Research Article
Determination of Food Preference in Pterygoplichthys multiradiatus
Edgar J Lozada-Gómez* and Omar Pérez-Reyes
Department of Environmental Sciences, College of Natural Sciences, University of Puerto Rico, Rio Piedras Campus, San Juan 00925, Puerto Rico, USA
Edgar J Lozada-Gómez, Department of Environmental Sciences, College of Natural Sciences, University of Puerto Rico, Rio Piedras Campus, San Juan 00925, Puerto Rico, USA
Received Date:December 27, 2023; Published Date:January 19, 2024
Abstract
Plastic debris is widespread in aquatic ecosystems and can potentially affect aquatic organisms’ ability to feed, grow, reproduce, and survive. However, the breadth and depth of the potential effects of microplastics on aquatic organisms are poorly understood. In this study, we evaluated the effect of exposure to plastics particulate on plecos (Pterygoplichthys multiradiatus). A seven-day exposure treatment with plastic and organic matter particles (with equal weights of 5.0 g in different shapes and sizes), exposure had no significant effect on the ingestion of the different exposed particles. The number of plastics and organic matter found by stomach analysis was relatively low, with a range of one to seven particles remaining in the stomach of individual fish at the end of a one-week exposure period. This suggests that these fish are able to detect and avoid ingestion of these plastic particles and organic matter in this size range, regardless of their body size. This proposes that this species prefers feeding at lower concentrations of these influenced particles to the natural food particles found in their natural habitat. Future research should focus on determining whether plastics may have more subtle effects on aquatic organisms, such as influencing the ability to feed on these contaminants.
Keywords:Armored Catfish; Exposure; Fish; Organic Matter; Plastic; Pleco
Introduction
Plastic pollution in rivers is a growing global concern. According to studies [1,2], plastic pollution severely affects transportation on rivers, fishing tourism, and access to clean and freshwater, endangering the lives of people that depend on this resource. Similarly, plastic ingestion by freshwater organisms is becoming increasingly common as plastic particles become smaller (e.g., microplastics, less than 5 mm in diameter). Plastics do not readily biodegrade but can be broken down into smaller pieces by physical abrasion and ultraviolet light [2]. Organisms living in these aquatic environments can be affected by the disposal and accumulation of this plastic debris by becoming entangled in it and sometimes ingesting it, causing intestinal obstructions that increase mortality rates [1]. Ingested plastic particles can physically damage the gastrointestinal tract (GIT). For example, consuming plastic can cause physical damage to the GIT due to how plastics are compacted in the gut [2], because the indigestible particles fill the stomach and decrease the sensation of hunger leading to starvation (due to intestinal obstruction). In addition, ingesting plastic can also be detrimental to the health of a wide variety of aquatic organisms. Several studies have examined the environmental impacts of microplastics in freshwater ecosystems, such as ingestion by fish species [3, 4, 5]; invertebrates [6-8], and vertebrates [9]. Despite growing evidence that numerous fish taxa consume plastic particles, little is known about the potential health consequences for the organism that ingests them [2].
Fish diets vary significantly between and within species. This variation results from feeding strategy or inherent variations in prey size [10]. Some species have extremely specialized diets, suggesting that they do not consume plastic frequently in their natural habitats. For example, [3], found that at least 33% of the specimens of Rutilus rutilus had one microplastic particle in their GIT; most of the particles were fibers, fragments, and filaments. This suggests a selective feeding of these plastic particles over other types of plastics. In addition, with ontogenetic dietary changes, fish exhibit individual variations in feeding behavior and may differ in their preference to consume plastics [11]. Therefore, to understand the effects of plastic ingestion on fish populations, it is necessary to quantify the variation among individuals in plastic ingestion liking and the effects of direct plastic ingestion.
In this study, part of our objective was to determine if plastic ingestion by Pterygoplichthys multiradiatus depends on direct exposure to these wastes, or in order to determine if plastic particles and different exposed organic matter are part of the diet of the species. Similarly, by exposing them to different organic matter, we were able to learn more about the feeding preference of P. multiradiatus, since there are records of their diet, composed mainly of detritus, algae, and various plant matter [12], but the diet has never been evaluated quantitatively. We hypothesized that larger individuals of P. multiradiatus were more likely to ingest the maximum possible number of plastic particles and organic matter than smaller individuals, such as the ability to distinguish between food and non-food particles. This study will provide valuable new evidence about the factors influencing the selection ingestion of plastic particles in freshwater fish.
