy. The sunk cost fallacy, a suboptimal behavior, is the reluctance to abandon a strategy or course of action because one has invested heavily in it, even when it is clear that abandoning it would be a better strategy. A sunk cost, sometimes called a retrospective cost, refers to an investment already incurred that cannot be recovered. Objectively one should give up a course of action if it is failing, independent of the resources already sunk into it. This behavior has sometimes been attributed to the cultural norm to avoid being wasteful. A prior loss is somehow a waste, however, if it is a sunk cost, it is not recoverable and should not enter into the decision to continue with the investment. What does it mean if other animals also show this bias?
The fourth behavior thought to be culturally determined is
the suboptimal choice involved in unskilled gambling behavior.
People often engage in the kind of economic risk-taking behavior
in which on average the return is less than the investment (e.g., slot
machines, roulette, and especially lotteries). Biologists propose that
optimal foraging theory should ensure that animals are sensitive
to the outcomes of their choices, and they should learn to avoid
those behaviors that are suboptimal. Humans, on the other hand,
often report that they engage in games of chance (gambling) for the
enjoyment of the game, rather than for the monetary rewards. If
the difference in motivation is correct, other animals should avoid
making suboptimal choices. Yet, there is evidence that several
other species show instrumental choice behavior that is objectively
suboptimal (Clement et al.) [22]. I have selected these examples of
other animals behaving similarly to that of humans, as examples to
suggest that there are many underappreciated similarities. Much of
the research cited has been conducted with pigeons because
a. So much research has been conducted with pigeons,
perhaps because they are easy to work with and for practical
reasons (e.g., they have very good vision; hodos et al.) [24] and
b. If the behavior can be demonstrated with pigeons, a
species that is as evolutionarily distant from humans, it likely
can be found in other species, especially those more closely
related to humans.
The set of behaviors and species discussed is meant to be illustrative, not comprehensive, and there are more that could be proposed.
Human Behaviors Thought to be Cognitive
Parsimony recommends that if a simple associative account can account for the results of an experiment, it is not necessary to posit a cognitive explanation (Morgan) [25]. Of course, it does not rule out a cognitive explanation, it just makes a cognitive account unnecessary. Thus, in evaluating cognitive accounts it is important to rule out simple associative explanations.
Imitation
There are times when the search for cognition in non-human animals can help clarify the mechanisms underlying a behavior. Because young humans readily copy the behavior of others (Meltzoff) [26], the capacity to imitate has been of interest to developmental psychologists. According to Piaget [15] the basis for children’s copying is cognitive in nature. That is, logically, Piaget viewed copying as a form of perspective taking. To obtain the outcome that a model is working to achieve, one would imagine being in the place of the model. If that were the case, however, youn children would not likely be capable of imitation, as perspectiveg taking is thought to develop in children only when they are 6 to 7 years old (Dixon & Moore) [27]. Furthermore, if true, imitation would not likely develop at all in most other animals. Thus, in the study of animal copying behavior, great effort has been made to separate behavior thought to be cognitive, from behavior thought to be based on simpler underlying mechanisms. If one considers all of the contexts in which one organism copies the behavior of another, many examples of copying can be explained in simpler terms. I will first list some of these mechanisms and then describe them in more detail.
The first example is behavior that is already in the animals repertoire and is triggered by similar behavior in another animal. This is often referred to as contagious behavior. Second, the mere presence of another animal may affect the observer’s motivation, and an increase in motivation may increase the probability that that the observer will make the target response. Third, if the behavior of another animal draws attention to a location, for example where food may be obtained, it may result in a response that resembles the behavior of the other animal, but the behavioral resemblance may be coincidental. Fourth, there is also the possibility that a simple conditioning mechanism is involved. For example, if a demonstrator pigeon is seen pecking at a light for a reinforcer, not only may an observer pigeon’s attention be drawn to the light, but the pairing of the light with food may be sufficient to get the observer to peck the light as well – a combination of drawing attention to a stimulus or location and a form of Pavlovian conditioning. Fifth, the behavior demonstrated may show the observer how the environment works (e.g., the direction that a door moves to allow access).
Contagious behavior
There are some cases in which an animal’s natural behavior is triggered or released by the presence of another animal engaged in that behavior. Mobbing occurs when a group of small birds attack a larger bird, often a bird of prey, to drive it away (Curio, Ernst, & Vieth) [28]. Often a conspecific (bird of the same species) joins or copies other birds in attacking the larger bird. However, mobbing is a genetically predisposed behavior that is triggered by the sight of other birds engaged in that behavior. Another example of behavioral contagion is related to eating behavior. For example, if a chicken is presented with a large amount of food, it will eat until sated (until it stops eating). If another hungry chicken is introduced, however, when the new bird begins eating, the sated bird will begin eating again (Bayer) [29]. Thus, the sated bird will copy the eating behavior of the hungry bird. However, eating is a predisposed behavior that is well established in each birds repertoire, so it would not qualify as imitative behavior.
Motivational effect
Zajonc [30] proposed that the mere presence of a conspecific will increase the arousal of an animal, and an increase in arousal may affect the likelihood of making a response that just happens to be similar to the response being made by the conspecific. For example, John, Chesler, Bartlett, and Victor (1968) found that cats that had observed a demonstrator being trained to jump over a hurdle to avoid foot shock learned the hurdle-jumping response faster than controls that did not observe the demonstrators. It may be, however, that being in the presence of a cat being shocked is sufficient to increase the observers’ fear (motivation) associated with the conditioning context. Under such conditions, the increase in motivation alone may account for the facilitated acquisition.
Perceptual facilitation
The presence of a conspecific may also draw attention to the conspecific’s location or to a stimulus with which the conspecific is interacting. Imagine that a demonstrator pigeon is pecking at a red light associated with food, and not pecking at a green light associated with the absence of food (Edwards, Hogan & Zentall) [31]. Furthermore, one finds that the observer learns to peck the red light and avoid pecking the green light faster than a different observer learns to peck the green light and avoid pecking the red light. It could be that attention drawn to the red light by seeing the demonstrator pecking it made it easier to learn to peck the red light, than learning to peck the green light.
Observational conditioning
In the example of perceptual facilitation just mentioned (Edwards et al) [31] attraction to the stimulus to which the demonstrator is pecking may also result in an association between that stimulus and the reinforcer that follows, a form of simple associative learning. It is well known that for pigeons, pairing a light with food will result in pecking the light (Brown & Jenkins) [32]. Consistent with the observational conditioning hypothesis, merely pairing a light with inaccessible food has been found to increase the likelihood that pigeons will peck at the light (Zentall & Hogan) [33]. An good example of observational conditioning is in the acquisition of fear of snakes by laboratory-reared monkeys exposed to a wild-born conspecific in the presence of a snake (Mineka & Cook) [34]. Although laboratory-reared monkeys are not naturally fearful of snakes, it appears that the fearful conspecific serves as an unconditioned stimulus and the snake as the conditioned stimulus. Importantly, exposure to a fearful conspecific or to a snake alone is insufficient to produce fear of snakes by the observer.
Goal emulation
When observation of a demonstrator allows an animal to learn how the environment functions, it may be sufficient to observe the action itself in the absence of the conspecific, for learning to occur. For example, if an animal observes a screen move to the left to allow access to food, when the animal is given access to the screen, it may be more likely to move the screen in the same direction, even in the absence of the conspecific demonstration (Campbell, Heyes, & Goldsmith; Klein & Zentall) [35,36]. Goal emulation may involve a sophisticated form of cognitive learning in its own right, but it would not be considered imitation. Although emulation typically takes place in a social context, it may not be considered social learning if it does not require learning the actions of a demonstrator.
True Imitation
To qualify as true imitation, one must rule out control by less cognitive or nonsocial mechanisms. A procedure that potentially satisfies this criterion is one in which a response can be made in two different ways, both of which have the same effect on the environment. This procedure is often referred to as the two-action procedure. For example, a bird may depress a treadle with either its beak or its foot. If observers are exposed to a demonstrator engaged in one of those behaviors, will the observer, when given the opportunity, make the response in the same way that it saw the response being made. If there is a positive correlation between the action of the demonstrator and the observer, one can say that the animal has imitated. Zentall, Sutton, and Sherburne [37] have found evidence for response copying of this kind in pigeons, as have Akins and Zentall [38] in Japanese quail. A method related to the twoaction procedure is the bi-direction control procedure (with the appropriate controls) in which the demonstrator pushes a screen either to the right or the left and the observer is found to push it in the same direction. Using this procedure, Heyes and Dawson [39] found that rats copied the behavior observed, as did Klein and Zentall [36] with pigeons, and Miller, Rayburn-Reeves, and Zentall [40] with dogs. With the bi-direction control procedure, it is important to control for goal emulation by asking if observation of the same movement in the absence of a conspecific would result in copying the movement to a similar degree. For a thorough analysis of the various procedures that have been used to study copying behavior in animals see Whiten and Ham [41], Zentall [42], Whiten et al. [43], Huber et al. [44], and Hoppitt and Laland [45].
The study of imitation in animals raises unanswered questions about the nature of the mechanisms involved, especially for imitation in which the observer cannot see itself making the observed response (e.g., for birds, stepping on a treadle) or if what the observer sees does not look similar to what it sees the demonstrator doing (e.g., for birds, pecking at a treadle). It seems unlikely that Piaget’s [15] hypothesis that imitation involves the observer taking the perspective of the demonstrator because, as noted earlier, even young children do not pass tests of perspective taking (Frye) [46]. Meltzoff [26] proposed a potential mechanism. He suggested that there is a form of cross modal matching that takes place automatically in the brain that can allow for an interaction between sight and touch or proprioception. A better understanding of the neural connections in the brain may be helpful in understanding the mechanism involved in this kind of copying behavior.
