Research Article
An Amide Derivative of Betulonic Acid as a New Inhibitor of Sars-CoV-2 Spike Protein-Mediated Cell Entry and Sars-CoV-2 Infection
Cheresiz SV1*, Kononona AA1, Skarnovich M2, Volkova AN3, Poletaeva Yu A1, Emaminia F1, Pyankov OV2, Schultz EE3 and Pokrovsky AG1
1Zelman`s School of Medicine and Psychology, Novosibirsk State University, Russia
2Vector State Research Center of Virology and Molecular Biology, Russia
3N N Vorozhtsov Novosibirsk Institute of Organic Chemistry, Siberian Branch of the Russian Academy of Science, Russia
Sergey V Cheresiz, Zelman`s School of Medicine and Psychology, Novosibirsk State University, 630090, Pirogova st., 1, Novosibirsk, Russia.
Received Date: October 15, 2022; Published Date: November 28, 2022
Abstract
Betulin (B), betulinic and betulonic acids (BAs) are the pentacyclic lupine triterpens, which possess an array of biological activities making them potential therapies for cardiovascular, inflammatory, neurological disorders, and cancer. They demonstrate a range of antiviral activities with different mechanisms, which aim different pharmacological targets. The COVID-19 pandemics showed the lack of specific treatments against SARSCoV- 2 and posed a challenge of repurporsing known antiviral drugs, including natural product, into potent coronavirus inhibitors. Two main SARSCoV- 2 targets promising for inhibition are the main protease involved in virion maturation, and the spike protein-mediated cell entry involving spike-receptor interactions. Entry inhibitors possess several advantages: easily accessibile extracellular targets, a non-overlapping resistance mutation spectra with other groups of antivirals, and others. A number of SARS-CoV-2 entry inhibitors were identified in silico and in vitro, the latter approach involving pseudotype entry inhibition assay and the suppression of SARS-CoV-2 replication, both also used in our research. BA derivatives inhibit HIV-1 and HSV-1 cell entry, but their activity against SARS-CoV-2 entry is unknown. Structural and in silico studies predict strong binding sites for BAs and betulin in the structure of SARS-CoV-2 spike protein. Here, we report a betulоnic acid amide derivative with inhibitory activity on SARS-CoV-2 replication. Using a replication-deficient vesicular stomatitis virus pseudotype, we show that SARS-CoV-2 spike-protein-mediated cell entry is inhibited.
Keywords: Betulinic acid; SARS-CoV-2; Pseudotype; Virus entry; Antivirals
Abbreviations: ACE2: Angiotensin-Converting Enzyme 2; B: Betulin; BA: Betulinic Acid; BSL-3: Biosafety Level 3; COVID-19: Coronaviral Disease 19; CPE: Cytopathic Index; ECHO-6: Enteric Cytopathic Human Orphan virus 6; DMSO: Dimethyl Sulphoxide; FACS: Fluorescence-Activated Cell Sorting; FDA: Federal Drug Administration; GFP: Green Fluorescent Protein; ELISA: Enzyme-Linked Immunosorbent Assay; HIV-1: Human Immunodeficiency Virus 1; HSV-1: Herpes Simplex Virus 1; HSV-2: Herpes Simplex Virus 2; IC50: Inhibitory Concentration 50%; IL-1β - Interleukin- 1β; IL-6: Interleukin-6; MeOH – Methanol; MERS-CoV – Middle-East Respiratory Syndrom Coronavirus; MTT: 3-(4,5-dimethylthiazol-2-yl)-2,5- Diphenyltetrazolium Bromide; RAS: Russian Academy of Sciences; RBD: Receptor-Binding Domain; ROS: Reactive Oxygen Species; rVSIV: Vesicular Stomatitis Indiana Virus; RSV: Respiratory Syncityal Virus; SARS-CoV-2: Severe Acute Respiratory Syndrom Coronavirus 2; SREBP: Sterol Regulatory Element-Binding Protein; SI: Selectivity Index; TC50 - Toxic Concentration 50%; THF: Tetrahydrofuran; TNF-α: Tumor Necrosis Factor-α; VSIV: Vesicular Stomatitis Indiana Virus
Introduction
Betulin (B) 1 and betulinic acid (BA) 2 are the pentacyclic lupinetype triterpens, which represent the widely distributed secondary metabolites found in different medicinal plants, mainly, belonging to the Betulaceae family and possessing an array of biological activities. A number of studies has reported the efficiency of BA and its derivatives as the therapies for cardiovascular, inflammatory, and neurological disorders, and was considered as an efficient anticancer drug for some types of cancer due to its ability to induce multiple apoptosis pathways [1,2,3]. It was extensively reported to be a selective agent inducing cell death in tumor, but not normal cells [4] – an opinion, which might now have to be somehow readdressed [5,6,7].
