Review Article
Anaerobic Digestion of Solid Waste A Review of the State of the Art
Marcos Batista Figueredo1*, Alexandre do Nascimento Silva1,2, José Roberto de Araújo Fontoura1, Roberto Luiz Souza Monteiro3 and Marcos Antônio Felipe Santos1
1Graduate Program in Modeling and Simulation of Biosystems, University of the State of Bahia, Department of Exact and Earth Sciences II, Brazil
2State University of Santa Cruz, Department of Engineering and Computing, Brazil
3Senai Cimatec University Center, Brazil
Marcos Batista Figueredo, Graduate Program in Modeling and Simulation of Biosystems, University of the State of Bahia, Department of Exact and Earth Sciences II, Brazil.
Received Date:August 11, 2023; Published Date: October 19, 2023
Abstract
Urban Solid Organic Waste (SOW) disposal and accumulation could reach critical levels in almost all world regions. These organic residues must be treated to avoid the depletion of natural resources and thus minimize the risks of loss of ecosystem balance. Several methods are currently applied to the treatment and management of SOW. This review focuses on anaerobic digestion, considered one of the most viable options for recycling small amounts of organic matter. This work provides an overview of the process, its advantages, and disadvantages in producing (biogas). The literature establishes that the involvement of a mix of microorganisms and the effects of co-substrates and environmental factors on the efficiency of the process has been comprehensively addressed. This indicates that anaerobic digestion can be an attractive option for converting raw solid organic waste into valuable products, such as biogas and other energy-rich compounds, which can play a critical role in meeting the world’s growing energy needs.
Keywords:Anaerobic digestion; Urban solid organic waste; Biodigester
Introduction
The emission of environmental gases and reduced energy resources created the need to search for clean and renewable energies [1,2]. Biomass is a vital renewable resource that can replace fossil fuels [3]. In this line and considering that in 2019, the generation of Urban Solid Waste (SOW) in Brazil was around 79 million tons, corresponding to 380 kg per person per year [4], the production of biogas from SOW has several advantages [5].
The advantages are higher energy production, lower environmental impact, and investment requirements [6]. Biogas is a flammable mixture when the biomethane concentration is greater than 40%. It is produced through a process that involves four steps, 1. hydrolysis; 2. acetogenesis; 3. acetogenesis; 4. methanogenesis through a microbial consortium containing different types of bacteria [7].
Different bacteria, including the genera Clostridium, Cellulomonas, Bacillus, Thermomonospora, Ruminococcus, Baceriodes, Acetovibrio, and Microbispora, carry out the hydrolysis of organic matter. Lactobacillus, Streptococcus, Bacillus, and Escherichia are mainly responsible for acidogenesis. Different genera, including Acetobacterium, Syntrophomonas, Clostridium, Sporomusa, Syntrophospora, Thermosyntropha, and Eubacterium, are involved in acetogenesis. A series of archaebacteria, including Methanococcus, Methanosarcina, and Methanolobus, are responsible for methanogenesis [8]. This microbial consortium needs specific conditions for optimal biogas production: pH in the range of 6-7.5 and temperature in the range of 35-550C [9].
The three primary resources for biogas production include lignocellulosic waste, domestic and urban wastewater, and industrial wastewater [9]. When these wastes are released into the environment without adequate disposal, environmental pollution and harmful impacts may occur. These untreated wastes create different problems with climate change, increasing the number of greenhouse gases [6].
Several methods are available to treat SOW, but anaerobic digestion seems promising (ZHANG et al., 2014). Anaerobic digestion of organic waste in landfills releases methane and carbon dioxide gases that escape into the atmosphere and pollute the environment [6]. Under controlled conditions, the same process has the potential to provide valuable products such as biofuel and organic additives (soil conditioner), and the treatment system does not require an oxygen supply [10].
The expansion of urban areas, driven by population growth and the complexity of waste that present a high risk of contamination, makes it essential to define and implement adequate public policies to ensure the correct disposal of solid waste. According to Moustakas et al. (2020), the National Solid Waste Policy (NSWP), established by Law No. 12,305 in 2010, stipulates guidelines and instruments for the integrated management and appropriate management of solid waste for the public and private sectors.
According to a more recent study by Da Silva et al. (2022), rapid urban expansion has significantly increased solid waste generation, demanding a comprehensive and sustainable approach to its proper management. This need is highlighted by Silva [11], who emphasizes the importance of effective public policies that consider the complexity of waste and promote recycling and waste reduction practices to mitigate the associated environmental and health impacts.
