Research Article
New Hybrid Hetero-Structure (Zr7O9F10/ZrB2) Ultraviolet (UV) Photo-Catalyst synthesized by Hydrothermal Method
Shahzad Ahmad Khan1*, Ligui Li1, Dengke Zhao1, and Shaowei Chen1,2
1South China University of Technology, China
2Department of Chemistry and Biochemistry, University of California, USA
Shahzad Ahmad Khan, Guangzhou Key Laboratory for Surface Chemistry of Energy Materials, New Energy Research Institute, School of Environment and Energy, South China University of Technology, Guangzhou Higher Education Mega Center, Guangzhou 510006, China.
Received Date: January 30, 2020; Published Date: February 06, 2020
Abstract
Photo-catalyst hybrid hetero-structure material zirconium oxide fluoride and zirconium diboride (Zr7O9F10/ZrB2) with urchin and nano-wall like morphologies were synthesized by hydrothermal method. Hetero-structure (Zr7O9F10/ZrB2) was synthesized by in situ ‘‘incomplete reaction’’. One dimensional Zr7O9F10 nano-needle arrays and two dimensional Zr7O9F10 nano-sheets stand on the surface of ZrB2 cores. Such ultra-violet visible spectra of hetero-structure have a wide absorption peak at 230nm. Absorption edge of the nano-wall and urchin like hetero-structure compared at higher temperature revealed that the absorption edge for nano-wall sample is red shifted due to strong nano-meteric effect and can be considered as photo-catalyst material. Such investigation will broaden the application of photo-catalyst material as described technique can easily be adapted.
Keywords: Chemical Synthesis; Grain growth; Composites; Optical properties; Zr7O9F10/ZrB2
Introduction
Zirconium diboride (ZrB2) based ceramics have substantial interest because of its extreme chemical and physical properties such as, high melting point, superior hardness and low electrical resistance. ZrB2 has several applications such as in Hall-Heroult cell cathodes for electrochemical processing of aluminum, evaporation boats, crucibles for handling molten metal’s, thermowell tubes for steel refining, thermocouple sleeves for high temperature use, nozzles, plasma electrodes, or as a dispersed material in metal and ceramic matrix composites for heaters and igniters [1-4]. Recently, several groups reported that the hetero-structure could markedly improve the distribution of binary hybrid system and increase the active surface of effective composition in comparison to simple hybridization [5,6]. Efforts are made to synthesize binary, triple or multiple hybrid materials with hierarchical architectures in the past decade [7-9]. Oxidation mechanism of ZrB2 as it oxidizes at high temperature which will degrade its properties. Studies on oxidation of ZrB2 and its composites have been carried out to understand the oxidation mechanisms and enhance oxidation resistance [10- 19]. Binary hybrid materials have attracted substantial attention because such materials combine the properties of each unit and provide a great deal of opportunity to explore or enhanced the properties [20,21].
The purpose of this paper is to use ZrB2 as raw material to synthesize a new class of photo-catalyst hybrid hetero-structure material, zirconium oxide fluoride and zirconium diboride (Zr7O9F10/ ZrB2) with urchin and nanowall like morphologies by hydrothermal method. Such materials combine the properties of each unit and provide a great deal of opportunity to explore properties. To the best of our knowledge there is no report about such system. Our facile synthesis provides a direct route for controlling the morphology of hetero-structure and a potential opportunity for selecting the binary hetero-structure. Ultra-Violet visible spectra showed that such hetero-structure can be considered as photo-catalyst material.
Materials and Experiment
Commercially available powder ZrB2, 99.5% (metals basis excluding Hf) Alfa Aesar Inc (US) grain size range 1-2 Micron, oxygen content 1 wt.% and HF (30%, AR) Sinopharm Chemical Reagent Co. Ltd (China) were used. In this experiment ZrB2 (1.41g), HF (1ml) and 50ml deionized H2O were added into a 100ml Teflonlined autoclave and mixed it. Teflon-lined autoclave maintained at 90 °C, 130 °C, 170 °C for 24 h, and then cooled at room temperature. Afterwards the product was centrifuged and washed with deionized water, anhydrous ethanol for five times respectively. The product was dried under vacuum at 80 °C for 12 h. Argon (Ar) gas was passed from the final product at 400 °C for 3 h as protective atmosphere.