Betulin (B) 1, betulinic acid (BA) 2 and their derivatives demonstrate a range of activities against unrelated viruses, which rely upon different mechanisms and are aiming at different pharmacological targets. Their anti-HIV activities are best documented, with representatives found in several classes of antiretroviral drugs [8,9,10], although acting with varying potency. The most potent anti-HIV BA and B derivatives inhibit virus maturation by blocking the cleavage of the capsid-spacer (CA/p2) peptide of the HIV-1 Gag polyprotein [11]. Betulinic acid and its various derivatives including C-28 amides, a number of the 2,3-dehydrobetulin and 2,3-dehydrobetulinic acid derivatives, as well as bevirimat, the C-3 BA ester of 2,2-dimethylsuccinic acid, which reached Phase II clinical trials, all belong to this group of action [12]. The most powerful inhibitors of this group have IC50 within nanomolar range [8]. Other BA derivatives, such as its glutaryl hemiester, may affect the HIV-1 maturation by inhibiting the viral protease, possibly, via blocking its dimerization, although with rather modest potency (IC50~5mM) [13].
Another group of BA derivatives has earlier been identified as quite potent HIV entry inhibitors. IC9564 (4S-[8-(28 betuliniyl) aminooctanoylamino]-3R-hydroxy-6-methylheptanoic acid) [14] and its stereoisomer, RPR103611 [15] block the HIV-1 envelopemediated membrane fusion at nanomolar range. The first compound appears to target gp120 surface subunit of the HIV-1 Env, while the second one, reportedly, targets gp41 transmembrane subunit or the interaction between gp120 and gp41, but further studies are needed to elucidate their precise mechanism of action [13]. Recently, the HIV-1 integrase inhibition by the ethanol extracts of Betula alnoides, which contain five pentacyclic triterpenoids including betulin and betulinic acid, has been reported. Upon chromatographic fractionation, the purified B and BA showed good inhibition of the HIV-1 integrase in vitro with the IC50 values of 17.7 and 24.8 mM, respectively [10]. While all above mentioned BAderived HIV-1 inhibitors had little effect on the HIV-2 replication, a shorter side chain at C-28 position of (n=5) and a carboxylic acid terminus of this chain confer the anti-HIV-2 entry inhibition activity, apparently, via targeting the V3 loop of the HIV-2 gp120 [16].
Apart from the HIV, the antiviral effect of betulin, betulinic and betulonic acids against herpesviruses (HSV-1) has been shown [17], while a later study, which involved time-of-addition experiments, pointed at a peculiar, yet, not elucidated mechanism of inhibition of the earliest steps of HSV-1 infection, before virus adsorption and penetration and, probably, related to a direct drugvirus interaction, since only pretreatment of virus prior to the infection has been efficient [18]. Meanwhile, some ionic derivatives obtained to improve BA solubility exhibited antiviral effect against another herpes simplex virus, HSV-2, with IC50 values comparable or superior to acyclovir (0.6-7.2 mM vs. 0.1-10.0 mM) [19].
Betulinic acid, as well as two closely related triterpenes isolated from a Chinese medicinal plant, Schefflera heptaphylla (3alpha-hydroxylup-20(29)-ene-23,28-dioic acid and 3-epibetulinic acid 3-O-sulfate), showed potent inhibition of different influenza A/H1N1 virus strains in cultured cell lines [20,21,11]. However, B and BA proved inefficient against another influenza A virus subtype, H7N1 (A/FPV/Rostock/34 strain), while betulonic acid showed weak antiviral activity [17]. The 3-oximation or the C-28 carboxyl substitutution improve the anti-influenza effect of betulonic acid [22] (Baltina et al., 2003), and its ureido derivatives also demonstrate, though rather weak, influenza A virus inhibition [23]. Also, isolated studies report the antiviral activity of BA and the related substances against ECHO-6 virus [17], respiratory syncitial virus (RSV), Coxsackie B3 (Cox B3) virus [20], papilloma viruses [24], hepatitis C [25] and B [26] viruses.
The current global outbreak of COVID-19 disease caused by SARS-CoV-2 showed the lack of specific treatment against this beta-coronavirus and posed a challenge to test the compounds with known antiviral activities, including those derived from natural products, and repurpose them into potent and low-toxic coronavirus inhibitors. Several lines of research and design of such inhibitors are being currently pursued.