Materials and Methods
Fish collection and acclimatization
Twelve plecos were collected in Río Bayamón (18.3767° N, 66.1370° W) near the Bayamon Golf Course, Bayamón, Puerto Rico. Pterygoplichthys multiradiatus fish were collected using fishing nets since the pools were shallow, less than one meter deep. Fishing nets were placed at the end of the pool (downstream) in order to facilitate the fish collection. The collection started from the pool’s upper part (upstream) towards the lower part; the fish moved downstream directly to the net and were captured. Collections consisted of catching as many fish as possible for a time limit; 40 to 60 minutes per pass per pool. At the end of the time limit, the collected fish were identified, counted, and transported under constant aeration to the University of Puerto Rico Río Piedras campus laboratory. Before starting the acclimatization of the fish in the aquaculture tanks, they were divided into two groups depending on the Total Body Length (TBL) and the width of the Fork of the Mouth (FM): Group 1: TBL of 30.5 – 37.8 cm and a FM of 2.9 – 3.8 cm; and Group 2: with a TBL of 39.9 – 47.2 cm and a FM of 3.7 – 4.6 cm. A metric tape and a Dial caliper were used to measure the TBL and FM of each fish. Total Body Length was measured from the most forward point of the head, with the mouth closed, to the farthest tip of the tail, with the tail compressed or squeezed, while the fish is lying on its side; the Fork Length (FL) was measured from the left corner to the right lateral corner of the fish’s mouth.
For the acclimatization process, the fish were separated into twelve wire cages (60 cm length x 60 cm width x 26 cm height) and placed in the aquaculture tanks (243 cm length x 121 cm width x 121 cm height) for seven days. The cages were designed with these dimensions to provide sufficient space for the fish to move around. In summary, six cages were placed in the first, and the other six in the second (Figure 1). Both water tanks were kept aerated throughout the study, and water chemistry factors were recorded daily. After seven days of acclimation, the fish were exposed to the treatments.
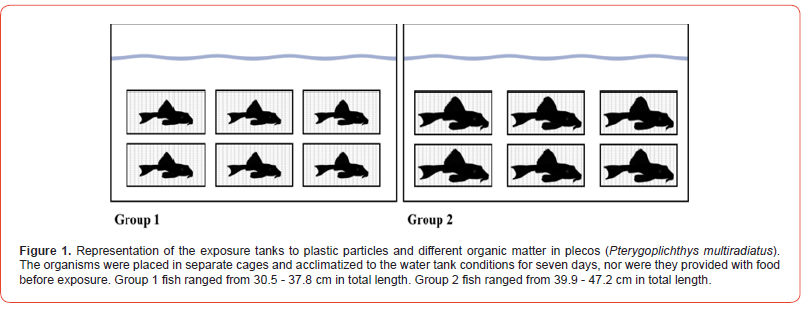
Food preference and plastics preparation
Polyethylene terephthalate (PET) and polystyrene (PS) were used in the experiment because they are among the most common types of plastics found in the environment [13]. For PET plastics pieces of plastic bottles, containers, drinking straws, and lids were cut until they reached the weight of, 5.0 g for each one. In addition, PET plastics were cut into irregular shapes since it was assumed that this would make it much easier for the fish to chew and ingest. A similar procedure was followed for the preparation of the PS plastic pieces.
Similar to the preparation of the plastics, we collected organic matter found in the river where the plecos were captured: pieces of bamboo, wood, leaf litter (e.g., Terminalia catappa and Ricinus communis), and land snail shells (Caracolus caracolla). All the organic material was placed in the oven at a temperature of 20 ˚C for 24-hours to remove the excess of water. After 24-hour in the oven the organic matter were cut until reached the weight of 5.0 g.
Acclimatization process of plastic particles and organic material
After drying and weight, the finishing of the plastics and organic matter were placed inside a wire cage (Food cage: 76 cm long x 60 cm wide x 13 cm high). Each food cage had bamboo (Bambusa vulgaris), lumber, leaf litter (Terminalia catappa and Ricinus communis), snail (Caracolus caracolla), PET, and PS. The food cage was placed inside the water tank for seven days before the experiment to encourage the growth of a microbial biofilm on the surface of each piece to be used and recreate the natural conditions observed in the stream [10].