Furthermore, in the studies by Júnior et al. [12] emphasize the importance of civil society participation in implementing the PNRS through mechanisms of environmental education and awareness to promote a change in behavior about solid waste. It is essential, therefore, to establish partnerships between the different actors involved, such as governments, industries, and the community, aiming at more efficient and sustainable management of urban solid waste [13]. Thus, the implementation of adequate public policies, as established by the PNRS, and the active involvement of all sectors of society are essential to face the challenges related to solid waste disposal in an urban context in constant expansion [14].
In this context, methane and hydrogen as potential fuels are considered comparatively cleaner than fossil fuels and have the advantage of not relying on fossil fuels for energy consumption. Thus, anaerobic digestion represents an opportunity to decrease environmental pollution and, at the same time, provide biogas and organic fertilizer or transport material for bio-fertilizers [15]. This review concentrated on the study of obtaining biogas through anaerobic digestion, thinking about it as a source of renewable energy and also as a raw material for the production of other fuels.
Renewable Energy
The Sustainable Development Goals (SDGs), established by the United Nations (UN) in 2015, are a series of global goals that aim to address the world’s leading social, economic, and environmental challenges. Consisting of 17 objectives and 169 associated targets, the main objective of the SDG is to promote sustainable development that balances present needs without compromising future generations.
The SDGs cover a wide range of issues, including poverty eradication, zero hunger, health and well-being, quality education, gender equality, clean water and sanitation, affordable and clean energy, decent work and economic growth, innovation, and infrastructure, reducing inequalities, sustainable cities and communities, responsible consumption, and production, climate action, life in water, life on land, peace, justice, and effective institutions, partnerships for sustainable development.
These goals have a time horizon up to 2030 and are interlinked, meaning progress on one goal is often linked to progress on other goals. In addition, the SDGs are designed to be achieved in an integrated manner, considering the three pillars of sustainable development: economic, social, and environmental. The SDGs provide a comprehensive and ambitious framework for tackling pressing global challenges and have been widely adopted by governments, the private sector, non-governmental organizations, and civil society around the world. They represent a call to collective action and the engagement of all stakeholders to achieve a more sustainable future for the planet and all people.
Renewable energy is an efficient solution for growing energy demand and reducing greenhouse gas emissions. In addition, it plays a crucial role in ensuring energy security, improving environmental protection, and stimulating job growth in several countries (LI et al., 2020). There is a continuous expansion of the global development of renewable sources and their expansion in scale; at the same time, implementation costs have decreased [16]. It is estimated that the world’s renewable energy consumption will increase by about 3% per year between 2018 and 2050, making it the main source of primary energy consumption by 2050 [17].
The most significant renewable energy sources include wind energy, solar photovoltaic energy, and biomass energy, emphasizing hydroelectric, geothermal, and marine energy. Wind and solar photovoltaic technologies have expanded considerably, providing a relatively affordable energy source (Deshmukh Et Al., 2021). However, these systems are characterized by highly variable and not always predictable energy output. On the other hand, hydroelectric energy depends on the rainfall regime, which can affect a country’s energy security. An example is Brazil, whose energy matrix is highly dependent on water sources and faced severe energy supply problems during a prolonged drought in 2001, drastically reducing hy droelectric generation capacity due to decreased reservoir levels [18].
On the other hand, biomass energy conversion technologies can provide a constant baseload and help balance the gaps between supply and demand in the energy sector. These techniques make it possible to use organic waste, such as agricultural and forestry waste, to continuously and reliably generate energy. In summary, although wind and solar photovoltaic energy are promising renewable energy sources, their variability can pose challenges to the stability of the energy supply. Hydroelectric power, in turn, is influenced by weather conditions. In this context, biomass technologies can play an essential role in providing a constant energy source, helping to fill the supply and demand gaps in the energy sector
Biomass is a carbon-neutral resource as well as a source of C/H/O elements to generate carbon-based organic products such as bioenergy (biofuel and biogas) and chemicals (biorefinery) (Jung et al., 2021). Thus, the valorization of biomass raw material has received considerable attention in recent decades, and biomass- based energy sources should have representative participation in the energy system of the future [19] Jung Et Al., 2021. From an energy point of view, biomass can be understood as any renewable resource derived from organic matter (of animal or vegetable origin) which can be used for energy production (Aziz; Hanafiah; Gheewala, 2019).