The Same/Different Concept
Children learn the same/different concept at an early age. Evidence that the concept has been learned is that once learned, it can be applied to any pair of objects, independent of the similarity of the training objects to the testing objects. The same/different concept is perhaps the most studied example of concept learning in animals. In the simplest version of a same/different concept experiment pigeons are trained on a task in which an instructional or sample stimulus indicates which of two comparison stimuli is correct. If a pigeon learns that the correct comparison stimulus is always, for example, the same color as the sample stimulus, one can ask if this training results in the formation of a sameness concept. This can be tested by transferring the pigeons to novel stimuli (Zentall & Hogan) [47]. If the transfer stimuli are sufficiently different from the training stimuli, positive transfer can be taken as evidence that something other than the sample-comparison associations were learned during training. That is, the relation between the sample stimuli and the correct comparison stimuli have been learned.
Zentall and Hogan [47] used such a design to study same/ difference learning. Half of the pigeons were trained to choose the stimulus that matched the sample, while the other half were trained to choose the stimulus that mismatched the sample (Figure 1). They trained the pigeons with colored stimuli (Exp. 1) or with different brightness values (Exp. 2) and transferred them to colors. In both experiments, half of each group was transferred to the same task (matching to matching or mismatching to mismatching) while the other half was transferred to the other task (matching to mismatching or mismatching to matching) evidence of positive transfer was found for the pigeons for which the task was the same, relative to pigeons for which the task was different. To rule out the possibility that primary stimulus generalization might have played a role in the transfer effects found, Zentall and Hogan [47] repeated the experiment with training stimuli (shapes) that were orthogonal to the transfer stimuli (colors) and found significant savings for the positive transferred pigeons relative to the negatively transferred pigeons.
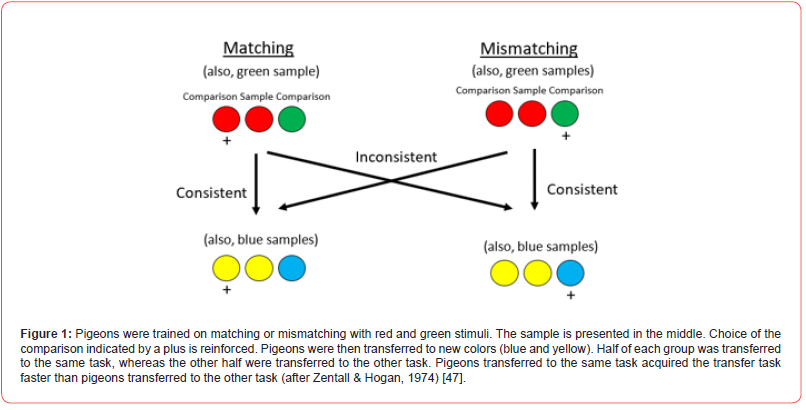
Larger transfer effects have been found with larger numbers of training stimuli consisting, for example, of pairs of same or different natural photographs (Katz, Wright & Bachevalier) [48], arrays of icons that were either all the same or all different (Young & Wasserman) [49], or arrays in which all of the images were the same or one of the images was different (Cook, Katz, & Cavoto) [50]. Another approach to the same-different concept is whether the concept can be expressed within a trial. For example, the sample consists of two stimuli that express the relation (either same or different). That is, the samples are either the same (e.g., two red squares) or different (e.g., a red square and a blue triangle) and the choice is between two pairs of comparison stimuli, all of which are different from the sample, but in one pair the stimuli are the same (e.g., two blue circles), whereas in the other pair they are different (e.g., a green plus sign and a yellow rectangle). Although neither pair of comparison stimuli match the sample, one pair represents the same relation, same or different. Thompson, Oden, and Boysen [51] trained chimpanzees on both standard (physical) matching and relational (conceptual) matching and tested them with novel stimuli.
Most of the primates showed rapid learning and good transfer to the novel stimuli. This kind of performance requires an understanding of a second-order relation, or a relation among relations. In all of these experiments, evidence for positive transfer was found when novel stimuli were presented. All of these studies provide converging evidence for the development of a same/ different concept by chimpanzees and pigeons. In all of these experiments, whatever specific associations were learned during training could not account for the transfer of training when novel stimuli were presented. An interesting extension of the samedifferent problem is reasoning by analogy (Gillan, Premack, & Woodruff) [52]. Given a pair of objects that bear a particular relation to each other (e.g., they look similar, but one is smaller than the other), chimpanzees are able to select the object that bears the same relation to a third object (Figure 2). The chimpanzee that was tested, Sarah, chose correctly significantly above chance when the critical relation was a difference in size, color, or marking (whether there was a dot on it or not). In the same series of experiments, they tested Sarah using a more abstract relation between two objects, an object and an instrument that acts on the object. For example, given a lock and a key and a third object, a can, Sarah was given a choice between a can opener and a brush. With this problem, the correct response was the can opener. However, on another trial she was given paper and pencil and the same can, with the same choice between a can opener and a brush. In this case, the correct response was the brush. The results indicated that Sarah could reason analogically with conceptual relations among complex stimuli.
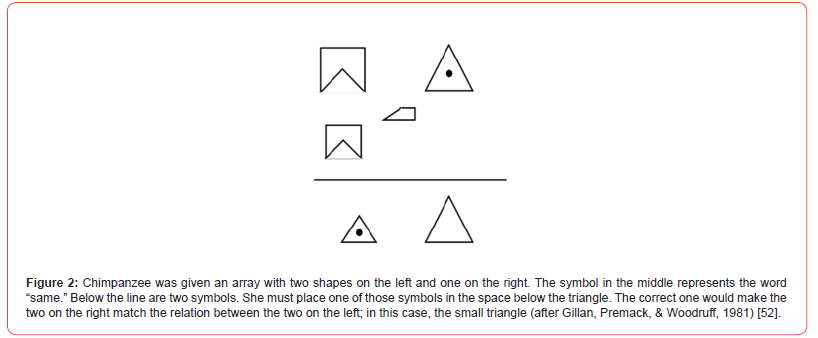
The Concept of Stimulus Equivalence
One of the important characteristics of human language is that objects and actions can be represented by symbols. The difference between an association and a symbol is, what is learned about symbols are representations that carry over to the objects or actions themselves (Womack) [53]. This feature allows for communication about objects in the absence of the objects themselves. For example, a child who is told that not all dogs are as friendly as her dog, can learn to be more careful around other dogs. To test for stimulus equivalence, pigeons are first trained on conditional discriminations (similar to matching but the relation between the sample and the correct comparison stimulus is arbitrary; neither comparison is the same as the sample). For example, one comparison stimulus (e.g., a circle) is correct in the presence of either a red light or a vertical line sample, whereas the other comparison stimulus (e.g., a dot) is correct in the presence of either a green light or a horizontal line sample (Figure 3). The question is do the two samples come to mean the same thing.
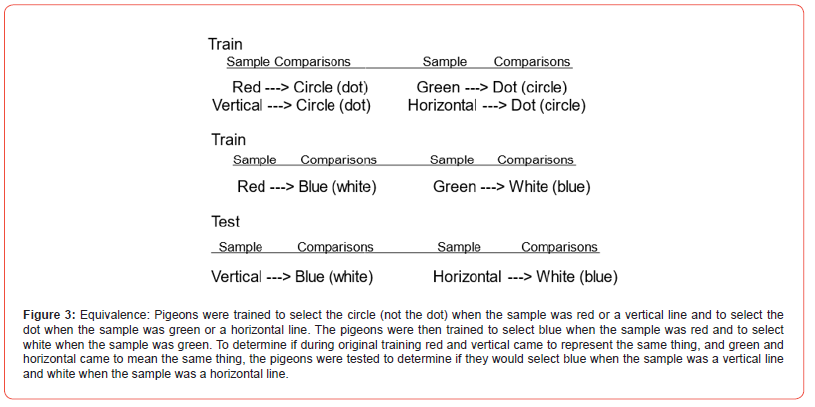
To mean the same thing implies that if something new is learned about one, it will automatically transfer to the other. To evaluate this form of equivalence, one can train the pigeons with another conditional discrimination that one of those samples, for example the red light, now indicates that a response to a blue light (but not a white light) will be reinforced. To test the pigeon for equivalence, the pigeon can be presented with the vertical line sample and the blue and white comparison stimuli (stimuli that they never saw together before). Choice of the blue light indicates that the red light and vertical lines have formed an equivalence set. That is, the new information that was learned about the red light has transferred to the vertical lines. Thus, this important cognitive characteristic of human language, not directly attributable to associative learning, can be acquired by pigeons (Sidman) [16]. Also germane to the question of animal language is research that has been done with chimpanzees. Several research projects have shown that chimpanzees can learn a large vocabulary (Gardner, Gardner, & Van Cantfort) [54], that they can respond appropriately to questions involving learned symbols (Premack) [55], and that they can make requests using learned symbols (Savage-Rumbaugh et al.) [56]. Whether the evidence from those projects qualifies as language, as humans use language, is still an open question but whatever critical differences remain, have not been found to be beyond the ability of these animals. One caveat in testing language ability in non-humans, one should be careful that the criterion one adopts (e.g., the absence of vocal language) does not similarly disqualify humans who have similar deficits (e.g., the hearing impaired who use American Sign Language). An interesting candidate proposed for language is the waggle dance of bees (von Frisch) [57]. Their dance on the vertical surface of the hive represents information about the direction, distance, and amount of food that they have experienced. However, their language is genetically predisposed. It is not learned and does not have any of the flexibility of human language.
Directed Forgetting
Cognitive processes are not always easy to define but they can be characterized as the search for evidence of active controlled processes. A cognition can be viewed as an active process if it intervenes between the information input and the response output (Roper & Zentall) [58]. For example, if humans are presented with a list of words, one at a time, and after each word they are told that it is a word that they will, or will not, be required to remember, they show a reduced ability to remember those words that they were told that they could forget (Bjork; Elme) [59,60]. This phenomenon is known as directed forgetting. Because the signal to remember or forget is presented following presentation of the word, it is assumed that the signal cannot affect the initial processing of the information but must affect its active maintenance (e.g., rehearsal) in memory. Its active maintenance presumably makes that information more available to be later recalled.