Exposure experiment
After the fish groups were acclimatized to the conditions in the aquaculture tanks for seven days, the fish were exposed to the previously weighed and conditioned plastic and organic matter pieces (Figure 2). After seven days of exposure, the fish were removed from their cages and preserved. Stomach dissection was performed to remove the GIT from the fish to identify any remaining plastic or other retained organic matter with stomach analysis. If pieces of plastic or organic matter of these materials were found in any of the internal organs (esophagus, stomach, and intestine), the piece was preserved in 10 mL of 75% ethyl alcohol and the organ where the material was found. On the other hand, the remaining materials were removed from the fish cages, placed in trays, and dried in the oven for 24 hours (at a temperature of 20 ˚C). The dry weight of each material was again obtained after exposure. A difference in the weighing will allow the determination of which materials the fish preferred.
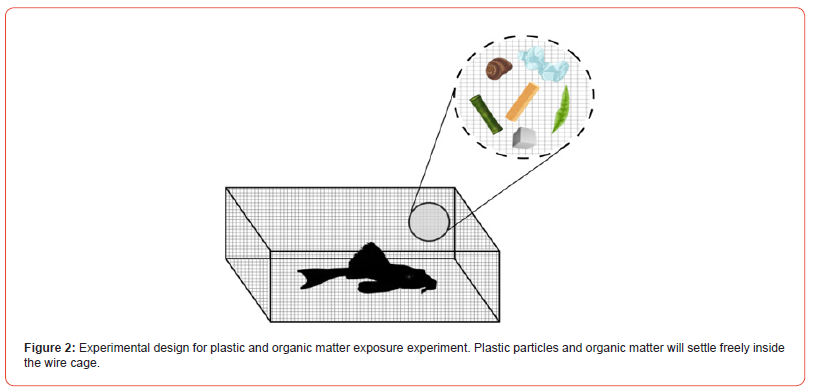
Statistical analysis
A one-way ANOVA analysis compared the water chemistry parameters between aquaculture tanks. A Pearson correlation test to compare the total fish body length (cm) and the amount of feed ingested by weight difference (in g) in fish of the same group and between groups, and a Chi-square test was used to determine food preference. In addition, a linear regression analysis was performed to observe the relationships between the mean values of Total Body Length (TBL) and the width of the Fork of the Mouth (FM) to determine if there is a relationship between fish size and the number of particles ingested.
Results
Water chemistry parameters
Table 1:Mean (±SE) water chemistry parameters in the exposure tanks. Measurements were taken from the acclimatization of the fish until the end of the exposure.
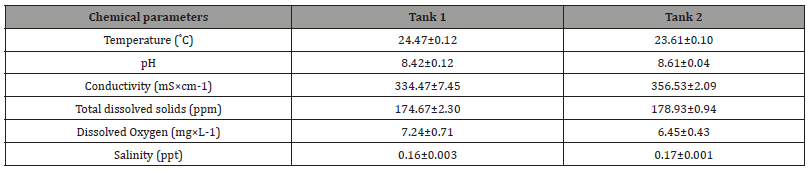
After one week of exposure, one-way ANOVA results for chemical parameters showed significant differences in temperature, pH, conductivity, total dissolved solids, dissolved oxygen and salinity between exposure water tanks (Table 1). Our results showed an increase in pH, conductivity and total dissolved solids in exposure Tank 2 water with high mean values 8.61 ± 0.04, 356.53 ± 2.09 ± S±cm-1, and 178.93 ± 0.94 ppm, respectively. While in tank 1 water chemistry recorded pH, conductivity and total dissolved solids with low mean values, 8.42 ± 0.12, 334.47 ± 7.45 ± S±cm-1, and 174.67 ± 2.30 ppm, respectively. The parameters of temperature and dissolved oxygen concentration in Tank 1 were significantly higher compared to Tank 2; while the salinity of Tank 2 showed a high mean value as opposed to Tank 1 with a low mean value (Table 1).