The first line is aiming at the inhibition of SARS-CoV-2 main protease, 3CL, involved in virion maturation due to its proteolytic processing of two viral polyproteins, pp1a and pp1ab. The resolution of crystal structure of SARS-CoV-2 3CLPRO revealed the high degree of structural similarity with 3CL protease of the first beta-coronavirus causing respiratory distress syndrome, SARS-CoV, with which it shares 96% homology and differs in only 12 amino acids [27,28]. An in silico approach combining virtual screening, docking and molecular dynamics revealed a striking similarity of ligand binding to SARS-CoV and SARS-CoV2 main proteases [29], and, thus, the inhibitors of the former are expected to efficiently inhibit the latter.
Betulinic acid was found to be one of the most potent SARSCoV 3CL protease inhibitors out of 221 herbal compounds screened [30] with a proposed inhibition mechanism involving formation of hydrogen bonds between BA and the amino acids at the active site of a pocket of protease enzyme [31]. Several in silico studies of BA-related compound binding to SARS-CoV-2 3CL protease reveal good binding energies of BA to the structure pocket of 3CL protease [32,33]. The latter study also provides an in vitro confirmation of a significant protease inhibition by BA, as compared to betulin (IC50=3.22-14.55 mM vs. 89.67 mM), and points at the significance of C-17 carboxylate moiety and C-3 m-hydroxyl group as essential features for 3CL binding and inhibition. Surprisingly, another in silico study reports an unfavorable BA binding to SARS-CoV 3CL protease [34].
Recently, BA-related compound from C. xylocarpa, betulonal, previously known as an anti-HIV compound, was identified in silico as a natural molecule with high-affinity binding to two SARS-CoV-2 targets, 3CL protease and RNA-dependent RNA polymerase [35]. The next direction investigates the potential of BA and its derivatives as SARS-CoV-2 entry inhibitors acting mainly by disrupting the interaction of the viral spike protein S with its cognate cellular receptor, ACE2. A virtual screen of an FDA-approved drug library for the compounds able to inhibit interaction of S1 subunit RBD (receptor-binding domain) with ACE2 identified six pockets in the 3D structure of S1 RBD, which can interfere with ACE2 binding either directly or via an allosteric mechanism upon drug binding and which are conserved with respect to SARS-CoV sequence. BA and betulin have shown selective binding to one of these pockets in silico, while BA proved to significantly inhibit ACE2:SARS-CoV-2 Spike interactions in vitro in submicromolar concentrations (0.1 mM) [36]. An in silico study of terpenoids from Chaga medicinal mushroom demonstrated that BA can stably bind Spike protein near the ACE2-binding site [37], while docking experiments performed in another study showed that BA can preferentially bind to sites different from the RBD [34]. Yet another activity of BA-related molecules, which can make them the potential anticoronavirus drugs, is the inhibition of a cellular target, SREBP (sterol regulatory element-binding protein) and the downstream fatty acid synthesis [38], which blocks the formation of doublemembrane vesicles, the specific organelles serving as the factories for coronavirus replication [39]. Betulin, which inhibits SREBP cleavage and maturation, can effectively reduce the production of a related coronavirus, MERS-CoV, in the infected cells [38]. Here, we report a betulоnic acid amide derivative, which shows inhibitory activity on SARS-CoV-2 replication in Vero E6 cells. Using a replication-deficient vesicular stomatitis virus (VSIV), we show that SARS-CoV-2 spike-protein-mediated cell entry inhibition is the mechanism responsible for the anti-coronavirus activity of the described betulonic acid derivative. We further plan to synthesize a series of BA-related compound starting from BA8 as a prototype molecule and to more precisely dissect the entry inhibition mechanism using the time-of-addition experiments [40-46].
Materials and Methods
Synthesis of BA derivatives and stock solution preparation
Вetulonic acid 3 and five derivatives, of three amides 4 (further referred to in the paper as BA-2m, BA7, and BA8), and two dipeptides 5,6 (DP1 and DP82f) were synthesized following the schemes published in [47-49]. The starting material for preparing betulonic acid was betulin 1, which was isolated from birch bark by method described in [50]. Chemical structures of the compounds of this BA series are shown in Figure 1.
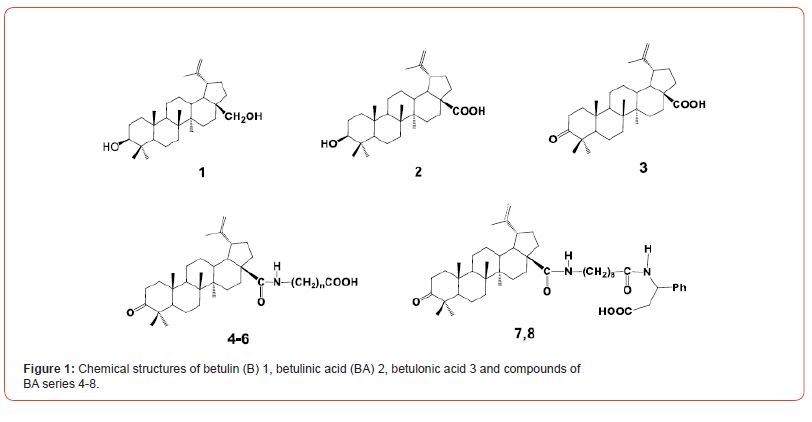
To prepare stock compounds, every dry compound was dissolved in sterile cell culture grade DMSO to the concentration of 10 mM, aliquoted and stored at -20oC.