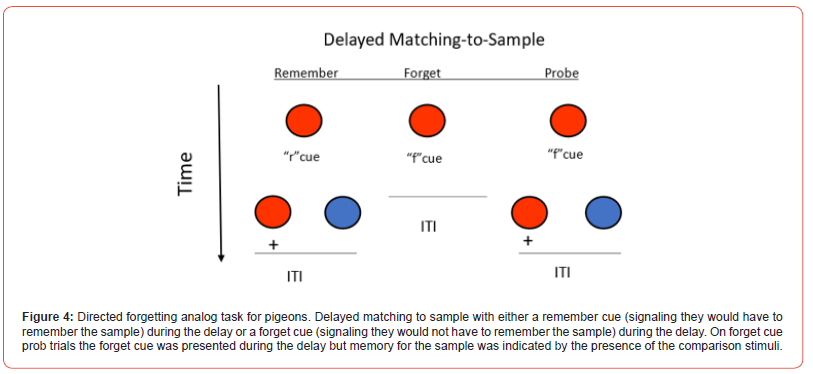
A version of directed forgetting has been studied in pigeons using a version of a conditional discrimination already described (Grant; Maki; Roberts, Mazmanian, & Kraemer) [61-63]. The challenge in studying directed forgetting in non-verbal animals is to find a way to incorporate the instructions to remember or forget, into the task (Zentall) [13]. To accomplish this, Maki [62] trained pigeons on a delayed matching task in which the sample stimulus was removed several seconds before the comparison stimuli appeared (delayed matching). Then, one of two signals was presented during the delay. One of these signals indicated that the comparison stimuli would be presented (a remember cue, e.g., vertical lines). The other signal indicated that the comparison stimuli would not be presented (a forget cue, e.g., horizontal lines). Directed forgetting was then assessed on probe trials on which, although the forget cue was presented, it was followed by the comparison stimuli. Much like with human subjects, the pigeons were “instructed to forget” the sample but then they are tested for their memory for the sample (see Figure 4 for the design). The finding that performance on such probe trials was poorer than on trials on which a remember cue was presented has been interpreted as evidence for directed forgetting (Maki et al.) [64]. A problem in interpreting the results of such an experiment is that although the forget cue signaled the absence of a test for sample memory, it also signaled that a reinforcer would not be obtained. Thus, the forget cue can be thought of as also having negative incentive value, a function different from that of the remember cue.
The difference in motivational value between the remember and forget cues can be corrected by substituting a source of reinforcement following the forget cue, but a one that does not require working memory for the sample. Various substitute events have been used but perhaps the best one involves replacing the comparison stimuli with a simple simultaneous discrimination involving stimuli different from either the conditional discrimination or the remember and forget cues (Grant & Barnet; Kendrick, Rilling, & Stonebraker; Maki, & Hegvik) [65-67]. That is, independent of the sample stimulus, one of the two comparison stimuli presented following the forget cue is always correct (Figure 5). As the forget cue should have motivational value comparable to the remember cue, any deficit found on forget cue probe trials (compared with whatever deficit in accuracy occurs on remember cue trials) should be attributable to an increase in sample forgetting. However, in most experiments that have used controls for the motivational differences between remember and forget cues, little evidence for a directed forgetting effect has been found by pigeons.
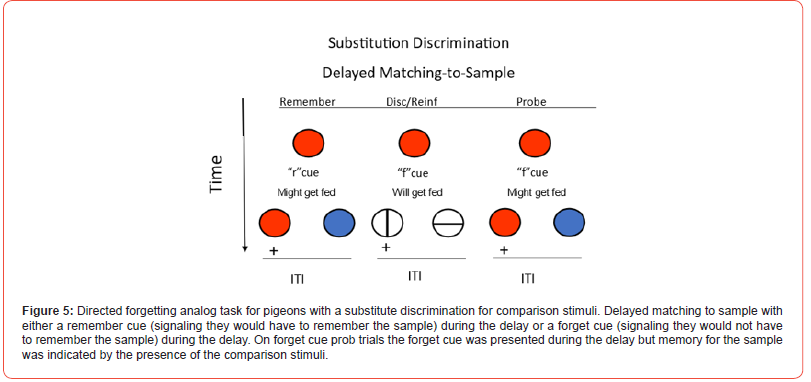
It may be, however, that other differences in procedure between directed forgetting in humans and pigeons might account for the difference in findings. Roper, Kaiser, and Zentall [68] reasoned that in related experiments, human subjects are often given items in the form of a serial list and after each item, an instruction to remember or to forget that item is presented. Because the list procedure involves both remember and forget items within the list, when the subjects encountered a forget cue, they could use the time before the next item was presented to rehearse prior items that they were instructed to remember. Thus, for humans, the forget cue allowed subjects to reallocate their active processing to remember items. With the pigeons, however, on a forget cue trial, there was only the just-presented sample that was eligible for forgetting. Thus, for the pigeons, there were no other stimuli for which to reallocate memory.
To evaluate this hypothesis, Roper et al. [68] used a design depicted in Figure 6 in which the forget cue instructed the pigeon to forget the sample, but it also instructed the pigeon to remember the forget cue itself. The forget cue could be one of two different stimuli, and which of the two comparison stimuli presented following the forget cue was correct depended on remembering the forget cue. This procedure still instructed the pigeon that it could forget the sample stimulus presented at the start of the trial, but it forced the pigeon to attend to the forget cue. Now, on forget-cue probe trials, after presentation of either of the forget cues, instead of the comparison stimuli relevant to the forget cues (as during training), the pigeon received the comparison stimuli relevant to the original sample, and the pigeon was tested for its ability to retrieve the color of the sample stimulus. Under these conditions, in spite of the appropriate controls for motivation and other non-memorial factors, pigeons showed a significant deficit in forget-cue probe trial accuracy (forget-cue trials when memory for the sample was requested) as compared with remember-cue trials. Thus, Roper et al. found evidence for directed forgetting. These results suggests that memory for pigeons is not an automatic process but, much like for humans, instructions to remember or forget can alter the way that the pigeon processes the stimulus following its offset.
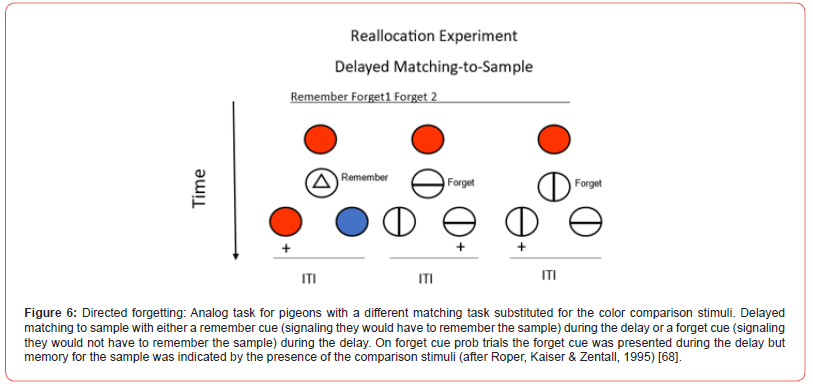
Human Biases that may not be Cognitively or Culturally Based
Several human behaviors that are thought to result from experience unique to humans have also been studied in other animals. When evidence for them has been found in other animals, it suggests that although those behaviors may be influenced by human culture they may have predisposed origins. In this part of the review, I will present the results of four programs of research on human biases thought to be culturally determined.
Cognitive dissonance
Cognitive dissonance is thought of as the mental discomfort that comes from behaving inconsistently with one’s beliefs (Festinger) [18]. Because it deals with beliefs cognitive dissonance would be a challenge to demonstrate in animals. One indirect approach to cognitive dissonance in animals was suggested by Egan, Santos, & Bloom [69]. They attempted to show cognitive dissonance in young children and monkeys by offered them a choice between two “equally preferred” items and then offering them a choice between the unchosen item and another “equally preferred” item. They found that the third item tended to be selected over the originally not chosen item. They argued that subjects had decided that the originally unchosen item must have been inferior to the originally chosen item (or they would not have rejected it). The dissonance produced by having initially rejected the item (although it was presumably equally preferred), encouraged them to reject it once again.
However, this effect depends entirely on the authors’ claim that all three items were of equal preference. But even small initial differential preferences likely would have resulted in a similar outcome, independent of cognitive dissonance. Imagine that there were small preferences among the items, in the order A, B, C (most to least preferred) and those preferences influenced choice. If initial choice was between A and B, A would have been selected and then when offered a choice between B and C, B would have been selected (the originally not selected item). In this case, the outcome would have been inconsistent with the authors’ conclusion. However, if the initial choice was between B and C, B would have been selected, and then when offered a choice between A and C, A would have been selected (the third item). Similarly, if the initial choice was between A and C, A would have been selected and then when offered a choice between B and C, B would have been selected (again, the third item). Thus, in the absence of cognitive dissonance, in two cases out of three, by chance, the third item would have been selected over the originally non-selected item. In fact, both the children and the monkeys selected the third item (unselected in the first choice) a bit less than 2/3 of the time.
A more tractable form of cognitive dissonance is justification of effort (Aronson & Mills) [21]. Justification of effort occurs when one gives greater value to rewards that are more difficult to obtain. However, typically, rewards that are more difficult to obtain are in fact worth more, especially in humans, if only because of the social rewards that may often follow them. For example, an A grade in a difficult course may be associated with greater social rewards than an A grade in an easier course. Even without those added rewards, one may value such a grade more because of past association with those social rewards. Such a social effect would not likely occur in non-human animals. That is, if an animal was required expend great effort for one outcome and less effort for another, comparable outcome, one would not expect the animal to show a preference for the outcome that followed greater effort. If the outcomes were truly comparable, either there should be no differential preference between the two outcomes, or one might expect that the outcome that followed the lesser effort would be preferred. Of course, the challenge is to make the outcomes the same.