Exposure experiment
The proportions observed in the difference by weight of exposed particles showed that Group 1 fish appeared to be more likely to ingest particles of plastics (PS, polystyrene and PET, polyethylene terephthalate) and organic matter (leaf, wood, bamboo, shell) of all sizes and shapes than the larger Group 2 fish. Our results showed an increased intake of leaf, wood, and shell in Group 1 fish with high mean values 0.8 ± 0.09 g, 0.6 ± 0.14 g, and 1.0 ± 0.38 g, respectively (Figure 3). While in Group 2 fish, leaf, wood, and shell intake was recorded with low mean values 0.25 ± 0.4 g, 0.46 ± 0.09 g, and 0.70 ± 0.17 g, respectively (Figure 3). On exposure, PS and PET, plastic particles were significantly higher in Group 1 fish with high mean values than Group 2 fish (Figure 3). However, there was a large difference in the amount of particles ingested when analyzing stomach contents, with fish ingesting up to a maximum of 7 small fragments of organic matter (in a Group 2 classified fish) compared to a minimum of 1 small plastic fragment in a Group 1 fish. Fish were found to have a higher amount of these particles in their stomach when exposed to these particles of different shapes and sizes. Upon dissection, one fish from Group 1 and one fish from Group 2 were found to have plastic fragments (PS). However, two fish from Group 2 had higher retention of particulate organic matter fragments in the stomach (Figure 4).
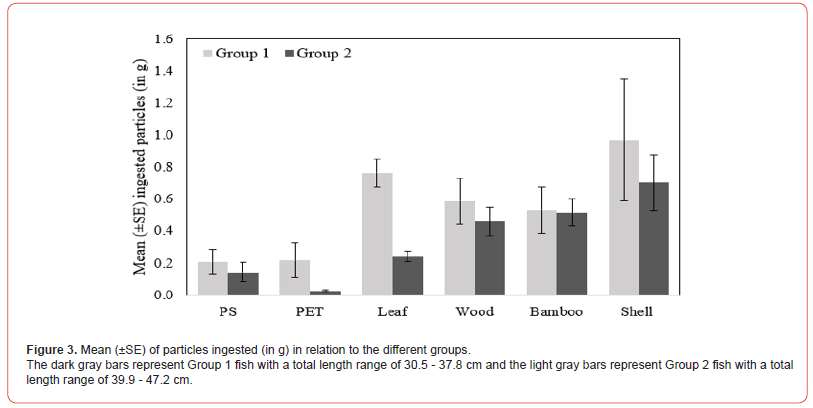
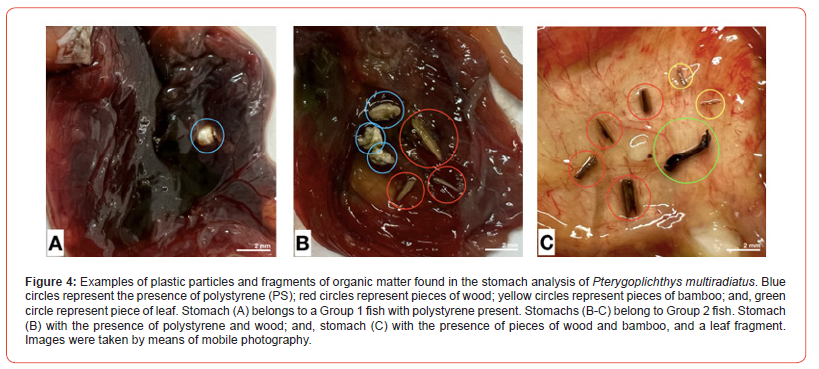
Pterygoplichthys multiradiatus. Blue circles represent the presence of polystyrene (PS); red circles represent pieces of wood; yellow circles represent pieces of bamboo; and, green circle represent piece of leaf. Stomach (A) belongs to a Group 1 fish with polystyrene present. Stomachs (B-C) belong to Group 2 fish. Stomach (B) with the presence of polystyrene and wood; and, stomach (C) with the presence of pieces of wood and bamboo, and a leaf fragment. Images were taken by means of mobile photography.