In recent years, there has been significant growth in renewable energies, and their competitiveness has increased. According to data from the International Renewable Energy Agency (IRENA, 2020), global installed renewable energy capacity has more than doubled in the last decade, driven by the development and consolidation of new renewable energy sources. By the end of 2020, global installed renewable energy capacity reached 2,789,061 MW. The distribution of this capacity is as follows: hydroelectric energy corresponds to 43.41%, solar energy 25.37%, wind energy 26.29%, bioenergy 4.41%, geothermal energy 0.5%, and marine energy 0.02%.

The new dataset from SIGA (the Information System of Generation from ANEEL), figure 1, provides valuable insights into Brazil’s energy generation enterprises. It comprehensively examines the diverse fuel sources, focusing on five significant modalities: fossil, biomass, solar, wind, and hydroelectric energy. Our analysis suggests a promising trend in Brazil’s pursuit of amplifying its renewable energy network. The country is inclined to harness environmentally friendly, sustainable energy sources. A steady increase in the use of biomass, solar, wind, and hydroelectric power can be observed, indicating the ongoing transition from traditional fossil fuels to greener alternatives. As Brazil continues this trajectory, it strengthens its position as a global leader in renewable energy generation, contributing significantly to climate change mitigation efforts.
Anaerobic Digestion
Anaerobic digestion of organic waste is a process by which almost all organic waste can be biologically transformed into another form without oxygen. From organic matter, a gaseous mixture is formed consisting of 50-75% methane and 25-50% carbon dioxide. There are also small amounts of hydrogen, hydrogen sulfide, ammonia, and other waste gases in biogas [20]. The biogas production process consists of four biochemical reactions carried out by different groups of microorganisms, coinciding in a one-step process, namely, hydrolysis: the first reaction is liquefaction.
The production of biogas (Figure 2) through anaerobic digestion involves four main steps: hydrolysis, acidogenesis, acetogenesis, and methanogenesis. These steps describe the biochemical process in which organic waste is decomposed by bacteria in an oxygen-free environment, resulting in the production of biogas, mainly composed of methane (CH4) and carbon dioxide (CO2).
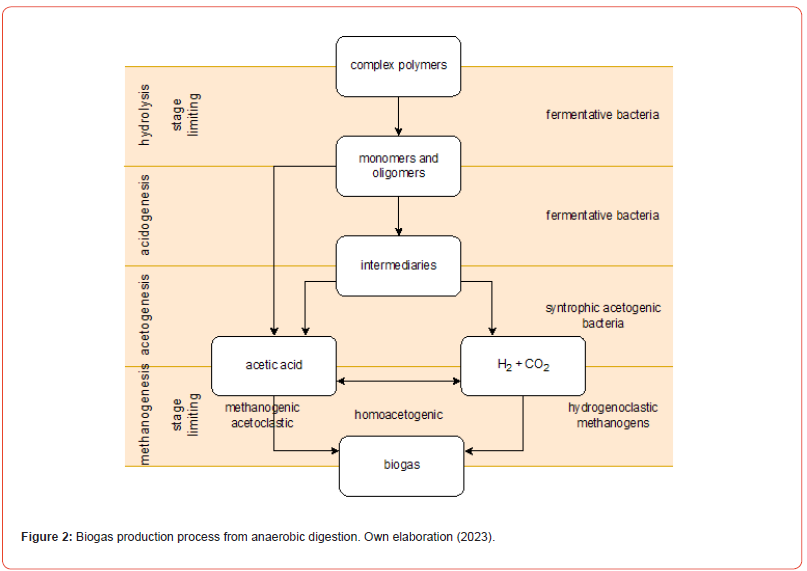
Long-chain organic compounds (fats, proteins) are broken down into simple organic compounds (amino acids, sugars) by enzymes released by bacteria. Acidogenesis: Hydrolysis products are converted into organic acids by acid-forming bacteria. Acetate, hydrogen, and carbon dioxide are also formed and used as starting substances for methane formation. Acetogenesis: Organic acids and alcohols are converted to acetic acid, hydrogen, and carbon dioxide by acetogenic bacteria. The hydrogen produced must be consumed by methanogenic microorganisms, as excess hydrogen inhibits the formation of acetic acid. Methanogenesis: The products of the previous reactions are converted into methane, carbon dioxide, and water by methanogenic microorganisms (Wellinger; Murphy; Baxter, 2013) [21].
Table 1:Main parameters of the anaerobic digestion process. Own elaboration (2023).