The morphologies of samples were analyzed by field emission scanning electron microscopy (FESEM, Hitachi S-4800, Japan) and high resolution transmission electron microscopy (HRTEM, JEOL 2100F, Japan). The composition of the samples were analyzed by an energy dispersive X-ray detector (EDX, Thermo Noran VANTAGESI, 120 kV) and X-ray diffraction XRD, Rigaku Ultima II, Japan) with Cu Kα irradiation. The absorption and reflection spectrum were recorded on a Lambda 35 UV-Vis spectrophotometer at room temperature (PerkinElmer Inc., USA). Before measurement BaSO4 was used as referenced datum mark with 100% reflection. The results showed that ZrB2 oxidized in the environment of HF. However, the oxidization can be minimized through appropriate process.
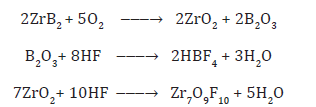
Results and Discussion
From FESEM Fig 1, of experimental samples it was revealed that experimental samples synthesized at different temperatures had different morphology under a strong ultrasonic wave treatment for 1h. ZrB2 had same morphology with experimental sample synthesized at 90 °C except only the surface was evolved to protruding un-smooth structure which was composed of numerous particles on the surface. The experimental sample synthesized at 130 °C was sea-urchin shaped. The nano-needles 90-100nm in diameter with a sharp tip of 35-45nm radiate from the surface of sea-urchin shaped experimental sample. The experimental sample synthesized at 170 °C formed the nano-sheets. The two structures nano-needles and nano-sheets synthesized at temperatures, 130 °C and 170 °C respectively were core/shell structures (Figure 1).
Figure 2, EDX of the experimental sample synthesized at 170 °C verified that Zr, B, O and F were present in the hybrid experimental sample with different percentages (Figure 2).
The XRD Fig 3, of the experimental samples showed that binary hybrid material were hexagonal P6/mmm(191) ZrB2 (JCPDS Card No. 34-0423) and orthorhombic Pbam(55) Zr7O9F10 (JCPDS Card No. 27-0998). The diffraction peaks were with scanning rate 4° minute-1 at 2θ = 25.20, 32.60, 41.66, 51.74, 58.18, 62.51, 64.40, 68.30, 74.07 corresponded to (001), (100), (101), (002), (110), (102), (111), (200) and (201) of ZrB2. While the other diffraction peaks were with scanning rate 4° minute−1 at 2θ = 21.82, 26.99, 27.68, 35.02, 46.89, corresponded to (001), (170), (200), (171) and (112) of Zr7O9F10. Experimental samples peaks broaden with the increase of temperature which showed that the experimental samples had the strong nano-meteric effect (Figure 3).
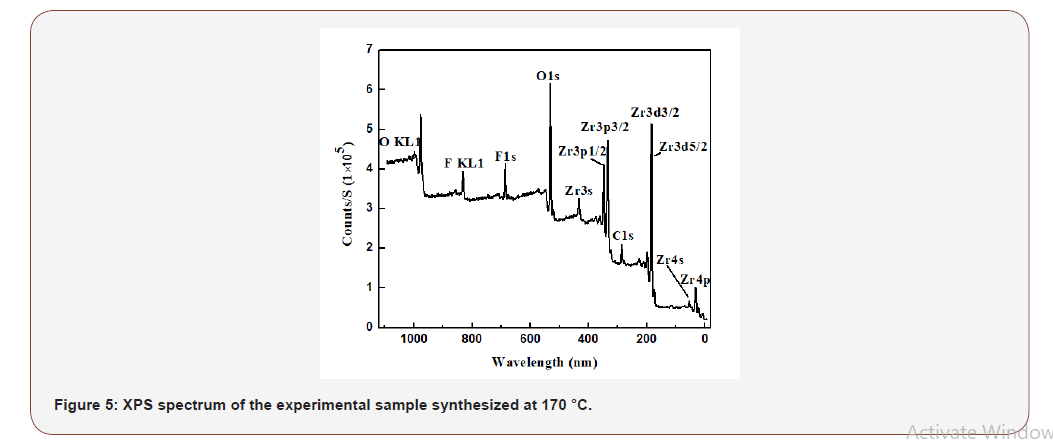
From Figure 4, of high-resolution transmission electron microscopy (HRTEM) was conducted to the experimental sample synthesized at 170 °C to understand the crystal structure and growth process. The distance between the lattice planes perpendicular to nanosheet was 0.328 nm, which corresponded to (200) d-space of Zr7O9F10 (JCPDS Card No. 27- 0998). The space distance along the nano-sheet was 0.405 nm, which corresponded to (001) d-space of Zr7O9F10 as shown in Fig 4a. The projected periodic growth of the crystal structure was along the (001) direction. Urchin and nanowall like structures were hetero-structure and the outer shell was of Zr7O9F10 and inner core of ZrB2 (Figure 4).
