Short Communication
Derivation of the pH-Dependent Higuchi Equation
Nashiour Rohman1*, Tariq Mohiuddin1, Khalid Ahmed2, Imran Khan3, and Adam A Skelton4
1Department of Physics, College of Science, Sultan Qaboos University, P. O. Box 36, Al-khoudh, Muscat P. C. 123, Oman
2L. E. J. Nanotechnology Centre, H. E. J. Research Institute of Chemistry, International Centre for Chemical and Biological Sciences, University of Karachi, Karachi – 75270, Pakistan
3Department of Chemistry, College of Science, Sultan Qaboos University, P. O. Box 36, Al-khoudh, Muscat P. C. 123, Oman
4Department of Pharmaceutical Sciences, University of KwaZulu-Natal, Durban 4000, South Africa
Nashiour Rohman, Department of Physics, College of Science, Sultan Qaboos University, P. O. Box 36, Al-khoudh, Muscat P. C. 123, Oman.
Received Date: December 12, 2022; Published Date: December 16, 2022
Abstract
We have converted the diffusion-controlled Higuchi equation to pH-dependent form in this paper. We have modified the original Higuchi equation to produce the pH-dependent variant by incorporating the Nernst-Planck equation into Flick’s first law. A time-dependent medication particle delivery from a silica matrix might be predicted using the modified equation.
Keywords: Higuchi equation; Flick’s first law; Nernst-Plank equation; Drug delivery; pH-dependent
Introduction
Since Higuchi [1] deduced the drug delivery rate for the first time in 1961, the application of mathematical modelling to create regulated and sophisticated drug delivery systems has opened up new avenues in the field of pharmaceutical science [2-4]. In recent years, this paradigm has been the subject of extensive research [1, 5-13]. The model accounts for the excess loading of a drug particle into a carrier matrix above its solubility limit. Flick’s first law states that due to the gradient in concentration, when the assembly is dissolved into the fluid, which is regarded as a sink, the drug particles are drastically released into the surrounding fluid [14]. According to Higuchi’s theory, which is illustrated in Figure 1 and known as the ‘moving boundary approach’, [15] the boundary of the concentration gradient moves in the opposite direction of the stream of drug particles. The speed at which the gradient’s concentration boundary moves determines how quickly drug particles migrate. Higuchi arrived at the following two equations based on this theory [1]:
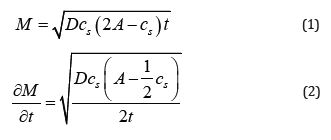
In Eqs (1) and (2), A represents the surface area of the matrix, c0 and cs represent the initial and saturated concentrations of solute drug particles in the matrix, and D represents the diffusion of particles in the matrix. M represents the cumulative mass of solute accumulated in the surrounding fluid in time from A ‘s surface area. Here, we attempted to derive the pH-dependent variant of the Higuchi equation. The resulting equation will be helpful to examine experimental findings of pH-dependent drug loading and release by carrier matrix (Figure 1).
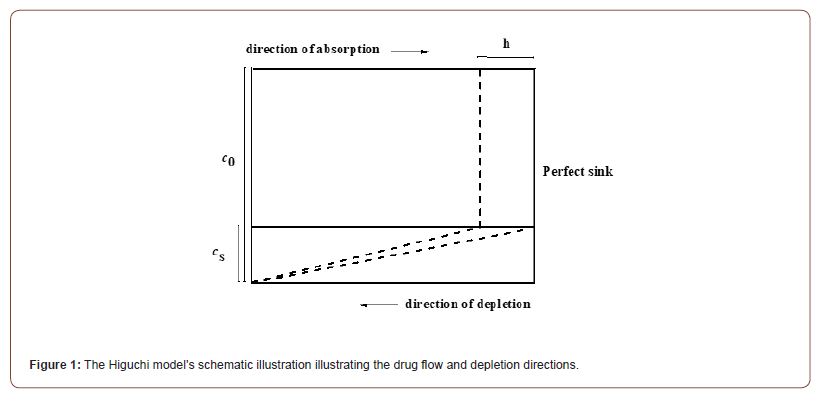
Derivation
The following eq (3) is applicable if A is the area, M is the total mass of drug particles accumulated over time t, co, is the starting drug concentration in the matrix, and cs is the maximum quantity of drug that can be dissolved in the matrix.
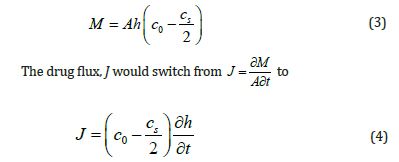
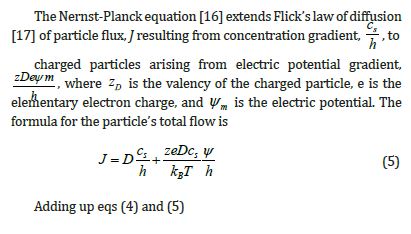
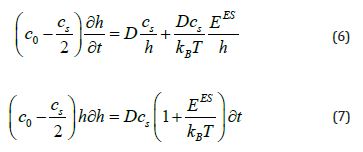
When eq (7) is integrated, the result is
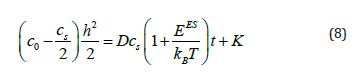
where K is the integration constant. Applying the boundary condition results in i.e., whent t = 0, h = 0.