Cells, viruses and plasmids
Vero E6 cells used as the targets for infection with SARSCoV- 2 infectious isolate were grown in MEM medium (Gibco, USA) supplemented with 10% calf serum (Gibco, USA). 293FT cells used as the producer cells for virus pseudotypes and as targets for pseudotype infection (when transfected with an ACE2-expressing plasmid, see the corresponding portion of Materials and Methods for details) were grown in DMEM/F12 medium (Clontech, USA) supplemented with 10% calf serum (Clontech, USA) and penicillin/ streptomycin.
SARS-CoV-2hCoV-19/Russia/Vologda-171613-1208/2020 isolate used in experiments on infection inhibition in Vero E6 cells was obtained from the collection of Vector State Research Center of Virology and Molecular Biology and passaged 3 times in Vero E6 cells prior to the infection experiments.
rVSV/SARS-CoV-2 S and rVSV/VSV-G pseudotypes based on the capsid of recombinant vesicular stomatitis virus and used for the entry inhibition studies are described in a separate portion of Materials and Methods.
pGBW-m4137382 plasmid for the expression of SARS-CoV-2 Spike protein with a 19 a.a. truncation of transmembrane domain and pLP-VSVG plasmid for the expression of VSV G glycoprotein, which were used to transfect virus pseudotype producer cells are from Addgene repository. pUCHR-hACE2 plasmid for the expression of ACE2 receptor used for the transfection of virus pseudotype target 293FT cells was kindly provided by Dr.D.Mazurov (Institute of Gene Biology, RAS, Moscow) [51]. peGFP-N1 plasmid used to trace the transfection efficiency was purchased from Clontech.
Colorimetric assay of cell metabolic activity/viability (MTT test)
MTT test was used to determine the cytotoxicity of different concentrations of BA derivatives to target cells and to evaluate the degree of cytopathic effect (CPE) induced by SARS-CoV-2 isolate in Vero E6 cells. Briefly, at the end of cytotoxicity tests or SARS-CoV-2 infection experiments, tetrazolium dye MTT (3-(4,5-dimethylthiazol-2-yl)-2,5-diphenyltetrazolium bromide) was added to the cells. After 2-4 h of incubation, the produced formazan crystals were dissolved in DMSO or HCl/SDS, and the absorbance was measured using a designated plate reader, at 450 nm and 570 nm for DMSO and HCl/SDS, respectively.
Inhibition of SARS-CoV-2 infection by BA derivatives
Vero E6 cells used as the targets for infection with SARS-CoV-2 hCoV-19/Russia/Vologda-171613-1208/2020 isolate were split in 96-well plates one day before the infection and preincubated for 2 h in 100 mL of BA derivatives at different concentrations (100, 20, 4.0, 0.8 and 0.016 mM). Four or six wells (quadruplicates or hexaplicates) were used for each concentration of each BA derivative. Remdesevir taken at the same concentrations was used as a reference SARSCoV- 2 inhibitory compound. After preincubation, 100 mL of virus were added to the wells, and the plates were incubated for 3 days at 37oC and 5% CO2. Then, the plates were first visually inspected under the microscope to ensure that CPE in positive control wells containing the virus without inhibitors reached 85-100%. After that, the MTT test was performed, as described above.
After the absorbance has been measured, TC50 (50% toxic concentration, at which 50% of non-infected cells remain viable) and IC50 (50% inhibitory concentration, at which 50% of infected cells remain viable) were calculated using SoftMaxPro-4.0 software. Then, selectivity index (SI) was calculated for each compound using the following formula – SI= TC50/IC50. The compounds possessing SI > 8 – 10 are considered active, those with SI from 2 to 8 are considered to have moderate antiviral activity, while those with SI below 2 were counted as inactive ones [52,53].
All experiments with infectious SARS-CoV-2 isolate were performed at Vector State Research Center of Virology and Molecular Biology BSL-3 facility.