From Fig 5, X-ray photoelectron spectroscopy (XPS) was conducted to the experimental sample synthesized at 170 °C. The XPS spectrum showed that Zr atom had two binding energies +2 and +4 oxidation state. The first binding energy was 182 ± 0.47 eV of Zr3d3/2 and second binding energy was 176 ± 0.47 eV of Zr3d5/2. The biding energy of F1s was 684 ± 0.47 eV and the biding energy of O1s was 530 ± 0.47 eV. The B1s valence state was absent in the spectrum because ZrB2 was nearly embedded by the Zr7O9F10 nanosheets. XPS cannot detect the B element from inner ZrB2 as XPS is a surface analytic apparatus (Figure 5).
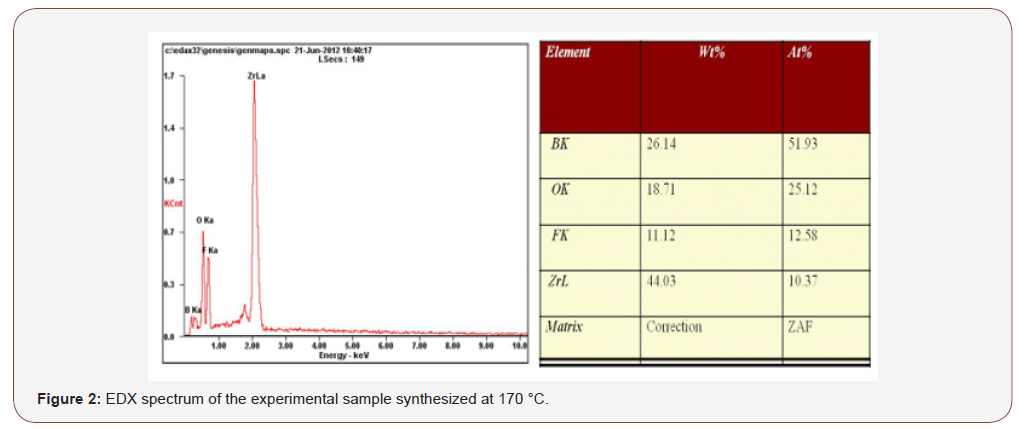
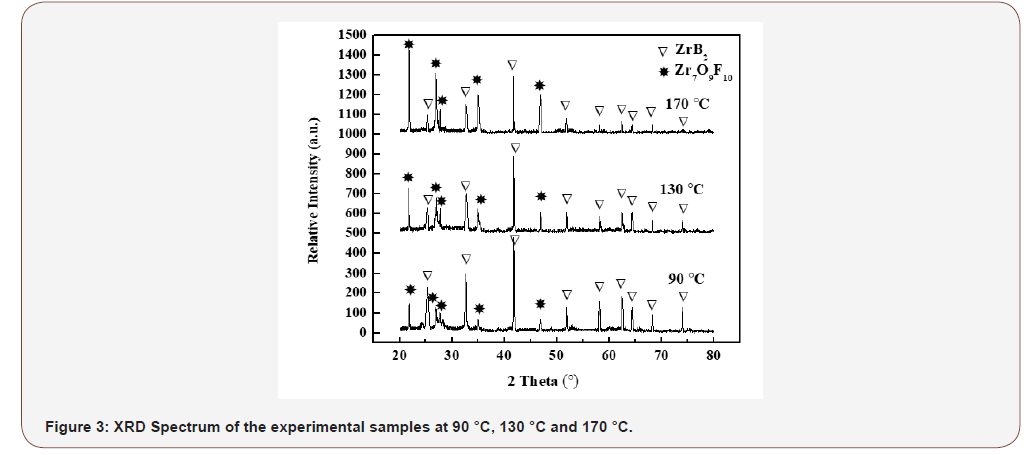
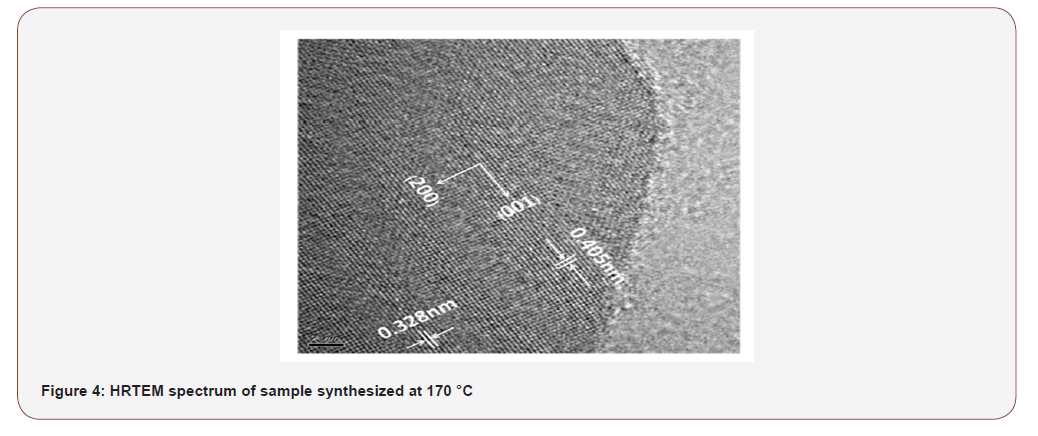