Food preference
The correlation between the total fish body length and the amount of food ingested by weight difference showed positive and negative variations in the group correlation coefficients. The concentrations of plastic particles and organic matter ingested by Group 1 showed a positive degree of correlation in PS (r = 0.0637, p <0.05), PET (r = 0.6489, p <0.05), Leaf (r = 0.01370, p <0.05), Wood (r = 0.6112, p <0.05), and Shell (r = 0.7862, p <0.05), respectively, in relation to the total fish body length (Figure 5a, b, c, d, f). In comparison, the negative correlation coefficient was only in Bamboo (r = -0.5913, p <0.05) (Figure 5e). Group 2 showed negative correlation coefficients in most of the exposed materials in PET (r = -0.3555, p <0.05), Leaf (r = -0.1587, p <0.05), Wood (r = -0.2648, p <0.05), Bamboo (r = -0.9145, p <0.05), and Shell (r = 0, p <0.05), respectively (Figure 6b, c, d, e, f). Only PS (r = 0.8733, p <0.05) revealed a grade with positive correlation coefficient with the total fish body length (Figure 6a). However, the correlation to compare the total fish body length and the amount of food ingested by weight difference between the groups revealed positive correlation coefficients in two variables, PS (r = 0.02898, p <0.05) and Shell (r = 0.03149, p <0.05), respectively (Figure 7a, f). The degrees of negative correlation was in four variables, PET, Leaf, Wood, and Bamboo (Figure 7b, c, d, e). There was no significant relationship between the total fish body length and the number of particles ingested at exposure (Figure 7). The Chi-square test for food preference between observed and expected showed no significant relationship between particles ingested and the two groups of fish separated by size (X2 = 1.875, df = 2, p = 0.1025).
A positive linear regression was observed between total body length (cm) and the width of the fork of the mouth (cm) for the species in the two groups. Therefore, the linear regression result showed significant evidence in relating the total fish length and mouth width for the species P. multiradiatus in the two groups (R2 = 0.884, F(1,10) = 76.2, p >0.05) (Figure 8).
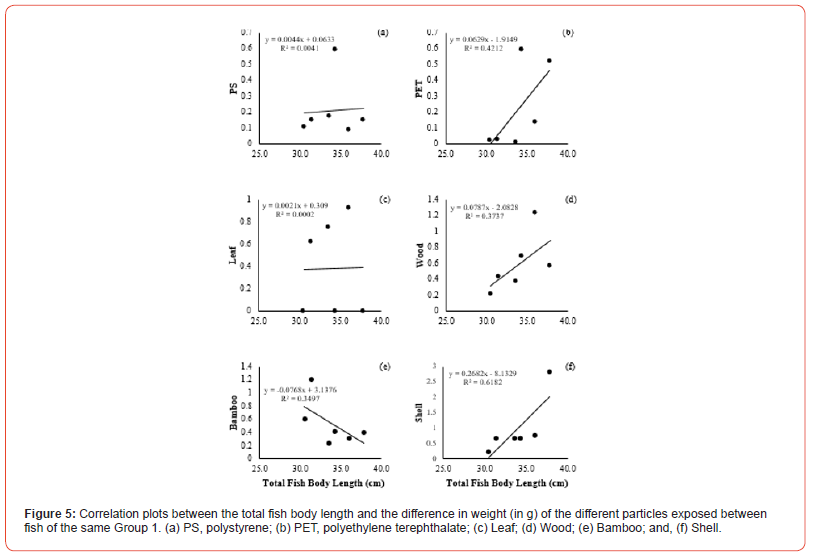
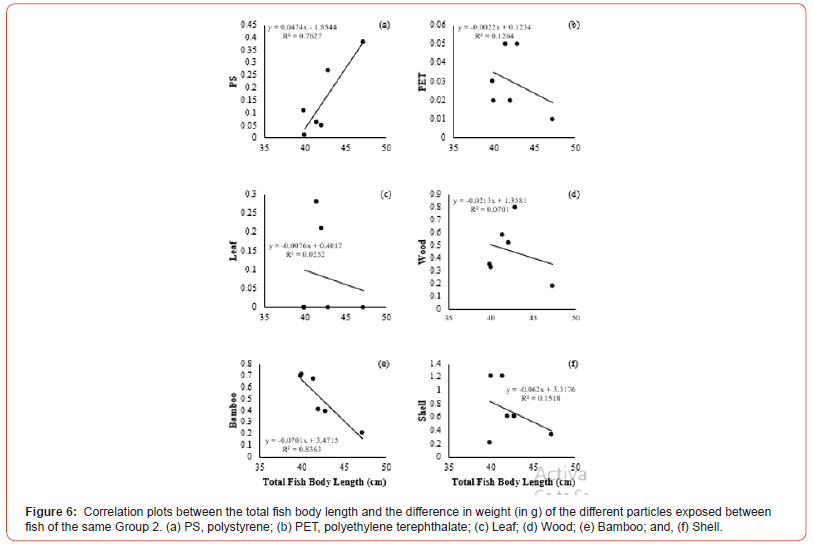
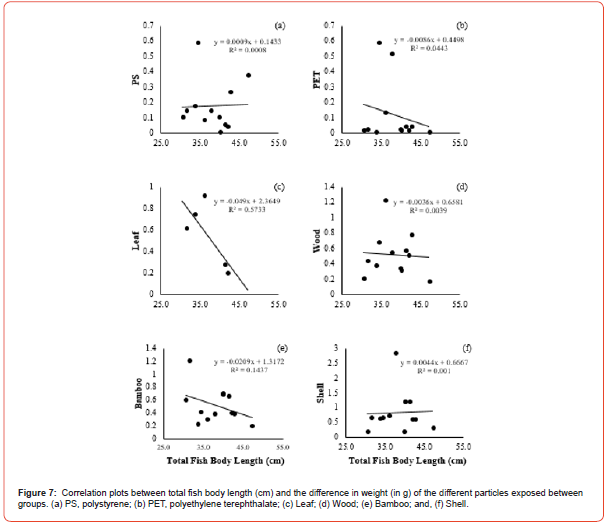
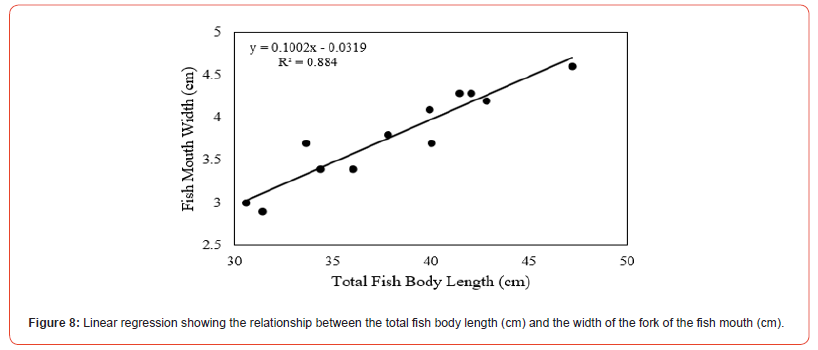