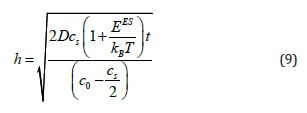
and K = 0. The result of substituting the h value from eq (9) into eq (8) is
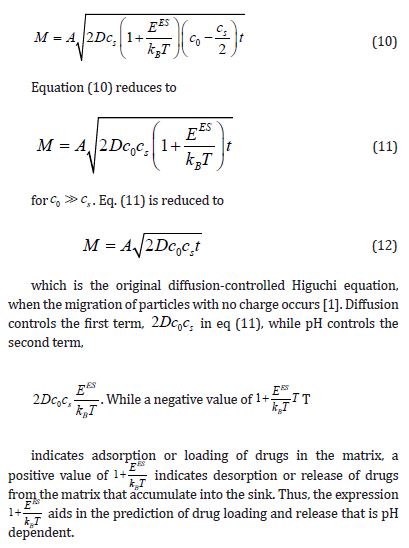
Conclusion
The diffusion-regulated Higuchi equation has been derived to result in pH-dependent medication release from drug carrier. This is achieved by include the potential gradient migration of the Nernst-Planck equation in the Higuchi model. This equation will be helpful for a qualitative preliminary evaluation of pH-dependent medication release from a carrier. We are currently working to confirm the proposed equation with experimental data.
Conflict of Interest
No conflicts of interest are disclosed by the authors.
Acknowledgement
This work was granted by College of Health Sciences (CHS), UKZN, Durban, South Africa and Grant No. SR/SCI/PHYS/18/01, Sultan Qaboos University, Oman.
References
- T Higuchi (1961) Rate of release of medicaments from ointment bases containing drugs in suspension. J Pharm Sci 50: 874-875.
- N Rohman, T Mohiuddin, K Ahmed, I Khan, S Rajkhowa, et al. (2022) Theoretical modelling of electrostatic interactions in pH-dependent drug loading and releasing by functionalized mesoporous silica nanoparticles, Chemical Papers.
- K Ahmed, SN Inamdar, N Rohman, AA Skelton (2021) Acidity constant and DFT-based modelling of pH-responsive alendronate loading and releasing on propylamine-modified silica surface, Physical Chemistry Chemical Physics 23: 2015-2024.
- SN Inamdar, K Ahmed, N Rohman, AA Skelton (2018) Novel pKa/DFT-Based Theoretical Model for Predicting the Drug Loading and Release of a pH-Responsive Drug Delivery System, The Journal of Physical Chemistry C 122: 12279-12290.
- L Wu, CS Braze (2008) Mathematical Model to Predict Drug Release, Including the Early-Time Burst Effect, from Swellable Homogeneous Hydrogels.
- A. Peppas (2013) Historical perspective on advanced drug delivery: How engineering design and mathematical modeling helped the field mature, Advanced Drug Delivery Reviews 65: 5.
- G Pontrelli, G Toniolo, S McGinty, D Peri, S Succi, et al. (2021) Mathematical modelling of drug delivery from pH-responsive nanocontainers, Computers in biology and medicine 131: 104238.
- J Siepmann, NA Peppas (2011) Higuchi equation: Derivation, applications, use and misuse, International Journal of Pharmaceutics 418: 7.
- GL Flynn (1976) Analysis of Diffusion through Concentric Right Circular Cylinders and Concentric Spheres.
- DR Paul (2011) Elaborations on the Higuchi model for drug delivery. Int J Pharm 418: 13-17.
- R. Paul (1985) Modeling of solute release from laminated matrices, Journal of Membrane Science 23: 221-235.
- JH Petropoulos, KG Papadokostaki, M Sanopoulou (2012) Higuchi's equation and beyond: overview of the formulation and application of a generalized model of drug release from polymeric matrices, Int J Pharm 437: 178-191.
- J Siepmann, F Siepmann (2012) Modeling of diffusion controlled drug delivery, Journal of Controlled Release 161: 351-362.
- A Fick (1995) On liquid diffusion, Journal of Membrane Science 100: 33-38.
- KM Moroney, L Kotamarthy, I Muthancheri, R Ramachandran, M Vynnycky (2021) A moving-boundary model of dissolution from binary drug-excipient granules incorporating microstructure, International Journal of Pharmaceutics 599: 120219.
- RF Probstein (1989) 2 - Transport in Fluids, in: R.F. Probstein (Ed.) Physicochemical Hydrodynamics, Butterworth-Heinemann: pp. 9-30.
- A Fick (1855) V On liquid diffusion, The London, Edinburgh, and Dublin Philosophical Magazine and Journal of Science 10: 30-39.
-
Nashiour Rohman*, Tariq Mohiuddin, Khalid Ahmed, Imran Khan and Adam A Skelton. Derivation of the pH-Dependent Higuchi Equation. Insi in Chem & Biochem. 2(3): 2022. ICBC. MS.ID.000538.
-
General Chemistry& Chemical technology, Quaternary ammonium salt; rearrangement-cleavage reaction; ylid; alkylation-elimination reaction; chalcone; thermal; alkylation.
-
This work is licensed under a Creative Commons Attribution-NonCommercial 4.0 International License.