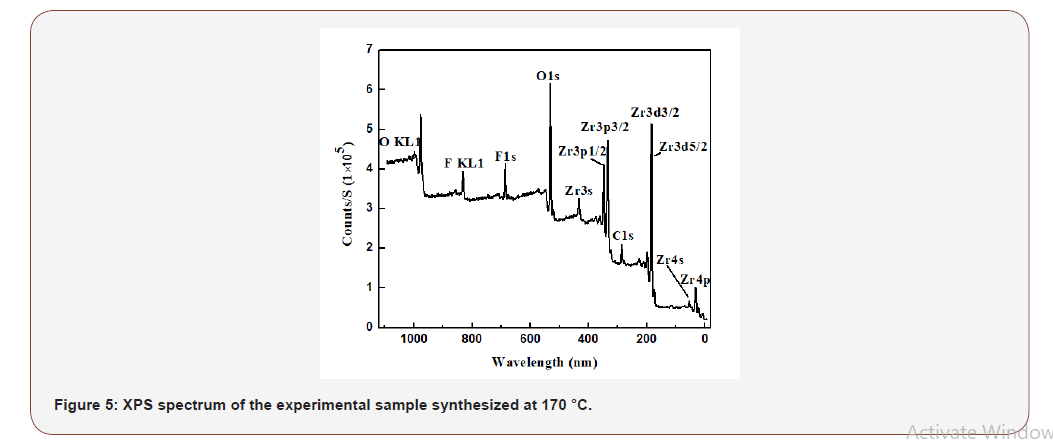
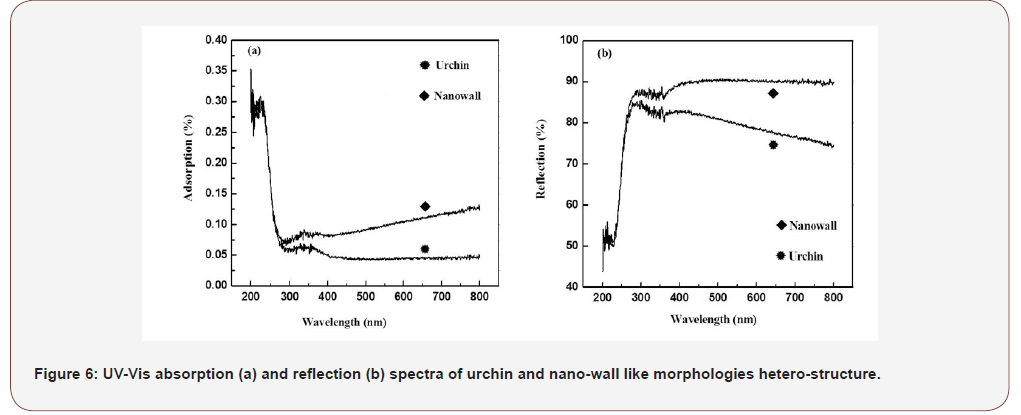
From Figure 6, uv-vis absorption and reflection spectra for (Zr7O9F10/ZrB2) hetero-structure at different temperatures are shown in Figure 6. Hetero-structure had a wide absorption and reflection bands from 230 nm to 290 nm in middle ultra-violet range. Absorption edge of the nano-wall and urchin like hetero-structure compared at higher temperature revealed that the absorption edge for nano-wall sample was red shifted 45 nm. Structural evolution of hetero-structure illustrated above has remarkable changes in the optical properties. UV-Vis spectrum revealed that the heterostructure (Zr7O9F10/ZrB2) was photocatalyst material (Figure 6).