Production of rVSV pseudotypes for entry inhibition assay
rVSV/SARS-CoV-2 S and rVSV/VSV-G pseudotypes based on the capsid of replication-deficient recombinant vesicular stomatitis virus (rVSV) and used for the entry inhibition studies were produced, as published elsewhere. Briefly, the pseudotype producer 293FT cells were co-transfected with the plasmids for viral glycoprotein expression (pGBW-m4137382 for rVSV/SARSCoV- 2 S and pLP-VSVG for rVSV/VSVG) and peGFP-N1 plasmid to trace the transfection efficiency in 100 mm plates using Fugene HD transfectant. As much, as 10 mg of pGBW-m4137382 or 0.5 mg of pLP-VSVG were used per 100 mm dish, tracer plasmid was always used at 0.5 mg per dish. 48 h post transfection, the monolayers of producer cells expressing viral glycoproteins on their surface were infected by the stock rVSV/VSV-G pseudotype (kindly provided by Professor Robert Davey of National Emerging Infectious Diseases Laboratories (NEIDL), USA), and the virus pseudotypes secreted into growth medium were collected 48 h post infection. Pseudotypes were cleared by low-speed centrifugation (3000 rpm x 5`) to get rid of cell debris, aliquoted and stored at -80o. rVSV/ SARS-CoV-2 S pseudotype was used to study the inhibition of spike protein-mediated cell entry, while rVSV/VSV-G pseudotypes by BA derivatives. rVSV/VSV-G pseudotypes were used in the same assays to confirm the specificity of pseudotype entry inhibition. A specific inhibitor of SARS-CoV-2 S-mediated entry should inhibit the infection by rVSV/SARS-CoV-2 S, but not the rVSV/VSV-G pseudotypes.
Pseudotype entry inhibition assay
The target cells for the pseudotype entry inhibition assay were obtained by the transfection of 293FT cells split in 96 well plates by 0.1 mg of pUCHR-hACE2 plasmid per well to express the cellular ACE2 receptor of SARS-CoV-2 entry. Also, 0.01 mg of peGFP-N1 plasmid per well was transfected to evaluate the efficiency of cotransfection, which was determined 24 h later, before pseudotype infection, by detaching the transfected cells in a test well and counting the percentage of GFP-positive cells by FACS using BD Accuri C6 instrument. The cytotoxicities of studied BA-derived inhibitors exerted on such ACE2-transfected 293FT cells were also measured using MTT test before setting up the pseudotype infection.
24 h post transfection, the target cells were preincubated for 2 h with different concentrations of BA-derived inhibitors and then infected in triplicates (3 wells) by either rVSV/SARS-CoV-2 S, or the rVSV/VSV-G pseudotypes. The signal of the transduced rVSV reporter gene, firefly luciferase, was measured in the infected 293FT/ACE2 cells 24 h post infection using designated plate-reader format luminometer. The degree of pseudotype entry inhibition by different BA-derived inhibitor concentrations was calculated as the ratio of luminescences of inhibited to the non-inhibited wells. Statistical analysis was performed using Excel statistical software.
Results
Synthesis and description of BA derivatives
The synthesis of 4,5 involves the reaction of betulonic acid chloride with methyl esters of ω-amino acids or methyl N-(9- aminononanoyl)-3-amino-3-phenylpropionate hydrochloride in CH2Cl2 in the presence of Et3N and subsequent hydrolysis of the esters by NaOH in THF and MeOH. Hydrogenation of compound 5 gave 6. One of the dipeptide of betulonic acid, exhibited high antiviral activity and acts as a powerful immunostimulating agents [40].
All chemical structures of the compounds of this BA series are shown in Figure 1.
10 mM stock solutions of BA derivatives in DMSO were prepared and stored at -20oC. The dilutions of BA-related compounds in growth medium were prepared and used immediately.
Cytotoxicities and Inhibition of SARS-CoV-2 infection by BA derivatives
In order to study the cytotoxicities and antiviral properties of the described panel of BA derivatives, all compounds were brought to their working concentrations of 100, 20, 4.0, 0.8, and 0.016 mM by diluting them in growth medium. A ribonucleotide analogue of viral RNA polymerase, remdesivir, known to efficiently block the replication of SARS-CoV-2, was used as a reference inhibitor, and was diluted to the same concentrations, as BA derivatives.