Discussion and Conclusions
In this study, we found that when plastic and/or organic matter particles were larger than the natural food, plecos (Pterygoplichthys multiradiatus) consumption is generally low and independent of these concentrations’ materials available in the stream. This suggests that this species prefers feeding at lower concentrations of these influenced particles to the natural food particles found in its natural habitat. Therefore, the amount of plastic found in the stomach analysis may have been influenced by the size of the plastic particles that were broken into smaller fragments by the movement of the fish within the cage. This is of concern because detritivores fish such as this species are more likely to consume plastics due to the breakdown and fragmentation into smaller and smaller pieces in the environment naturally caused and influenced by the type of movement behavior of these fish. Similarly, the low retention of plastic particles in the stomach suggests that plecos are able to detect and avoid ingestion of these large plastic particles consumed much less frequently than smaller plastics (e.g., microplastics). In addition, for all plecos exposed to particulate organic matter, only two were found to have small fragments in their stomach after one week of exposure, with Group 2 fish having the highest presence of these particles (Table 1, Figure 4). These results support the hypothesis that larger fish of the same species were more likely to ingest the maximum possible number of plastic particles and organic matter than smaller fish, such as the ability to distinguish between food and non-food particles. This demonstrates that particular ingestion of plastic and these organic matters by pleco can occur even at the low concentrations available in the environment, regardless of fish size and mouth fork size. The linear regression analysis showed no significant relationship between total fish body length and the width of the fork of the mouth (Figure 8), suggesting that the amount of ingestion of plecos is not directly proportional to body size.
The diet of these fishes generally consists of food composed of small plant fragments, detritus and algae, organic sediments, small invertebrates, and incidental ingestion of eggs of other fish species [14-16].