Conclusion
The zirconium oxide fluoride and zirconium diboride heterostructure (Zr7O9F10/ZrB2) material have been successfully synthesized under controlled conditions of temperature by facile hydrothermal method and incomplete reaction mechanism. The zirconium oxide fluoride and zirconium diboride hetero-structure (Zr7O9F10/ZrB2) materials combine the properties of each unit and provide a great deal of opportunity to explore properties. Heterostructure has remarkable changes in the optical properties. UV-Vis spectrum revealed that the hetero-structure (Zr7O9F10/ZrB2) was the photocatalyst material.
Acknowledgements
This work was supported by the National Natural Science Foundation of China (NSFC 51402111 and 21528301), and the Fundamental Research Funds for the Central Universities (SCUT Grant No. 2018ZD21).
Conflict of Interest
No conflict of interest.
References
- JG Song, LM Zhang, JG Li, J RSong, J Disper (2007) Sci Tech 28: 1072-1076.
- IB Bankovskaya, MP Semov, AE Lapshin (2005) Glass Phys Chem 31: 433- 438.
- JG Song, JG Li, JR Song, LM Zhang (2007) Mater Manuf Process 23: 475-478.
- D Sciti, S Guicciardi, A Bellosi (2006) J Am Ceram Soc 89: 2320-2322.
- Z Wang, B Huang, Y Dai, X Qin, X Zhang, et al. (2009) J Phys Chem C 113: 4612.
- X Zhang, L Zhang, T Xie, D Wang (2009) J Phys Chem C 113: 7371.
- Z Liu, DD Sun, P Guo, JO Leckie (2007) Nano Lett 7: 1081.
- KS Song, MK Park, YT Kwon, HW Lee, WJ Chung, e al. (2001) ChemMater 13: 2349.
- R Ostermann, D Li, Y Yin, JT McCann, Y Xia (2006) Nano Lett 6: 1297.
- AK Kuriakose, JL Margrave (1964) J Electrochem Soc 111: 827-831.
- WC Tripp, HC Graham (1971) J Electrochem Soc 118: 1195-1199.
- JB Berkowitz-Mattuck (1966) J Electrochem Soc 113: 908-914.
- RJ Irving, IGWorsley (1968) J Less-Common Met 16: 103-112.
- F Monteverde, A Bellosi (2003) J Electrochem Soc 150: B552-B559.
- D Sciti, M Brach, A Bellosi (2005) Scripta Mater 53: 1297-1302.
- A Rezaie, WG Fahrenholtz, GE Hilmas (2006) J Am Ceram Soc 89: 3240-3245.
- WG Fahrenholtz (2007) J Am Ceram Soc 90: 143-148.
- A Rezaie, WG Fahrenholtz, GE Hilmas (2007) J Eur Ceram Soc 27: 2495-2501.
- TA Parthasarathy, RA Rapp, M Opeka, RJ Kerans (2007) Acta Mater 55: 5999-6010.
- Jones M, Kumar S, Lo SS, Scholes GD (2008) J Phys Chem C 112: 5423-31.
- Gautam UK, Fang X, Bando Y, Zhan J, Golberg D (2008) ACS Nano 2: 1015-1021.
- F Huang, Z Fu, A Yan, W Wang, H Wang, et al. (2009) CrystGrowth Des 9: 4017-4022.
- F Huang, Z Fu, A Yan, W Wang, H Wang, et al. (2009) Mater Lett 63: 2655-2688.
-
Shahzad Ahmad K, Ligui L, Dengke Z, Shaowei C. New Hybrid Hetero-Structure (Zr7O9F10/ZrB2) Ultraviolet (UV) Photo-Catalyst synthesized by Hydrothermal Method. Insi in Org & Inorg. 1(2): 2020. IOIC. MS.ID.000506.
-
Spectrofluorımetric, Antı-Hypertensıve Drugs, Pharmaceutıcal preparations, Chemistry, fluorescence intensity, Pharmaceutical additives, Benazepril HCl, Hydrochlorothiazide, Spectrofuorimetry
-
This work is licensed under a Creative Commons Attribution-NonCommercial 4.0 International License.