All compounds were added to Vero E6 cells plated in 96-well plates one day before the experiment. The cells were preincubated with the compounds for 2 h before being infected with SARS-CoV-2 hCoV-19/Russia/Vologda-171613-1208/2020 isolate. After 3 days of infection, when an 85-100% cytopathic effect was observed in the positive control wells infected in the absence of inhibitors, MTT was added to the wells, and the absorbance was analyzed 2 h thereafter. The TC50, IC50, and SI values were determined for those substances, for which this was feasible due to their toxicity and antiviral activity profiles. The resulting figures are provided in Tab. 1. Two peptide derivatives, DP1 and DH8.2f, proved to be rather toxic to the non-infected cells (showing TC50 values of 7.0 and 32.21 mM, respectively), while lacking antiviral activity, and, thus, IC50 could not have been calculated. Surprisingly, almost the same toxicity/activity profile was found for the first compound of the series, betulinic acid (TC50 ~7.6 mM and IC50 non-available), which has been previously reported to exhibit toxicity against cancer, but not normal cells [41]. Instead, all amide derivatives (BA 2m, BA7, and BA8) demonstrated lack of toxicity (TC50 > 100 mM), but two of them (BA 2m and BA7) were also lacking virus inhibitory activity (IC50 > 100 mM), and SI value could not have been calculated for these two. The last amide, BA8, had some inhibitory activity on virus-induced CPE ((IC50 ~40.8 mM) and represented a moderate virus inhibitor according to its calculated SI (> 2.45) (Table 1).
Table 1:TC50, IC50, and SI values of BA derivatives and remdesivir in Vero E6 cells.
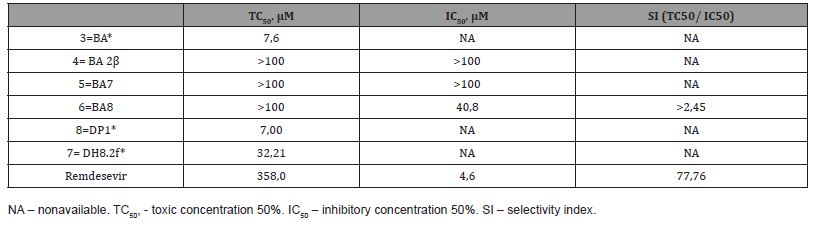
In order to more accurately determine the SI value for the only active compound, BA8, we attempted to determine its TC50 and IC50 in a wider concentration range (>100 mM). To this end, a 3-fold dilution series of 9 BA8 dilutions starting from 500 mM was prepared, and 22.5x103 Vero E6 cells split in 96-well plate in hexaplicates (6 wells) 2 days before the infection were infected by SARS-CoV-2 hCoV-19/Russia/Vologda-171613-1208/2020 at the multiplicity of infection (MOI) equal to 0.1 TCID50/cell in the presence of BA8. Again, remdesevir prepared as a similar dilution series, was used as a reference inhibitor. As one can see from the results shown in Table 2, the precise TC50 value still remained undetermined within this range of concentrations (TC50 > 500 mM), while a refined IC50 obtained in this experiment was 131 IC50 mM. This resulted in SI value of > 3.82 indicating at an apparent, though moderate, inhibition of SARS-CoV-2 infection (Table 2).
Table 2:TC50, IC50, and SI values of BA derivatives and remdesivir in Vero E6 cells.

Pseudotype production and entry inhibition assay
As a next step, we attempted to start dissecting the mechanism of action of BA8 compound and tested its activity against rVSIV/ SARS-CoV-2 S pseudotypes. Pseudotypes are recombinant viruses composed of a capsid of a non-pathogenic virus (in our model, rVSIV with luciferase reporter gene) and expressing viral glycoproteins in their lipid envelope, either homologous or foreign, typically, belonging to the more pathogenic viruses (in our case, SARS-CoV-2 spike protein). These viral pseudotypes have two advantages – they are biologically safe due to rVSV genome modifications, and the inhibition of rVSV/SARS-CoV-2 S pseudotype infection clearly classifies the compound as coronavirus entry inhibitor. To test the specificity of this entry inhibition, a control rVSV/VSV-G pseudotype infection is set up an inhibited by a compound. A specific coronavirus entry inhibitor should suppress the infection by the former, but not the latter pseudotype.
We prepared both pseudotypes by transfecting the monolayer of pseudotype producer 293FT cells with the plasmids expressing the respective viral glycoprotein, and further infected them by stock rVSV/VSV-G pseudotype, as described in the Materials and methods. Virus pseudotypes secreted to the cell growth medium were collected 48 h post infection, cleared by low-speed centrifugation, and their luciferase transduction titer was determined using 293FT cells transfected by an ACE2 receptor expressing plasmid as the targets (data not shown).
The target cells for pseudotype entry inhibition assay were prepared by transient transfection of 293FT cells. Cells split in 96-well plate were co-transfected at 90% confluence with a combination of plasmids, one for the expression of virus entry receptor, ACE2, and another for tracing transfection efficiency by GFP expression. One day post transfection, its efficiency was determined by detaching the transfected monolayer in a test well and counting the percentage of GFP-positive (and, hence, efficiently co-transfected by ACE2 + GFP) by FACS. Since the original 293FT cell line is not permissive to SARS-CoV-2 or rVSV/SARS-CoV-2 S pseudotype infection, the level of successfully transfected cells reports the permissivity of these target cells to these infections. In our experiments, we achieved the levels of transfection of ~ 50- 60%.