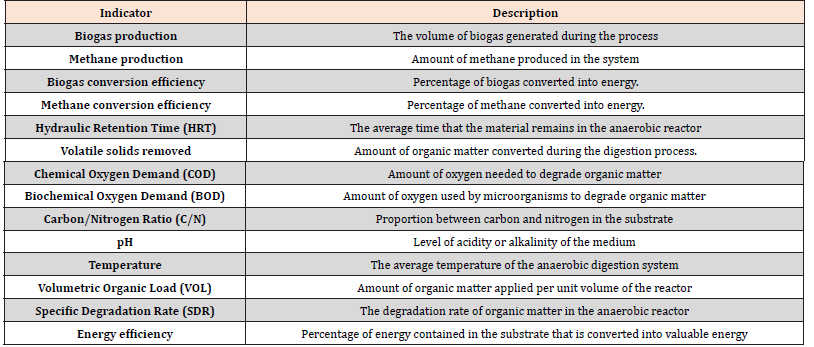
Anaerobic digestion applies0 to a wide range of wastes (Table 1). In addition, this process has some advantages over the aerobic process due to a low energy requirement for operation and a low biomass production [22], it is considered a viable technology in the competent treatment of organic waste and the simultaneous production of renewable energy [20]. Anaerobic digestion of organic waste is also an environmentally valuable technology; both Singh & Basak [23] and Kumar & Samadder [24] described the benefits of this process to reduce environmental pollution in two main ways: the sealed environment of the process prevents the of methane into the atmosphere while burning methane will release carbon-neutral carbon dioxide (with no net effect on the atmosphere of carbon dioxide and other greenhouse gases).
On the other hand, the anaerobic process has some disadvantages, such as long retention times and low removal efficiency of organic compounds. Another problem is efficiency; there are still no new technologies to simplify the process, making it abundant and low-cost [25]. After refining and compression, the biogas still contains impurities. If the generated biofuel is used to power cars, it can corrode the metallic parts of the engine. This corrosion would lead to increased maintenance costs. The gaseous mixture is more suitable for cooking stoves, water boilers, and lamps [26].
Table 2:Biogas chemical composition. Own elaboration (2023).
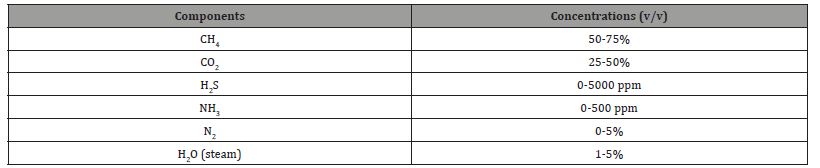
For an effective integration into the biofuel sector, biogas production through anaerobic digestion must be feasible in technical and economic terms. This viability must be compared not only with other sources of bioenergy but also with traditional fossil fuels. In addition, the biogas production must be technically adequate for the implantation site. Biogas, as an energy source, is a viable option subject to continuous improvements and optimizations. This means that technical advances and improvement strategies can be applied to make the biogas production process more efficient, sustainable, and profitable (Postawa; Szczygieł; Kułażyński, 2021).
Thus, the production of biogas through anaerobic digestion has the potential to establish itself as a viable energy alternative, capable of contributing to the reduction of dependence on fossil fuels and providing economic, environmental, and social benefits. Continued research and development in this area are crucial to further boost the efficiency and competitiveness of biogas as a sustainable energy solution.
Anaerobic Co-Digestion
Anaerobic co-digestion (AcoD) is the instantaneous digestion of two or more substrates and mixtures of co-substrates [27]. AcoD can also be called “co-fermentation.” The use of AcoD of different organic materials in a biodigester can increase the stability of the anaerobic process due to a better balance of carbon to nitrogen (C/N) [28], which accelerates the biodegradation of solid organic waste through biostimulation. According to Hagos et al. [29], co-digestion can, in addition, alleviate the inhibitory effect of high concentrations of ammonia and sulfide. Anaerobic co-digestion of sludge residues with fruit, vegetable, and food residues is a promising technology, offering many advantages, including a balanced C/N ratio, inhibition of ammonia and high biogas production, and optimizing anaerobic digestion performance. Moreover, biogas recovery is already well-used in China, India, and Sri Lanka [30]. The use of a co-substrate with a waste of low nitrogen and lipid content increases the production of biogas due to the complementary characteristics of both types of waste, thus reducing the problems associated with the accumulation of intermediate volatile compounds and high concentrations of ammonia [31].