Clement et al. [22] tested the effect of prior effort on outcome preference in animals by giving pigeons an experience with different effort, 1 peck or 20 pecks. In this experiment, the outcomes were identical amounts of mixed grain. In order to differentially signal the outcome, if the original effort required only 1 peck, it was always followed by, for example, a red light, and a peck to the red light was followed by the reward. If the original effort required 20 pecks, it was always followed by a green light, and a peck to the green light was followed by the same reward. To get the pigeons used to looking for the red or green light, the pigeons were required to discriminate the red light from a simultaneously presented yellow light and they were required to discriminate the green light from a simultaneously presented blue light, but choice of the yellow or blue light was always incorrect (Figure 7). Following extensive training with these contingencies, the pigeons were given a choice between the red light and the green light, the two positive colors from training. Any simple associative theory should predict indifference between the two lights because the peck requirement to the light, the delay to reinforcement, and the magnitude of reinforcement was the same to both lights. Alternatively, if the pigeons treated the trials as easy trials (1 peck) and harder trials (20 pecks), one might expect the pigeons to prefer the red light. Instead, the pigeons preferred the green light 2 to 1 – the light that they had to work harder to obtain.
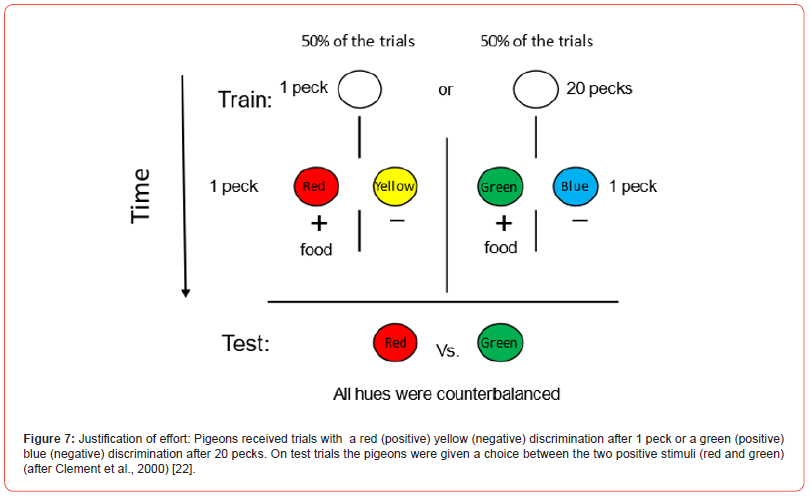
The results suggested that the value of the colored light was judged relative to the effort that was required to obtain it. Were this effect to occur in humans, it likely would be attributed to cognitive dissonance. But given that it occurred in pigeons, one would be more likely to describe it as a form of contrast. In this case, the contrast would be between the state of the pigeon just prior to the appearance of the colored light and when the colored light appeared. A simple model of such a contrast effect appears in Figure 8. Relative value appears on the ordinate, with time on the abscissa. Each trial is assumed to start at a relative value of zero and in this case, pecking is assumed to have a somewhat negative valence. The reinforcer (or in this case, the red or green signal for the reinforcer) is assumed to have a positive value and its relative value is determined by the difference between the state of the animal at the end of the effort and the appearance of the signal for reinforcement. That difference should be greater, the greater the effort (or time) required to obtain it.
This is a very simple model, and it does not necessarily specify what is responsible for the relative negative value of the event that precedes the signal for reinforcement. In fact, if the model is correct, any negative, or less preferred, event that precedes the signals for reinforcement should produce a similar effect. For example, organisms generally prefer short delays over longer delays to reinforcement. If the model is correct, pigeons should prefer a signal for reinforcement that is preceded by a longer delay. DiGian, Friedrich, and Zentall [70] tested this procedure and found that they do. Similarly, hungry pigeons prefer food over the absence of food. Again, if pecks to a light sometimes result in food and sometimes result in the absence of food, if the model is correct, they should prefer a signal for food that follows the absence of food, over a signal that follows the presence of food. Friedrich, Clement, and Zentall [71] tested this prediction and found that the pigeons did prefer signals that followed the absence of food over those that followed the presence of food.
One might wonder about the evolutionary value of such a bias for rewards that follow relatively aversive events. It might be proposed that food obtained after greater effort or delay, might give animals greater motivation to keep looking for food. If so, might locations of food become more preferred if they were harder to obtain? To test this hypothesis Friedrich & Zentall [70] required pigeons to peck many times for food presented at one feeder, and to peck very little for food presented at another feeder. When the pigeons were offered a choice between the two feeders, there was a shift in their preference towards the feeder that they had to work harder to obtain. To demonstrate that the model depicted in Figure 8 has further generality, Alessandri, Darcheville, and Zentall [72] tested the model with children and found that children too preferred a shape that followed 20 mouse clicks over a different shape that followed a single mouse click (both were followed by a brief video clip of a favorite cartoon). Similarly, Klein, Bhatt, and Zentall [73] found a comparable preference with adult subjects using the mouse click procedure. Furthermore, Alessandri, Darcheville, Delevoye-Turrell, & Zentall [74], using differential force required on a transducer as the differential prior event, found that adult humans preferred signals for reinforcement that followed greater force over less force. Interestingly, in both experiments with human adults, when they were asked about the relation between the greater effort and the signals for reinforcement, they rarely reported that they were aware of the correlation. The results of this line of research suggest that when such behavior occurs in humans, contrast between less preferred events and rewards may account for (or at least contribute to) various examples of what has been explained in terms of cognitive dissonance.
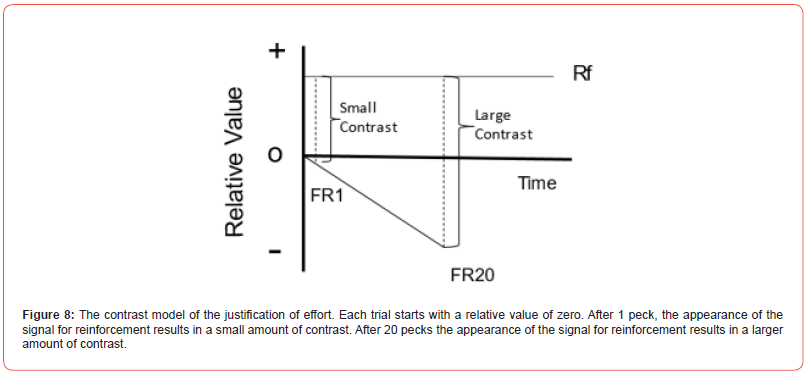
Can contrast of this kind be recruited to explain the more complex form of cognitive dissonance originally described by Festinger and Carlsmith [75]? In their experiment, subjects were given a lengthy, uninteresting task followed by a request to inform the next subject that the task was interesting. In return for this favor to the experimenter, the subject was given either $1 or $20. However, when asked to judge the task themselves, subjects that were given $1 judged the task as more interesting than those that were given $20. Consider the results of that experiment applied to a version of the model presented in Figure 9. Because the subjects were asked about their memory for the uninteresting task, it can be indicated by a negative value. Being offered $1 should have modest positive value and looking back at the uninteresting task (from $1 positive to somewhat negative) should be modestly negative. Being offered $20, however, should have strongly positive value and looking back at the uninteresting task (from having strongly positive value to having somewhat negative value) should be strongly negative. Hence, according to the contrast model, relatively speaking, judgements of the task for subjects given more money should be more negative than judgements of the task for subjects given less money. In general, almost any example of cognitive dissonance can be fit to the contrast model. That is not to say that the dissonance between beliefs and behavior does not contribute to contrast, only that underlying cognitive dissonance may be a simpler process, better described as contrast. The fact that one can see a justification of effort effect, a form of cognitive dissonance, in a pigeon, should suggest that even in humans, one should look for the most parsimonious account of behavior before necessarily assuming that the more cognitive account is correct.
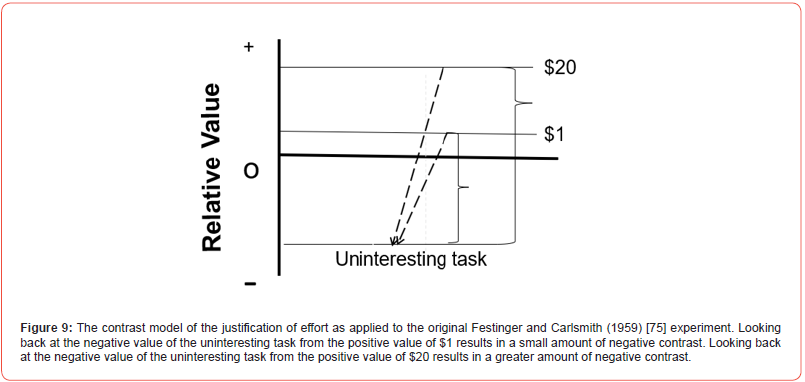
Base-rate neglect
Base rate neglect is the tendency to fail to sufficiently consider the general prevalence of a particular characteristic, in favor of individuating information (Tversky & Kahneman) [76]. An example of base rate neglect would be, given that certain symptoms are rarely associated with a disease (say, 10% of the time) but there is a test that is 80% accurate. Assuming that a patient tests positive for the disease, what is the probability that the patient actually has the disease? As it turns out, the positive test increases the probability that the patient has the disease but not as much as most people think it does because of the low probability that the symptoms are associated with the disease (Figure 10). The probability of testing positive and having the disease is .08 (the probability of having the disease, .10, times the accuracy of the test, .80) but the probability of testing positive and not having the disease is .18 (the probability of the test being inaccurate, .20, times the probability of not having the disease, .90). Now given a positive test, the probability that a patient actually has the disease is the probability of testing positive and having the disease (.08) divided by the total probability of testing positive (.08 + .18 = .26) or.08/.26 = .37. Thus, the test increased detecting someone with the disease from .08 to .37 but under these conditions, the test will detect someone with the disease only about a third of the time. Most people guess that the probability that someone has the disease is close to the accuracy of the test (.80). Even individuals who have an idea about the low base rate of having the disease guess that the probability that someone has the disease is above 50% (Tversky & Kahneman) [77].