Also, the cytotoxicities of BA8 derivative and the parent compound, BA, were determined in ACE2-transfected target 293FT cells. Using the 100, 50, 25, and 12.5 mM 2-fold dilution series, BA8 proved non-toxic, likewise in Vero E6 cells, while BA demonstrated apparent toxicity, though, to a lesser extent, than in Vero E6 (10- 30% toxicity within 25 – 50 mM range, CC50 was not reached (Figure 2, A).
The same concentrations of compounds were used to inhibit SARS-CoV-2 or rVSV/SARS-CoV-2 S pseudotype infection following the entry inhibition protocol provided in the Methods. Target cells were preincubated with substances for 2 h, and infecting pseudotypes were then added to the wells. The infections were set up in triplicate wells, and the transduced luciferase signal, which reports the successful pseudotype entry/infection, was measured 24 h thereafter. The measured signals were represented as relative values normalized to the luciferase signal of positive control wells holding target cells and pseudotypes without inhibitors. The reference inhibitor was not included into the pseudotype entry inhibition assay, since coronavirus entry inhibitors are not currently commercially available (Figure 2, B).
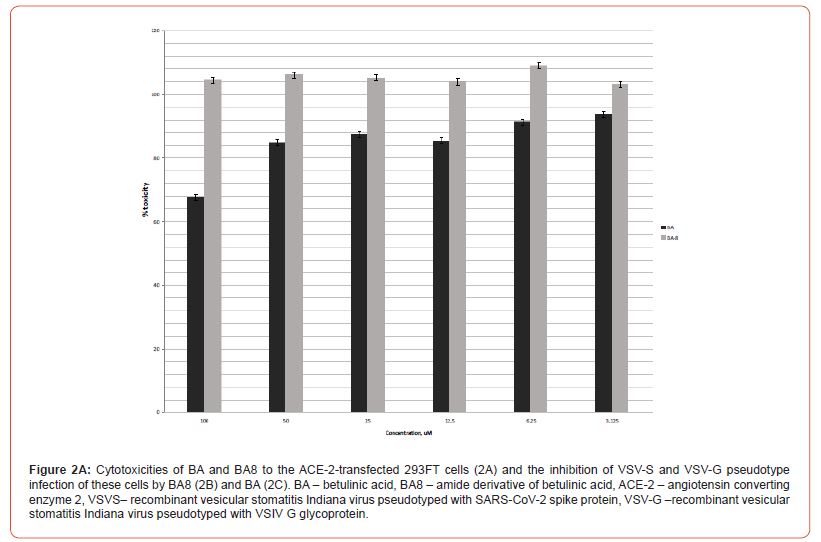
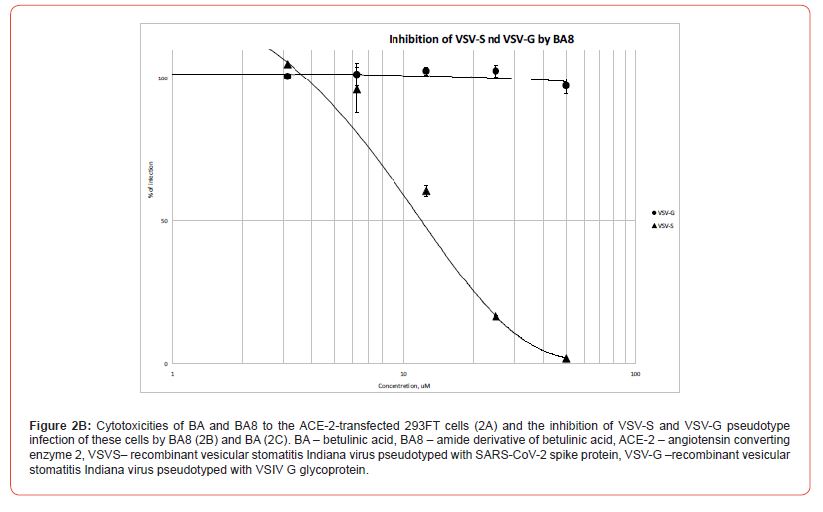
The pseudotype entry inhibition curves represented in Figure 2, B clearly show a specific inhibition of rVSV/SARS-CoV-2 S pseudotype (with IC50 slightly over 10 mM), while the rVSV/VSV-G remains barely suppressed, which enables us to classify BA8 as a specific SARS-CoV-2 entry inhibitor molecule. Conversely, betulinic acid itself only mildly reduces the signal of pseudovirus-transduced luciferase (30% reduction at 50mM of BA, Figure 2, C), which is, clearly, a non-specific effect, since both pseudotype entries mediated by SARS-CoV-2 spike protein S or VSIV glycoprotein G, are suppressed to an equal extent (Fig. 2, C). Meanwhile, this paired minor reduction in the measured luciferase signals observed at 25 and 50 mM of BA well correlate with the overall reduction of metabolic activity of 293FT cells in the presence of these BA concentrations (Figure 2, C).