The Anaerobic Bioreactor
Anaerobic bioreactors have potential applications for the digestion of solid organic waste and can reduce the environmental load compared, for example, to conventional sanitary landfills [32]. Research has observed that bioreactor modeling exerts a strong influence on its performance [33], and thus a variety of bioreactors have been developed in recent years [34].
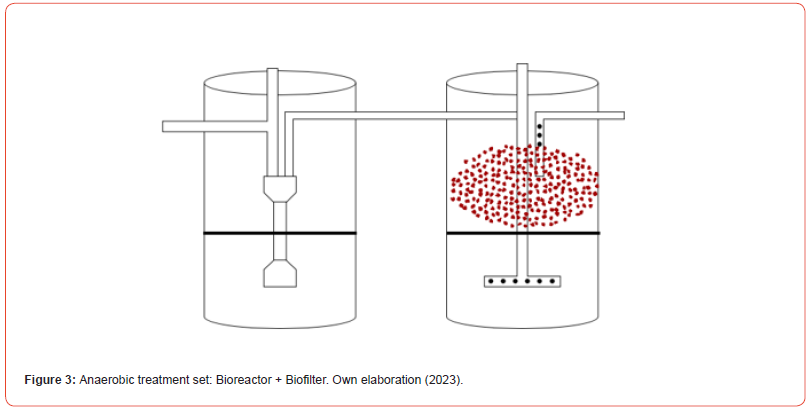
According to Sadino-Riquelme et al. [35] and Paul et al. [36], the modeling of an anaerobic bioreactor (Figure 3) presents a correct description of the mixing conditions present there. It is necessary to represent the process adequately. This is especially true for fullscale installations, as the influence of hydrodynamic conditions on overall behavior depends on scale. However, they point out that the modeling assumes perfect mixing conditions: media homogeneity.
Several bioreactors are currently in use, including batch reactors, which are the simplest, filled with the raw material and left for a period that can be considered the hydraulic retention time, after which they are emptied [37]. They are helpful because they can perform quick digestion with simple and cheap equipment and are also useful for evaluating the digestion rate efficiently [38]. On the other hand, it has some limitations, such as high fluctuations in gas production and quality, biogas losses during the emptying of bioreactors, and restricted heights of the bioreactor [39]. The second type of bioreactor is known as a one-stage continuous feed system, where all biochemical reactions occur in one bioreactor. The third type of bioreactors is continuously fed two-stage or multi-stage systems in which various biochemical processes such as hydrolysis, acidification, acetogenesis, and methanogenesis occur separately [40,41].
Anaerobic digestion of organic material involves different degradation steps [42]. According to Tong et al. [43], the microorganisms participating in the process may be specific to each degradation step and, therefore, may have different environmental requirements. The involvement of a diverse range of microorganisms and the effects of co-substrates and environmental factors on the efficiency of the process was comprehensively addressed, emphasizing temperature, pH, humidity, substrate, and nitrogen in this work.
The temperature affects the anaerobic digestion of various biomasses, which can bring about significant changes and effects on the microbial community, process kinetics and stability, and methane yield [44]. Lower temperatures during the process decrease microbial growth, substrate utilization rates, and biogas production [24]. Furthermore, lower temperatures can also result in cellular energy depletion, leakage of intracellular substances, or complete lysis. In contrast, high temperatures reduce biogas yield due to the production of volatile gases such as ammonia, which suppress methanogenic activities [36].
Generally, anaerobic digestion is carried out at mesophilic temperatures. Operation in the mesophilic range is more stable and requires less energy expenditure (ZOU et al., 2020). Generally, a temperature range between 35-37◦C is considered suitable for methane production, and a temperature shift from mesophilic to thermophilic can cause a sharp drop in biogas production until the required populations increase in number [45].
The effect of the Hydrogenionic potential (pH) in the process manifests itself directly by affecting the enzymatic activity or indirectly by affecting the toxicity of several compounds [46]. However, the ideal pH for methanogenesis was around 7,0 [31]. Guštin and Marinšek-Logar [47] reported a very narrow range of suitable pH (7.0-7.2) in 18 experiments carried out with wastewater. Likewise, Ravi et al. [46] noted that a pH range of 6.8 - 7.2 was optimal for anaerobic digestion. The works by Carotenuto et al. [48] and Eryildiz, Taherzadeh et al. [49] showed that the most favorable pH range to reach the maximum biogas yield in anaerobic digestion is 6.5-7.5.