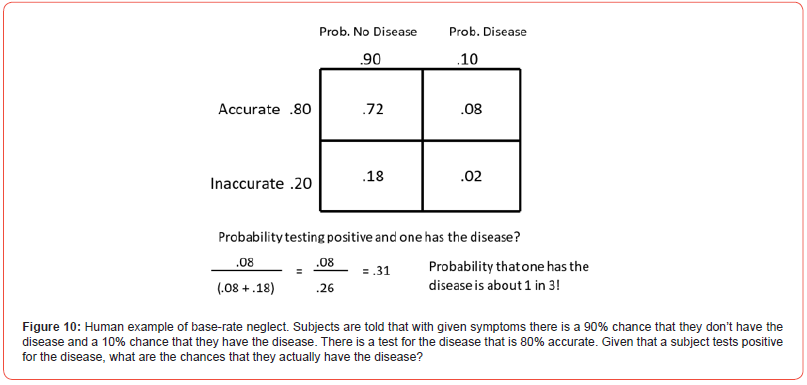
One of the problems with the results of such an experiment is people are known to have a difficult time understanding probabilities. One might hypothesize that if subjects had actual experience with the probabilities, they would do much better. But Goodie and Fantino [78] found that experience with the actual events does not eliminate the effect. With animals, experience is the only way to teach them about the probabilities, so one can ask if pigeons also show base rate neglect by not sufficiently taking base rates into account. To set up this test of base rate neglect, imagine a conditional discrimination in which a red sample indicates that the red comparison stimulus is correct, and a green sample indicates that the green comparison stimulus is correct, and each sample occurs equally often (see top line of Figure 11). Imagine, as well, a second conditional discrimination involving red and white samples associated with vertical and horizontal comparison stimuli, respectively (see bottom line of Figure 11), each occurring equally often and trials on both conditional discriminations are interspersed. Although the red sample appears twice as often as the green sample or the white sample, given the appearance of the red and green comparison stimuli or the vertical and horizontal comparison stimuli, there should not be a bias to choose either one.
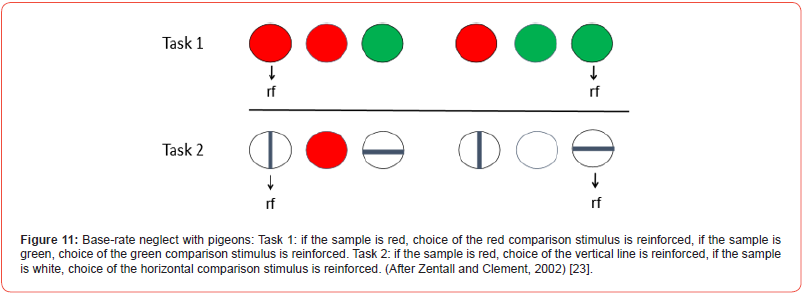
Pigeons can acquire these two conditional discriminations
quite readily, however, to produce errors or sample forgetting, a
delay is inserted between the offset of the sample and the onset of
the comparison stimuli. To be clear, if the pigeon cannot remember
the sample, the probability of being correct for choosing each of
the comparison stimuli should be the same (50%). Those are the
base rate probabilities (similar to the 10% probability of having the
disease in the absence of a test). In this case, the medical test is the
added information provided by memory for the sample stimulus.
With this procedure the red sample appears twice as often as either
the green or white sample but given the appearance of the red and
green comparison stimuli, or the vertical and horizontal lines, each
will be correct 50% of the time. Thus, in the absence of memory for
the sample, there should be no bias to choose either one. That is,
given that the probability of reinforcement associated with each of
the comparison stimuli was the same, one would expect that either
a. The pigeon would remember the sample and would have
been correct, or
b. The pigeon would have forgotten the sample and
comparison choice would have been based on the base rate
probability correct of the comparison stimuli (i.e., there should
not be a bias).
Zentall and Clement [23] found, however, that the pigeons showed a strong preference for the red comparison over the green comparison and the vertical line over the horizontal line comparison. Thus, in the absence of memory for the sample, although given that the probability of being correct for choosing either the red or green or the vertical or horizontal line comparison stimuli was the same, the pigeons showed a preference for the red stimulus and the vertical line. Apparently, they did so because the frequency of the red sample was greater than either the green or white sample. It should be noted, however, that half of those presentations of the red sample were irrelevant to the comparison stimuli that appeared on that trial. Thus, the pigeons choice was influenced by the irrelevant greater frequency of the red sample. Similar results were found by DiGian and Zentall [79] and by Zentall, Singer and Miller [80] using somewhat different experimental designs. There is another implication of this finding. It is that pigeons learn more than the association between the sample and the correct comparison stimulus because all four of those associations should have been equally strong. Instead, in the absence of sample memory, the pigeons appear to judge their comparison choice, based in part on the frequency of their experience with the sample frequencies. They do so in spite of the fact that half of those red sample frequencies should be irrelevant to the comparisons presented. The influence of sample frequency over the probability correct associated with the comparison stimuli suggests that pigeons misassign sample frequency similarly to the way humans misassign information about the accuracy of the medical test described earlier.
The sunk cost fallacy
That humans show a systematic bias when no obvious bias would be expected, it suggests that an unaccounted for mechanism is involved. However, as in the case of base rate neglect, a bias may not represent an actual loss of reward or delay to reinforcement. That is, there is no penalty for the preference for an outcome that one had to work harder to obtain (justification of effort) or for the base-rate neglect bias described for pigeons. When a bias results in choice of an outcome associated with an objectively smaller or suboptimal reward, all other things being equal, it is of particular interest because it would appear to be inconsistent with evolutionary principles of survival (and reproduction). Of course, the assumption that animals behave optimally assumes that the environment is similar to that in which the organism evolved. If the environment is not similar or if the natural contingencies are different, optimality would not necessarily be expected. However, if under similar conditions one finds that suboptimal behavior occurs in humans as well as in other animals, it would suggest that the mechanisms underlying the behavior may be similar and are not likely to be determined by culture or unique human experience. Furthermore, it suggests that humans are sometimes exposed to cultural conditions that result in suboptimal behavior.
The sunk cost fallacy is a cognitive bias that in humans makes one feel as if one should continue expending resources (money, time, or effort) into an endeavor because so much has already been invested in it. It is the feeling that to abandon the project would be to waste the resources that have already been invested. But logically, those resources have already been spent and the past investment should not enter into the decision to continue or not (Staw) [81]. A human example of the sunk cost fallacy is one may sit through a film that one does not like because to leave would be to waste one’s investment in the price of the ticket. In so doing, however, one is spending additional resources— additional time one could spend doing other things. Humans may escalate their investment in an enterprise, in an attempt to salvage a project, perhaps to prove to oneself or others that the previous commitment was rational. Alternatively, one may have misgivings about wasting resources, a disposition the economists call loss aversion (Novemsky & Kahneman) [82]. Arkes and Blumer [83] cite the sunk cost effect as a prime example of maladaptive behavior—the sort of mistake a nonhuman animal would not make because animals are more sensitive to reinforcement contingencies and are less likely to use abstract cultural rules to govern their behavior. Arkes and Ayton [84] concluded that it is only humans who demonstrate a true sunk cost fallacy. They contended that sunk cost fallacies occur when adult humans overgeneralize a cultural “don’t waste” rule because they are strongly loss-averse.
Navarro and Fantino [85] were the first to study the sunk cost effect in animals. They trained pigeons with a task that involved pecking for food. Pecking sometimes involved a high probability of getting food for a small number of pecks but it also could involve a lower probability of getting food with a larger number of pecks. At any time, however, by pecking a different light, they could start a new trial which would reinstate the original contingency (the high probability of getting food with a small number of pecks). Given these contingencies of reinforcement, to maximize the rate of reinforcement, the appropriate behavior would be to make the small number of pecks, and if no food was forthcoming, to restart the trial. Instead, the pigeons generally persisted in responding rather than starting a new trial, thereby requiring a larger number of pecks and thus, a longer delay for food. It is possible, however, that with this procedure the pigeons did not fully “understand” the contingencies.
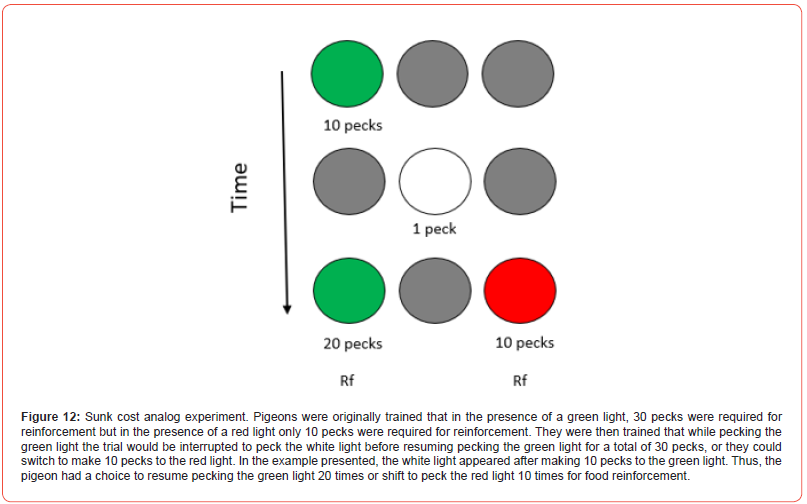
To ensure that the pigeons clearly recognized the alternatives (to either persist or to switch) Pattison, Zentall, and Watanabe [86] first trained pigeons that pecking a green light resulted in food after 30 pecks, whereas pecking a red light resulted in food after 10 pecks. When the pigeons were given a choice between the two colors, they always chose the red light. On critical trials, only the green light was turned on and after a variable number of pecks, the green light was turned off and a white light was turned on in the center (Figure 12). A peck to the white light turned on both the original green light on one side and the red light on the other side. On these trials, the pigeon could either peck the green light the number of times remaining, to equal a total of 30 pecks for food or peck the red light 10 times for food. The measure of interest was whether the pigeons would switch to the red light whenever they had already pecked the green light fewer than 20 times (because they would have required more than 10 pecks to the green light to get food) and return to the green light when more than 20 pecks had already been made to the green light (because they would have required fewer than 10 pecks to the green light to get food).