Due to their detritivores habits and demersal distribution, it is unclear whether these fish consume plastic particles directly from the water column and substrate [17]. Also, if they accidentally ingest plastics indirectly by consuming prey that had eaten plastics themselves. [18] showed a significant abundance of microplastic particles in the digestive tract of Pterygoplichthys pardalis at two different research stations, with fragments (34.92% and 37.76%, respectively) being the most abundant form of microplastic. Contrary to the research by [19], which showed that fibers were more abundant in the digestive tract of P. pardalis, indicating a selective feeding of fragments and fibers over other types of plastics on this species. Likewise, studies have recorded that some freshwater demersal fish species with feeding habits similar to those of P. multiradiatus have observed high concentrations of microplastics in stomach content analyses but do not ascertain whether it is part of the diet of these species [20-22]. It is currently unknown whether ingestion of small amounts of microplastics is detrimental to the health of these fish. However, understanding the levels of plastic pollution in fish populations requires understanding these trophic linkages.
In summary, these findings indicate that both smaller plastic particles and organic matter are easier for pleco to ingest than larger particles when exposed to a variety of sizes and shapes in their natural habitat, but do not ensure that these materials are preferred in the diet of this species. Likewise, these results indicate that ingestion of plastic and/or organic matter is low even when food availability is restricted and is not related to the body size of the fish. The literature documents that both plastic and organic matter arrive in nature irregularly [23], in addition to the fact that the aquatic fauna of Puerto Rico depends on the input and disposal of organic matter in water bodies. Consequently, fish can differentiate food particles from non-food particles and may experience intermittent periods of low food rationing that reduce fitness to feel compelled to consume exposed food. More research is needed to determine if exposure to different plastic particles will have a greater impact on P. multiradiatus fish populations and learn more about the diet of plecos, as they may have a more specific feeding preference found in their natural habitat.
Funding
This study was supported by the Puerto Rico Center for Environmental Neuroscience (PRCEN), grant # HRD-11736019 (PRCEN2) and the Shrimp and Fish Ecology Laboratory of the University of Puerto Rico at Río Piedras campus. The authors declare no competing financial interests.
Ethical Statement
Ethical review and approval for this study was approved by the Institutional Animal Care and Use Committee (IACUC), protocol number 2021-02-15-3027, at the University of Puerto Rico, Río Piedras Campus.
Data Availability Statement
The data that support the findings of this study are available upon reasonable request from the authors.
Acknowledgment
Thanks to Graciela López Bonano for her appreciated help in the field and to the members of the Shrimp and Fish Ecology Laboratory for their help in searching for materials for the research methodology.
Conflict of Interest
The authors declare no conflicts of interest.
References
- Roebroek CT J, Harrigan S, van Emmerik TH M, Baugh C, Eilander D, Pappenberger F, et al. (2021) Plastic in global rivers: are floods making it worse? Environmental Research Letters 16(2).
- van Emmerik T, Schwarz A (2019) Plastic debris in rivers. Wiley Interdisciplinary Reviews, Water: 7(1).
- Horton AA, Jürgens MD, Lahive E, van Bodegom PM, Vijver MG (2018) The influence of exposure and physiology on microplastic ingestión by the freshwater fish Rutilus rutilus (roach) in the River Thames, UK. Environmental Pollution 236: 188–194.
- Biginagwa FJ, Mayoma BS, Shashoua Y, Syberg K, Khan FR (2016) First evidence of microplastics in the African Great Lakes: Recovery from Lake Victoria Nile perch and Nile tilapia. Journal of Great Lakes Research, 42(1): 146-149.
- De Souza Petersen E, Krüger L, Dezevieski A, Petry MV, Montone RC (2016). Incidence of plastic debris in Sooty Tern nests: A preliminary study on Trindade Island, a remote area of Brazil. Marine Pollution Bulletin, 105(1): 373-376.
- Windsor FM, Durance I, Horton AA, Thompson RF, Tyler CR, et al. (2019) A catchment‐scale perspective of plastic pollution. Global Change Biology 25(4): 1207-1221.
- Redondo-Hasselerharm PE, Falahudin D, Peeters ETHM, Koelmans AA (2018) Microplastic Effect Thresholds for Freshwater Benthic Macroinvertebrates. Environmental Science & Technology 52(4): 2278-2286.
- Hurley R, Woodward J, Rothwell JJ (2017) Ingestion of Microplastics by Freshwater Tubifex Worms. Environmental Science & Technology 51(21): 12844-12851.
- Reynolds C, Ryan PG (2017) Micro-plastic ingestion by waterbirds from contaminated wetlands in South Africa. Marine Pollution Bulletin 126: 330-333.