High moisture contents generally facilitate anaerobic digestion [50]; however, in the study by Lay, Li & Noike [51], the difficulty in maintaining the same water availability throughout the cycle was identified digestion. Initially, water added at a high rate is reduced to a lower level as the anaerobic digestion process proceeds. High water content can affect process performance by dissolving easily degradable organic matter. It has been reported that the highest methane production rates occur at 60-80% humidity [52].
The anaerobic digestion rate is strongly affected by the type, availability, and complexity of the substrate [6,39, 36]. Different types of carbon sources support different groups of microbes (AL-ADDOUS et al., 2019). Before starting a digestion process, the substrate can be characterized as to the content of carbohydrates, lipids, proteins, and fibers [41]. In addition, the substrate must also be characterized as to the amount of methane that can potentially be produced under anaerobic conditions [20,34]. Carbohydrates are considered the most essential organic component of urban solid waste for biogas production [36]. However, starch can be an effective, low-cost substrate for biogas production compared to sucrose and glucose. According to Choong, Chou & Norli [10] and Phuttaro et al. [44], it was observed that the initial concentration and total solid content of the substrate in the bioreactor could significantly affect the process performance and the amount of methane produced during the process [25].
Nitrogen is essential for protein synthesis and is mainly required as a nutrient by microorganisms in anaerobic digestion [53]. Nitrogenous compounds in organic waste are usually proteins converted to ammonium by anaerobic digestion. In the form of ammonia, nitrogen contributes to stabilizing the pH value of the bioreactor where the process is taking place [34]. Ammonia in high concentrations can inhibit the biological process and methanogenesis in concentrations that exceed approximately 100 mM [49]. Kumar & Samadder [24] observe that the amount of ammonia in the digester can also affect the production of hydrogen and the removal of volatile solids [54-58].
Final Considerations
This review indicates that anaerobic digestion is one of the
most effective biological processes for treating solid organic waste
and sludge. The main advantages of this technology include
1. low-nutrient organic waste can be degraded by co-digestion
with different substrates in anaerobic bioreactors,
2. The process simultaneously leads to the production of
biogas that can be applied to homes or sites in remote locations,
which could be vital to meeting future energy needs.
However, different factors, such as substrate and co-substrate composition and quality, environmental factors (temperature, pH, organic load rate), and microbial dynamics, contribute to the efficiency or failure of the anaerobic digestion process. They must be optimized to achieve the maximum possible benefit from this technology regarding energy production and organic waste management.
Acknowledgement
None.
Conflict of Interest
No conflict of interest.
References
- Hagman L (2018) The role of biogas solutions in sustainable biorefineries. Journal of Cleaner Production 172: 3982-3989.
- Orjuela Castro JA, Aranda Pinilla JA, Moreno Mantilla, CE (2019) Identifying trade-offs between sustainability dimensions in the supply chain of biodiesel in Colombia. Computers and Electronics in Agriculture 161: 162-169.
- Paolini V (2018) Environmental impact of biogas: A short review of current knowledge. Journal of Environmental Science and Health, Part A 53(10): 899-906.
- ABRELPE EA (2018/2019) Panorama dos resíduos sólidos no Brasil. São Paulo: Grappa. Disponível em, Brasil.
- Balat M, Balat H (2009) Biogas as a renewable energy source-a review. Energy Sources Part A 31: 1280-1293.
- Hengeveld E (2020) Potential advantages in heat and power production when biogas is collected from several digesters using dedicated pipelines-a case study in the province of west-flanders (Belgium). Renewable Energy 149: 549-564.
- Al-Rubaye H (2019) Process simulation of two-stage anaerobic digestion for methane production. Biofuels 10: 181-191.
- Pampilón González L (2017) Archaeal and bacterial community structure in an anaerobic digestion reactor (lagoon type) used for biogas production at a pig farm. Journal of Molecular Microbiology and Biotechnology 27(5): 306-317.
- Achinas S, Achinas V, Euverink GJW (2017) A technological overview of biogas production from biowaste. Engineering 3(3): 299-307.
- Choong YY, Chou KW, Norli I (2018) Strategies for improving biogas production of palm oil mill effluent (pome) anaerobic digestion: A critical review. Renewable and Sustainable Energy Reviews 82: 2993-3006.
- Silva TSD (2023) Solid waste management in the municipality of Ibatiba, Espírito Santo: an assessment of the goals of the municipal plan for integrated solid waste management.
- Júnior AOS, Nascimento AFP (2021) Ten years of implementation of the national solid waste policy: possibilities for changing the management paradigm based on participation social: Studies in Social Sciences Review 2(2): 69-85.