The results indicated that when they had already invested 10 pecks in the green light and were still required to make 20 pecks for food, most of the time the pigeons returned to finish the 30 pecks required to the green light. In general, the pigeons preferred to complete the 30 peck requirement to the green light, in spite of the fact that they could have gotten fed sooner and with less effort for switching to the red light. It was only when no pecks were required to the green light that the pigeons showed a strong preference for the red light (see also, Watanabe) [87]. The pigeons acted as if, switching to the red light would have meant that the pecks already made to the green light would have been wasted. Once again, the fact that pigeons show an effect similar to that of humans suggests that the basis for the suboptimal behavior in humans is not likely to be due to a social or cultural basis. Although human admonitions to “avoid waste” may contribute to the sunk cost effect in humans, the demonstration of similar effects in pigeons suggests that there are basic behavioral origins of this behavior.
Unskilled gambling
In unskilled gambling, there is typically a low probability of obtaining a high valued outcome with a relatively low cost of participation (e.g., lotteries, slot machines, roulette) but generally the return on investment is less than the investment. Although people who gamble often recognize the low probability of winning they frequently describe its entertainment value (Basham & Luik) [88]. According to evolutionary theory (Stephens & Krebs) [89], however, animals should have evolved to maximize reward value and should not be attracted to its entertainment value. Thus, given the fact that in most unskilled gambling the return on investment is generally less than the investment, in the long run, evolution should not favor this kind of gamble
Although it would be difficult to set up conditions for an animal similar to buying a lottery ticket, one could more easily give an animal a choice between one alternative, the choice of which results in a high reward but only a small percentage of the time, versus a second alternative, the choice of which always results in a smaller, but over time, greater reward. For example, if one gave a pigeon a choice of a risky alternative for which there was a 20% chance of getting a signal for 10 pellets of food (and an 80% chance of getting signal for no food) or a better alternative for which there was a 100% chance of getting a signal for 3 pellets of food (Figure 13), one might expect the pigeon to prefer the sure 3 pellets of food over the average of 2 pellets of food. Zentall and Stagner [90] found, however, that pigeons had a strong preference for the suboptimal 2-pellet alternative. More typically, in this line of research, the magnitude of reinforcement is held constant and only the probability of reinforcement is manipulated. Thus, for example, a pigeon is given a choice between a signal for reinforcement 20% of the time (or a signal for the absence of reinforcement 80% of the time) versus a 100% chance of getting a signal for 50% reinforcement (Figure 14). With that design, Stagner and Zentall [91] found a similar strong preference for the suboptimal 20% signaled reinforcer over the 50% unsignaled reinforcer.
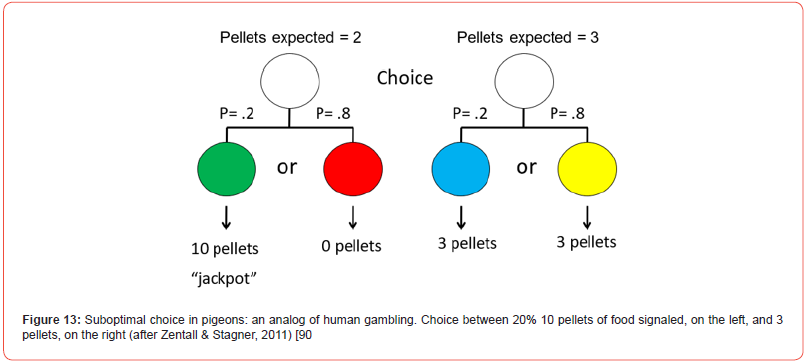
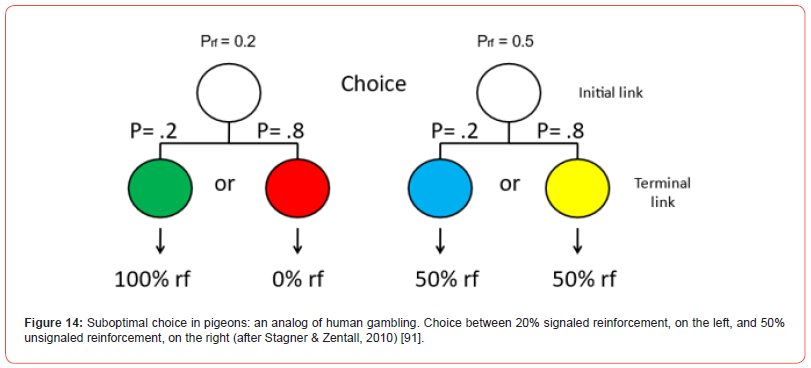
Although, in this case it might appear that it is the uncertainty associated with the optimal alternative (the signal for 50% reinforcement) that is responsible for the suboptimal choice of 20% reinforcement but as already noted, pigeons prefer a 20% chance of getting a signal for 10 pellets of food over a 100% chance of getting a signal for 3 pellets of food. Furthermore, surprisingly, there is also evidence that pigeons show a preference for a 50% chance of getting a signal for reinforcement (or a 50% chance of getting a signal for no reinforcer) over a 100% chance of getting a signal for reinforcement (Case & Zentall [92]; Figure 15). Although under these conditions pigeons do not always show a significant preference for the suboptimal alternative (see e.g., Spetch et al.) [93], any choice of 50% reinforcement when the alternative is 100% reinforcement would be considered suboptimal. Based on the results of several studies that reported a strong preference for 20% signaled over 50% unsignaled reinforcement, Smith and Zentall [94] proposed that the preference for the suboptimal alternative is determined by the value of the signal for reinforcement rather than the value of the initial choice. But, curiously, it also must be that the signal for the absence of a reinforcer has little or no negative value (McDevitt et al.) [95].
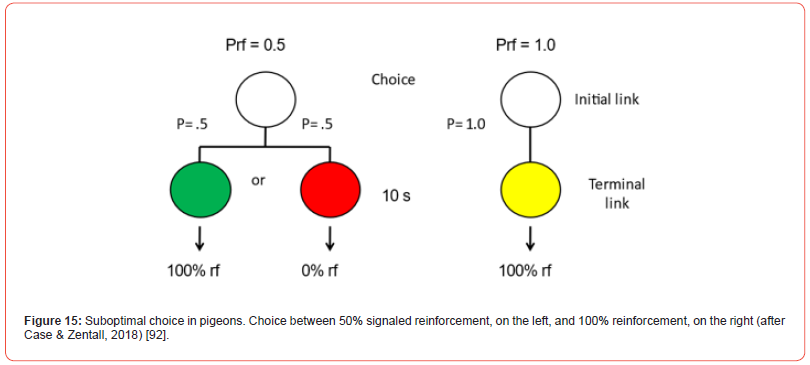
Laude, Stagner, and Zentall [96] investigated the value of the signal for the absence of reinforcement using a combined cue (or summation) procedure (Rescorla) [97] and found that as training proceeded, indeed, the signal for the absence of reinforcement had little inhibitory strength. That would also explain why pigeons prefer the alternative that provides a signal for 10 pellets of food over the alternative that provides a signal for 3 pellets of food. Although the signal for 100% reinforcement occurs only 20% of the time, it comes to function as a conditioned stimulus and its immediate appearance following the risky choice serves as a conditioned reinforcer. The signal for 50% reinforcement that follows choice of the optimal alternative, however, does not serve as effective a conditioned reinforcer because it does not reliably predict reinforcement. Thus, one can think of the reliable signals for reinforcement versus the unreliable signals for reinforcement in terms of probability discounting (Green, Myerson, & Vanderveldt) [98]. That is, more probable reinforcers are preferred over less probable reinforcers. If so, one would expect choice of the suboptimal alternative to be related to impulsivity because subjects that are impulsive generally discount outcomes more quickly. Laude et al. [99] tested this hypothesis and found a significant correlation between impulsivity, as measured by delay discounting (Evenden & Ryan) [100], and the development of a preference for the suboptimal alternative.
Although the degree of conditioned reinforcement associated with the signals for reinforcement can account for signals associated with differential probability of reinforcement, the finding that pigeons often prefer 50% signaled over 100% signaled reinforcement suggests that there must be an additional mechanism involved in the suboptimal choice because both alternatives provide a signal for 100% reinforcement (although the suboptimal alternative provides the signal for reinforcement only 50% of the time; Figure 14). Case and Zentall [92] suggest that the additional mechanism might be positive contrast. Paradoxically, for the suboptimal alternative, the contrast is between the expectation of reinforcement at the time of choice (50%) and the outcome associated with the signal for reinforcement (100%). For the optimal alternative, however, there is little contrast between the expectation of reinforcement at the time of choice (100%) and the outcome associated with signal for reinforcement (100%). Apparently, the positive contrast is sufficient to overcome the difference in the expected probability of reinforcement. A mechanism similar to positive contrast, signals for good news (SiGNs) was proposed by McDevit et al. [95].
In this section I have suggested that suboptimal choice research with pigeons is analogous to human unskilled gambling. Molet et al [101] tested this hypothesis more directly. Under conditions similar to those used in several of the pigeon experiments (20% signaled vs. 50% unsignaled reinforcement) university students who admitted to frequent gambling chose suboptimally more often than student nongamblers. Further support for the analogy of pigeon suboptimal choice and human gambling comes from demographic data that show between species parallels. For example, humans who earn less money, and thus, paradoxically, have more to lose, tend to gamble more than those who earn more money (Lyk-Jensen) [102]. Similarly, pigeons that have had less access to food tend to choose sub optimally more than pigeons that have had greater access to food (Laude, Pattison, & Zentall) [103]. As stated earlier, humans who gamble often state that they do so because it is entertaining. Is there an analog of this often proffered human motivation to gamble? Pattison, Laude, and Zentall [104] hypothesized that if gambling provided humans with a source of entertainment the same might be true of pigeons and if pigeons were provided with alternative sources of stimulation it might affect their propensity to choose sub optimally.