- Critchell K, Hoogenboom MO (2018) Effects of microplastic exposure on the body condition and behavior of planktivorous reef fish (Acanthochromis polyacanthus). PLOS ONE 13(3): e0193308.
- García‐Berthou E (1999) Food of introduced mosquitofish: ontogenetic diet shift and prey selection. Journal of Fish Biology 55(1): 135-147.
- Mendoza RE, Cudmore B, Orr R, Balderas SC, Courtenay WR, et al. (2009) Trinational Risk Assessment Guidelines for Aquatic Alien Invasive Species. Commission for Environmental Cooperation. 393, rue St- Jacques Ouest, Bureau 200, Montreal (Québec), Canada. ISBN 978-2-923358-48-1.
- Morét-Ferguson S, Law KL, Proskurowski G, Murphy EK, Peacock EE, et al. (2010) The size, mass, and composition of plastic debris in the western North Atlantic Ocean. Marine Pollution Bulletin, 60(10): 1873–1878.
- Nico L, Loftus W, Reid J (2009) Interactions between non-native armored suckermouth catfish (Loricariidae: Pterygoplichthys) and native Florida manatee (Trichechus manatus latirostris) in artesian springs. Aquatic Invasions 4(3): 511–519.
- Covain R, Fisch-Muller S (2007) The genera of the Neotropical armored catfish subfamily Loricariinae (Siluriformes: Loricariidae): a practical key and synopsis. Zootaxa, 1462(1): 1–40.
- Hoover JJ, Killgore KJ, Cofrancesco AF (2004) Suckermouth Catfishes: Threats to Aquatic Ecosystems of the United States? Aquatic Nuisance Species Research Program Bulletin: 1-13.
- Angulo-Olmos GG (2022) Microplásticos en el tracto gastrointestinal del bagre armado (Pterygoplichthy spp.) recolectados en una laguna urbana de la llanura de inundación del río Grijalva.
- Deriano A, Nurdin E, Patria MP (2021) Analisis Kelimpahan Mikroplastik pada Ikan Sapu-sapu Pterygoplichthys pardalis (Castelnau, 1855), Air, dan Sedimen di Dua Daerah Ciliwung, Jakarta Selatan Analysis of Microplastic Abundance in Sailfin Catfish Pterygoplichthys pardalis (Castelnau, 1855), Water, and Sediment in Two Ciliwung Areas, South Jakarta.
- Putri NR, Nurdin E, Patria MP (2021) Analysis of microplastics abundance in water, sediment, and digestive tract and gills of Amazon sailfin catfish Pterygoplichthys pardalis (Castelnau, 1855) in Situ Tipar, Depok, West Java, Indonesia. In The 2ndNational Conference for Ummah Network 2021 (INTER-UMMAH 2021) And the 3rd International Conference on Universal Wellbeing 2021 (ICUW 2021) “Edu Sandbox: competency development and innovative strategies for a new normal agenda." (Volume 2) ISBN 978-616-7773-37-7 (p. 253).
- Yuan W, Liu X, Wang W, Di M, Wang J (2019) Microplastic abundance, distribution and composition in water, sediments, and wild fish from Poyang Lake, China. Ecotoxicology and Environmental Safety 170: 180-187.
- McNeish RE, Kim L H, Barrett H, Mason S, Kelly JJ, et al. (2018) Microplastic in riverine fish is connected to species traits. Scientific Reports 8(1).
- Silva-Cavalcanti JS, Silva JD B, de França EJ, de Araújo MC B, Gusmao F (2017) Microplastics ingestion by a common tropical freshwater fishing resource. Environmental pollution 221: 218-226.
- Anderson A, Andrady A, Hidalgo-Ruz V, Kershaw PJ (2015) Sources, Fate and Effects of Microplastics in the Marine Environment: a Global Assessment; GESAMP Joint Group of Experts on the Scientific Aspects of Marine Environmental Protection.
-
Edgar J Lozada-Gómez* and Omar Pérez-Reyes. Determination of Food Preference in Pterygoplichthys multiradiatus. Online J Ecol Environ Sci. 1(5): 2024. OJEES.MS.ID.000522.
-
Armored Catfish; Exposure; Fish; Organic Matter; Plastic; Pleco
-
This work is licensed under a Creative Commons Attribution-NonCommercial 4.0 International License.