- Mattos FV, Pinho GC de S, Ramalho JCM, Calmon JL, Siman RR (2022) Sustainable management of urban solid waste based on LCA, AECV and ACVS: perspectives and paths for Brazil and developing countries. Brazilian Journal of Development 8(4): 22763-22774.
- Cigainski Lisbinski F, Balestrin Flores CE, da Silva DM, Pereira Bisognin R, Gehlen Bohrer RE (2020) A importância dos consórcios públicos na gestão dos resíduos sólidos urbanos: uma análise do consórcio intermunicipal CIGRES. Revista Gestão & Sustentabilidade Ambiental 9(2): 3-36.
- Medeiros RF, Amorim EB, Girotto V (2017) Transformation and obtaining of methane biogas through anaerobic biodigesters for energy generation. REGRAD-Electronic Journal of Graduation of the UNIVEM 10: 339-353.
- Lu J, GAO X (2021) Biogas: Potential, challenges, and perspectives in a changing China. Biomass and Bioenergy 150: 106127.
- Energy Information Administration (EIA) (2019) International Energy Outlook 2023. Disponível em: Acesso em, Brazil.
- Freitas FF (2019) The Brazilian market of distributed biogas generation: Overview, technological development, and case study. Renewable and Sustainable Energy Reviews 101: 146-157.
- Miltner M, Makaruk A, Harasek M (2017) Review on available biogas upgrading technologies and innovations towards advanced solutions. Journal of Cleaner Production 161: 1329-1337.
- Banu R, Kannah Y (2019) Anaerobic Digestion. BoD-Books on Demand.
- Cheng J (2017) Biomass to renewable energy processes. CRC Press.
- Angelidaki I, Ellegaard L, Ahring BK (2003) Applications of the anaerobic digestion process. Adv Biochem Eng Biotechnol 82: 1-33.
- Singh A, Basak P (2018) Economic and environmental evaluation of municipal solid waste management system using industrial ecology approach: Evidence from India. Journal of Cleaner Production 195: 10-20.
- Kumar A, Samadder S (2020) Performance evaluation of anaerobic digestion technology for energy recovery from organic fraction of municipal solid waste: A review. Energy 197: 117253.
- Kariyama ID, Zhai X, Wu B (2018) Influence of mixing on anaerobic digestion efficiency in stirred tank digesters: a review. Water Research 143: 503-517.
- Korbag I (2020) Recent advances of biogas production and future perspective. In Biogas. IntechOpen.
- Nghiem LD (2017) Full scale co-digestion of wastewater sludge and food waste: Bottlenecks and possibilities. Renewable and Sustainable Energy Reviews 72: 354-362.
- Al-Addous M (2019) Evaluating biogas production from the co-digestion of municipal food waste and wastewater sludge at refugee camps using an automated methane potential test system. Energies 12: 32.
- Hagos K (2017) Anaerobic co-digestion process for biogas production: Progress, challenges and perspectives. Renewable and Sustainable Energy Reviews 76: 1485-1496.
- Khayum N, Anbarasu S, Murugan S (2018) Biogas potential from spent tea waste: A laboratory scale investigation of co-digestion with cow manure. Energy 165: 760-768.
- Mao C (2017) Process performance and methane production optimizing of anaerobic co-digestion of swine manure and corn straw. Scientific Reports 7(1): 9379.
- Ji J (2020) Application of two anaerobic membrane bioreactors with different pore size membranes for municipal wastewater treatment. Science of The Total Environment 745: 140903.
- Prajapati KB, Singh R (2018) Kinetic modelling of methane production during bio-electrolysis from anaerobic co-digestion of sewage sludge and food waste. Bioresource Technology 263: 491-498.
- Clercq DD, Wen Z, Fei F (2019) Determinants of efficiency in anaerobic bio-waste co-digestion facilities: A data envelopment analysis and gradient boosting approach. Applied Energy 253: 113570.
- Sadino Riquelme C (2018) Computational Fluid Dynamic (CFD) modelling in anaerobic digestion: General application and recent advances. Critical Reviews in Environmental Science and Technology 48(1): 39-76.
- Paul A (2020) Biogas production and uses. In Biorefinery Production Technologies for Chemicals and Energy. John Wiley and Sons. pp. 347.
- Pieber B (2018) Continuous heterogeneous photocatalysis in serial micro-batch reactors. Angewandte Chemie International Edition 57(31): 9976-9979.