In their study, Pattison et al. [104] compared the suboptimal choice of pigeons that were typically housed (one to a cage, 23 hrs a day) with pigeons that were given 4 hrs a day in a large cage with three other pigeons. When both groups of pigeons experienced identical experimental procedures, the pigeons that were given a small amount of environmental enrichment chose the suboptimal alternative significantly less than the control pigeons. This finding suggests that problem gamblers may engage in this maladaptive activity in an effort to increase environmental stimulation and a potential treatment for problem gamblers may be to expose them to alternative forms of environmental stimulation (e.g., encourage them to join clubs and organizations that promote stimulating environments).
Conclusions
One can point to many distinctions between humans and other animals. Prime among them are that humans have elaborate language and culture. Although other animals show some evidence of having both (e.g., Pepperberg; Whiten) [105,106] the summative effects of those quantitative differences have resulted in large qualitative differences. Nevertheless, as I have tried to suggest here, there are many similarities between humans and other animals, both in their cognitive abilities and in their biases and suboptimal choice behavior. And those similarities exist even in animals that differ from each other as much as humans do from pigeons. The goal of this proposal is not merely to blur the lines between humans and other animals, but also to more consistently apply to humans the same level of analysis to distinguish complex cognitive behavior from simpler behavior that can be accounted for by simpler associative learning processes. When such an analysis is carried out, it may well be that the human behavior modeled by the animal research has both cognitive and associative components and it would be informative to understand how much each contributes to the behavior in question and how those two behavioral processes interact.
References
- Buss DM (2019) Evolutionary Psychology: The New Science of the Mind (6th ed.). Routledge. ISBN 978-1-138-08818-4.
- Thorndike EL (1911) Animal intelligence: Experimental studies. Macmillan Press, New York, United States.
- Hull C (1943) Principles of Behavior. Appleton-Century-Crofts, New York.
- Skinner BF (1938) The behavior of organisms: an experimental analysis. Appleton-Century, United States.
- Ebbinghaus H (1885/1962) Memory: A contribution to experimental psychology. Dover, New York.
- Postman LJ, Keppel G (1969) Verbal learning and memory: selected readings. Harmondsworth: Penguin, UK.
- Cofer CN (1961) Verbal Learning and Verbal Behavior. McGraw-Hill, New York.
- Johns EE (1985) Effects of list organization on item recognition. Journal of Experimental Psychology: Learning, Memory, and Cognition 11(3): 605–620.
- Tolman EC (1932) Purposive behavior in animals and men. Century/Random House UK.
- Lashley KS Wade M (1946) The Pavlovian theory of generalization. Psychological Review 53(2): 72–87.
- Wasserman EA, Zentall TR (Eds.). (2006) Comparative Cognition: Experimental Explorations of Animal Intelligence. Oxford University Press, New York.
- Zentall TR, Wasserman EA (Eds.) (2012) Oxford Handbook of Comparative Cognition. Oxford University Press, New York.
- Zentall TR (1997) Animal memory: The role of instructions. Learning and Motivation 28(2): 280-308.
- Seger CA (1994) Implicit learning. Psychological Bulletin 115(2): 163–196.
- Piaget J (1965) The Moral Judgment of the Child. Harcourt, Brace.
- Sidman M (1994) Equivalence relations and behavior: A research story. Authors Cooperative, Boston pp. 1-475.
- Urcuioli PJ (2008) Associative symmetry, antisymmetry, and a theory of pigeons' equivalence-class formation. Journal of the Experimental Analysis of Behavior 90(3): 257–282.
- Festinger L (1957) A theory of cognitive dissonance. Row, Peterson, Evanston, USA.
- Festinger L (1961) The psychological effects of insufficient rewards. American Psychologist 16(1): 1–11.
- Aronson E, Carlsmith JM (1963) Effect of severity of threat on the valuation of forbidden behavior. Journal of Abnormal and Social Psychology 66(6): 584–588.
- Aronson E, Mills J (1959) The effect of severity of initiation on liking for a group. Journal of Abnormal and Social Psychology 59(2): 177–181.
- Clement TS, Feltus J, Kaiser DH, Zentall TR (2000) Work ethic in pigeons: Reward value is directly related to the effort or time required to obtain the reward. Psychonomic Bulletin & Review 7(1): 100-106.
- Zentall TR, Clement TS (2002) Memory mechanisms in pigeons: Evidence of base-rate neglect. Journal of Experiment Psychology: Animal Behavior Processes 28(1): 111-115.
- Hodos W, Bessette BB, Macko KA, Weiss SRB (1985) Normative data for pigeon vision. Vision Research 25(10): 1525-1527.
- Morgan CL (1894) An Introduction to Comparative Psychology. Walter Scott, London.
- Meltzoff AN (1990) Towards a developmental cognitive science: The implications of cross-modal matching and imitation for the development of representation and memory in infancy. Annals of the New York Academy of Sciences 608(2): 1-37.
- Dixon JA, Moore CF (1990) The development of perspective taking: Understanding differences in information and weighting. Child Development 61(5): 1502-1513.
- Curio E, Ernst U, Vieth W (1978) Cultural transmission of enemy recognition: One function of mobbing. Science 202(4370): 899–901.
- Bayer E (1929) Beltrage zur Swelkomponententheone des Hungers. Zeitschrift fur Psychologae 122(1): 1–54.
- Zajonc RB (1965) Social facilitation. Science 149(3681): 269–274.
- Edwards CA, Hogan DE, Zentall TR (1980) Imitation of an appetitive discriminatory task by pigeons. Bird Behaviour 2(2): 87-91.
- Brown PL, Jenkins HM (1968) Auto-shaping of the pigeon's key-peck. Journal of the Experimental Analysis of Behavior 11(1): 1–8.
- Zentall TR, Hogan DE (1975) Key pecking in pigeons produced by pairing key light with inaccessible grain. Journal of the Experimental Analysis of Behavior 23(2): 199-206.
- Mineka S, Cook M (1988) Social learning and the acquisition of snake fear in monkeys. In TR Zentall, BG Galef Jr (Eds.), Social learning: Psychological and biological perspectives. Lawrence Erlbaum Associates pp. 51-73.
- Campbell FM, Heyes CM, Goldsmith AR (1999) Stimulus learning and response learning by observation in the European starling, in a two-object/two-action test. Animal Behaviour 58(1): 151–158.
- Klein ED, Zentall TR (2003) Imitation and affordance learning by pigeons (Columba livia). Journal of Comparative Psychology 117(4): 414-419.
- Zentall TR, Sutton JE, Sherburne LM (1996) True imitative learning in pigeons. Psychological Science 7(6): 343-346.
- Akins CK, Zentall TR (1996) Imitative learning in male Japanese quail (Coturnix japonica) involving the two-action method. Journal of Comparative Psychology 110(3): 316-320.
- Heyes CM, Dawson GR (1990) A demonstration of observational learning in rats using a bidirectional control. The Quarterly Journal of Experimental Psychology B: Comparative and Physiological Psychology 42(B-1): 59–71.
- Miller HC, Rayburn Reeves R, Zentall TR (2009) Imitation and emulation by dogs using the bidirectional control procedure. Behavioural Processes 80(2): 109-114.
- Whiten A, Ham R (1992) On the nature and evolution of imitation in the animal kingdom: Reappraisal of a century of research. Advances in the study of behavior 11(1): 239–283.
- Zentall TR (1996) An analysis of imitative learning in animals. In CM Heyes, BG Galef Jr (Eds.), Social learning and tradition in animals. Hillsdale, NJ: Erlbaum pp. 211-243.
- Whiten A, McGuigan N, Marshall Pescini S, Hopper LM (2009) Emulation, imitation, over-imitation and the scope of culture for child and chimpanzee. Philosophical Transactions of the Royal Society of London B Biological Sciences 364(1528): 2417-2428.
- Huber L, Range F, Voelkl B, Szucsich A, Virányi Z, et al. (2009) The evolution of imitation: What do the capacities of non-human animals tell us about the mechanisms of imitation? Philosophical Transactions of the Royal Society B 364(1528): 2299–2309.
- Hoppitt W, Laland KN (2008) Social processes affecting feeding and drinking in the domestic fowl. Animal Behaviour 76(5): 1529–1543.
- Frye D (1993) Causes and precursors of children’s theory of mind. In DF Hay, A Angold (Eds.), Precursors and causes of development and psychopathology. Wiley, Chichester, UK.
- Zentall TR, Hogan DE (1974) Abstract concept learning in the pigeon. Journal of Experimental Psychology 102(3): 393 398.
- Katz JS, Wright AA, Bachevalier J (2002) Mechanisms of same/different abstract-concept learning by rhesus monkeys (Macaca mulatta). Journal of Experimental Psychology: Animal Behavior Processes 28(4): 358-68.
- Young ME, Wasserman EA (2002) Detecting variety: what's so special about uniformity? Journal of Experimental Psychology: General 131(1): 131-43.
- Cook RG, Katz JS, Cavoto BR (1997) Pigeon same different concept learning with multiple stimulus classes. Journal of Experimental Psychology: Animal Behavior Processes 23(4): 417–433.
- Thompson RKR, Oden DL, Boysen ST (1997) Language-naive chimpanzees (Pan troglodytes) judge relations between relations in a conceptual matching-to-sample task. Journal of Experimental Psychology: Animal Behavior Processes 23(1): 31–43.