- Jaramillo F (2018) Advanced strategies to improve nitrification process in sequencing batch reactors-a review. Journal of Environmental Management 218: 154-164.
- Abuabdou SM (2020) A review of anaerobic membrane bioreactors (anmbr) for treating highly contaminated landfill leachate and biogas production: effectiveness, limitations and future perspectives. Journal of Cleaner Production 255: 120215.
- Hans M, Kumar S (2019) Biohythane production in two-stage anaerobic digestion system. International Journal of Hydrogen Energy 44(32): 17363-17380.
- Algapani DE (2019) Bio-hydrogen and bio-methane production from food waste in a two-stage anaerobic digestion process with digestate recirculation. Renewable Energy 130: 1108-1115.
- Shahidul M (2018) Optimization of factors affecting biogas production from POME. Sci Int 30(6): 851-859.
- Tong J (2018) Factors influencing the fate of antibiotic resistance genes during thermochemical pretreatment and anaerobic digestion of pharmaceutical waste sludge. Environmental Pollution 243: 1403-1413.
- Phuttaro C (2019) Anaerobic digestion of hydrothermally-pretreated lignocellulosic biomass: Influence of pretreatment temperatures, inhibitors and soluble organics on methane yield. Bioresource Technology 284: 128-138.
- Liu C (2018) Biogas production and microbial community properties during anaerobic digestion of corn stover at different temperatures. Bioresource Technology 261: 93-103.
- Ravi PP (2018) Effects of target pH-value on organic acids and methane production in two-stage anaerobic digestion of vegetable waste. Bioresource Technology 247: 96-102.
- Gustin S, Marinsek Logar R (2011) Effect of pH, temperature, and air flow rate on the continuous ammonia stripping of the anaerobic digestion effluent. Process Safety and Environmental Protection 89(1): 61-66.
- Carotenuto C (2016) Temperature and pH effect on methane production from buffalo manure anaerobic digestion. International Journal of Heat and Technology 34: S425-S429.
- Eryildiz B, Taherzadeh MJ (2020) Effect of pH, substrate loading, oxygen, and methanogens inhibitors on Volatile Fatty Acid (VFA) production from citrus waste by anaerobic digestion. Bioresource Technology 302: 122800.
- Fujishima S, Miyahara T, Noike T (2000) Effect of moisture content on anaerobic digestion of dewatered sludge: ammonia inhibition to carbohydrate removal and methane production. Water Science and Technology, 41(3): 119-127.
- Lay JJ, Li, YY, & Noike T (1997) Influences of pH and moisture content on the methane production in high-solids sludge digestion. Water Research 31(6): 1518-1524.
- Liu G (2007) Research progress in anaerobic digestion of high moisture. Agricultural Engineering International: CIGR Journal.
- Tanimu MI (2014) Effect of carbon to nitrogen ratio of food waste on biogas methane production in a batch mesophilic anaerobic digester. International Journal of Innovation, Management and Technology 5(2): 116.
- Da Silva MHC, de Lima LNF, Silva C, da Silva BV, de Almeida Tavares HS, et al. (2020) Resíduos sólidos: o uso da gestão ambiental como ferramenta para o manejo adequado do lixo urbano. Brazilian Journal of Development 6(11): 85668-85677.
- Deshmukh MKG (2023) Renewable energy in the 21st century: A review. Materials Today: Proceedings 80(3): 1756-1759.
- Gao Y (2018) A review of recent developments in hydrogen production via biogas dry reforming. Energy Conversion and Management 171: 133-155.
- Leung DY, Wang J (2016) An overview on biogas generation from anaerobic digestion of food waste. International Journal of Green Energy 13: 119-131.
- LI L (2022) Review and outlook on the international renewable energy development. Energy and Built Environment 3(2): 139-157.
-
Marcos Batista Figueredo*, Alexandre do Nascimento Silva, José Roberto de Araújo Fontoura, Roberto Luiz Souza Monteiro and Marcos Antônio Felipe Santos. Anaerobic Digestion of Solid Waste A Review of the State of the Art. Online J Ecol Environ Sci. 1(3): 2023. OJEES. MS.ID.000514.
-
Urban solid organic waste, Biodigester, Higher energy production, Acetogenesis, Methanogenesis, Hydrolysis, Cellulomonas, Bacillus, Ruminococcus, Microbispora, National solid waste policy
-
This work is licensed under a Creative Commons Attribution-NonCommercial 4.0 International License.