- Gillan DJ, Premack D, Woodruff G (1981) Reasoning in the chimpanzee: I. Analogical reasoning. Journal of Experimental Psychology: Animal Behavior Processes 7(1): 1–17.
- Womack M (2005) Symbols and Meaning: A Concise Introduction. Walnut Creek: AltaMira Press, Maryland, United States.
- Gardner RA, Gardner BT, Van Cantfort TE (Eds.) (1989) Teaching Sign Language to Chimpanzees. State University of New York Press, New York, United States.
- Premack D (1976) Language and Intelligence in Ape and Man: How much is the gap between human and animal intelligence narrowed by recent demonstrations of language in chimpanzees? American Scientist 64(6): 674–683.
- Savage Rumbaugh ES, Murphy J, Sevcik RA, Brakke KE, Williams SL, et al. (1993) Language Comprehension in Ape and Child. Monographs of the Society for Research in Child Development 58(3-4): 1–222.
- Frisch Kvon (1967) The dance language and orientation of bees. [Trans. Leigh E. Chadwick.] Harvard University Press, Cambridge, Massachusetts, United States.
- Roper KL, Zentall TR (1993) Directed forgetting in animals. Psychological Bulletin 113(3): 513-532.
- Bjork RA (1972) Theoretical implications of directed forgetting. In AW Melton, E Martin (Eds.), Coding processes in human memory New York: Winston & Wiley pp. 217-235.
- Elmes DG (1969) Role of prior recalls and storage load in short-term memory. Journal of Experimental Psychology 79(3): 468-472.
- Grant DS (1981) Stimulus control of information processing in pigeon short-term memory. Learning and Motivation 12(1): 19-39.
- Maki WS (1981) Directed forgetting in animals. In NE Spear, RR Miller (Eds.), Information processing in animals: Memory mechanisms Hillsdale, NJ: Erlbaum pp. 199-226.
- Roberts WA, Mazmanian DS, Kraemer PJ (1984) Directed forgetting in monkeys. Animal Learning & Behavior 12(1): 29-40.
- Maki WS, Gillund G, Hauge G, Siders WA (1977) Matching to sample after extinction of observing responses. Journal of Experimental Psychology: Animal Behavior Processes 3(3): 285-296.
- Grant DS, Barnet RC (1991) Irrelevance of sample stimuli and directed forgetting in pigeons. Journal of the Experimental Analysis of Behavior 55(1): 97-108.
- Kendrick DE, Rilling ME, Stonebraker TB (1981) Stimulus control of delayed matching in pigeons: Directed forgetting. Journal of the Experimental Analysis of Behavior 36(2): 241-251.
- Maki WS, Hegvik DK (1980) Directed forgetting in pigeons. Animal Learning and Behavior 8(1): 567-574.
- Roper KL, Kaiser DH, Zentall TR (1995) True directed forgetting in pigeons may occur only when alternative working memory is required on forget-cue trials. Animal Learning & Behavior 23(3): 280-285.
- Egan LC, Santos LR, Bloom P (2007) The origins of cognitive dissonance: evidence from children and monkeys. Psychological Science 18(11): 978-983.
- DiGian KA, Friedrich AM, Zentall TR (2004) Discriminative stimuli that follow a delay have added value for pigeons. Psychonomic Bulletin & Review 11(5): 889-895.
- Friedrich AM, Clement TS, Zentall TR (2005) Discriminative stimuli that follow the absence of reinforcement are preferred by pigeons over those that follow reinforcement. Learning & Behavior 33(3): 337-342.
- Alessandri J, Darcheville JC, Zentall TR (2008) Cognitive dissonance in children: Justification of effort or contrast? Psychonomic Bulletin & Review 15(3): 673-677.
- Klein ED, Bhatt RS, Zentall TR (2005) Contrast and the justification of effort. Psychonomic Bulletin & Review 12(2): 335-339.
- Alessandri J, Darcheville JC, DelevoyeTurrell Y, Zentall TR (2008) Preference for rewards that follow greater effort and greater delay. Learning Behavior 36(4): 352-358.
- Festinger L, Carlsmith JM (1959) Cognitive consequences of forced compliance Journal of Abnormal and Social Psychology 58(2): 203-211.
- Tversky A, Kahneman D (1982) Evidential impact of base-rates. In: Kahneman D, Slovic P, Tversky A, (eds). Judgment under uncertainty: Heuristics and biases. Cambridge University Press Cambridge pp. 153–160.
- Tversky A, Kahneman D (1974) Judgment under Uncertainty: Heuristics and Biases. Science 185(4157): 1124-1131.
- Goodie A, Fantino E (1999) Base rates versus sample accuracy: Competition for control in human matching to sample. Journal of the Experimental Analysis of Behavior 71(2): 155–169.
- DiGian KA, Zentall TR (2007) Matching-to-sample in pigeons: In the absence of sample memory, sample frequency is a better predictor of comparison choice than the probability of reinforcement for comparison choice. Learning & Behavior 35(4): 242-261.
- Zentall TR, Singer RA, Miller HC (2008) Matching-to-sample by pigeons: The dissociation of samples from reinforcement contingencies. Behavioural Processes 78(2): 185-190.
- Staw BM (1981) The escalation of commitment to a course of action. Academy of Management Review 6(4): 577–587.
- Novemsky N, Kahneman D (2005) The Boundaries of Loss Aversion. Journal of Marketing Research 42(2): 119–128.
- Arkes HR, Blumer C (1985) The psychology of sunk cost. Organizational Behavior and Human Decision Processes 35(1): 124–140.
- Arkes HR, Ayton P (1999) The sunk cost and Concorde effects: Are humans less rational than lower animals? Psychological Bulletin 125(5): 591-600.
- Navarro AD, Fantino E (2005) The sunk cost effect in pigeons and humans. Journal of the Experimental Analysis of Behavior 83(1): 1–13.
- Pattison KF, Zentall TR, Watanabe S (2012) Sunk cost: Pigeons (Columba livia), too, show bias to complete a task rather than shift to another. Journal of Comparative Psychology 126(1): 1-9.
- Watanabe S (2009) The Concorde fallacy in pigeons. In S Watanabe, AP Blaisdell, L Huber, A Young (Eds.), Rational animals, irrational humans. Keio University, Tokyo, Japan pp. 135–149.
- Basham P, Luik J (2011) The social benefits of gambling. Economic Affairs 31(1): 9-13.
- Stephens DW, Krebs JR (1986) Foraging Theory. Princeton University Press, Princeton.
- Zentall TR, Stagner JP (2011) Maladaptive choice behavior by pigeons: An animal analog of gambling (sub-optimal human decision-making behavior). Proceedings of the Royal Society B Biological Sciences 278(1709): 1203-1208.
- Stagner JP, Zentall TR (2010) Suboptimal choice behavior by pigeons. Psychonomic Bulletin & Review 17(3): 412-416.
- Case JP, Zentall TR (2018) Suboptimal choice in pigeons: Does the predictive value of the conditioned reinforcer alone determine choice? Behavioural Processes 157(1): 320-326.
- Spetch ML, Belke TW, Barnet RC, Dunn R, Pierce WD (1990) Suboptimal choice in a percentage-reinforcement procedure: effects of signal condition and terminal-link length. Journal of the Experimental Analysis of Behavior 53(2): 219-234.
- Smith AP, Zentall TR (2016) Suboptimal choice in pigeons: Choice is based primarily on the value of the conditioned reinforcer rather than overall reinforcement rate. Journal of Experimental Psychology: Animal Behavior Processes 42(2): 212-220.
- McDevitt MA, Dunn RM, Spetch ML, Ludvig EA (2016) When good news leads to bad choices. Journal of the Experimental Analysis of Behavior 105 (1): 23-40.
- Laude JR, Stagner JP, Zentall TR (2014) Suboptimal choice by pigeons may result from the diminishing effect of nonreinforcement. Journal of Experimental Psychology: Animal Behavior Processes 40(1): 12-21.
- Rescorla RA (1971) Summation and retardation tests of latent inhibition. Journal of Comparative and Physiological Psychology 75(1): 77–81.
- Green L, Myerson J, Vanderveldt A (2014) Delay and probability discounting. In FK McSweeney, ES Murphy (Eds.), The Wiley Blackwell handbook of operant and classical conditioning. Wiley Blackwell pp. 307–337.
- Laude JR, Beckmann JS, Daniels CW, Zentall TR (2014) Impulsivity affects gambling-like choice by pigeons. Journal of Experimental Psychology: Animal Behavior Processes 40(1): 2-11.
- Evenden JL, Ryan CN (1996) The pharmacology of impulsive behaviour in rats: The effects of drugs on response choice with varying delays of reinforcement. Psychopharmacology 128(2): 161–170.
- Molet M, Miller HC, Laude JR, Kirk C, Manning B, et al. (2012) Decision-making by humans as assessed by a choice task: Do humans, like pigeons, show suboptimal choice? Learning & Behavior 40(4): 439-447.
- Lyk Jensen SV (2010) New evidence from the grey area: Danish results for at-risk gambling. Journal of Gambling Studies 26(3): 455-67.
- Laude JR, Pattison KF, Zentall TR (2012) Hungry pigeons make suboptimal choices, less hungry pigeons do not. Psychonomic Bulletin & Review 19(5): 884–891.
- Pattison KF, Laude JR, Zentall TR (2013) Social enrichment affects suboptimal, risky, gambling-like choice by pigeons. Animal Cognition 16(3): 429-434.
- Pepperberg IM (1999) The Alex Studies. Harvard University Press, Cambridge, Massachusetts, United States.
- Whiten A (2021) The burgeoning reach of animal culture. Science 372(6537): eabe6514-eabe6520.
-
Thomas R Zentall*. Comparative Cognition: The Narrowing Gulf Between Humans and Other Animals. Sci J Biol & Life Sci. 3(3): 2024. SJBLS.MS.ID.000564.
-
Comparative cognition; suboptimal behavior; biased behavior
-
This work is licensed under a Creative Commons Attribution-NonCommercial 4.0 International License.