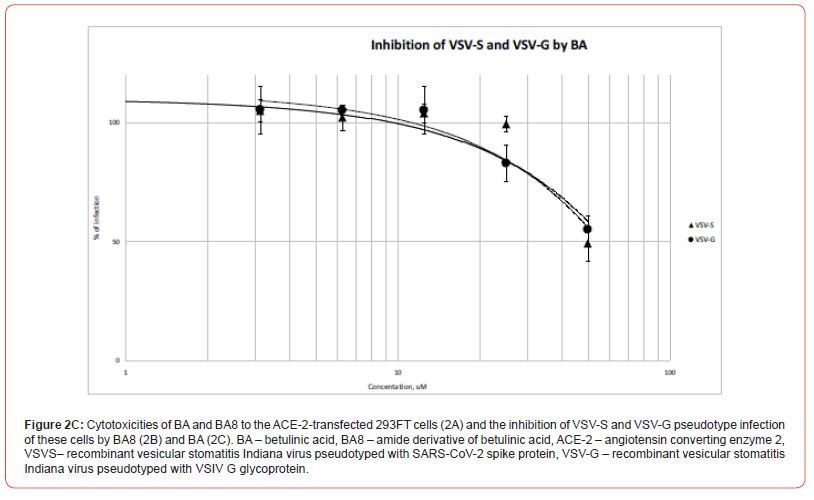
Discussion
In this paper, we report the betulinic acid amide derivative, which acts as an inhibitor of SARS-CoV-2 spike protein-mediated cell entry and possesses some inhibitory activity on the replication of an infectious SARS-CoV-2 isolate in an in vitro cell culture. Virus entry to the target cells is the earliest step in the infection, and entry inhibitors, thus, possess a number of attractive features. Since many of them are directed against extracellular targets (viral glycoproteins and cellular surface receptors), these are easily accessible to the inhibitor molecules of different nature (peptides, antibodies or small-molecule inhibitors). As they are acting on the other spectrum of targets, than the antivirals of other classes (polymerase and protease inhibitors or else), they develop a non-overlapping drug resistance profiles with such antivirals, which may be important for multidrug antiviral therapies, such as antiretroviral therapy against the HIV [42]. Entry inhibitors may also prevent the development of adverse immune responses, such as cytokine overproduction/storm, since the components of the virion do not access the cytosol upon such inhibition and, thus, do not trigger antiviral response.
A number of SARS-CoV-2 entry inhibitors have been already identified in the in vitro screens of approved drug libraries, which has an advantage of easy repurposing of such drugs for COVID-19 treatment. Such studies, typically, combine the pseudotype entry inhibition assay and the suppression of replication of an infectious SARS-CoV-2 isolate [43], both also used in our research. Even more compounds are predicted in silico to possess an entry inhibition activity against SARS-CoV-2 [44]. Betulinic acid and its derivatives has been long shown to inhibit cell entry of unrelated viruses, such as the HIV-1 [14,15] and herpes simplex virus, HSV-1 [18]. Its activity against coronavirus entry has not been extensively characterized, as yet, however, a number of structural and in silico studies (some of which are cited in the Introduction) predict several strong binding sites for betulinic acid and betulin in the structure of SARS-CoV-2 spike protein, which can serve as the targets for therapeutic intervention. One study demonstrated a good inhibitory activity of betulinic acid against ACE2:SARS-CoV-2 Spike interactions measured by an in vitro cell-free test [36].
A possible role of betulinic acid as an anti-coronavirus therapeutics of entry inhibitor class is underscored by two recent publications reporting the inhibition activity of a tri-herbal medicine, Coronil, targeting SARS-CoV-2 spike protein, both in terms of its fusogenic activity and fusion-irrelated toxicity [45,46]. Coronil could inhibit the ACE2:SARS-CoV-2 interactions measured in an ELISA-based cell-free model and reduced the infection of human lung epithelium cells by viral pseudotypes bearing SARSCoV- 2 spike proteins in their envelopes. Additionally, it modulated the production of proinflammatory cytokines (IL-6, IL-1β, and TNF-α) in the infected cells [45]. Moreover, in a humanized zebrafish model with human lung epithelium cells xenotransplanted into the swim bladder, coronil treatment inhibited the adverse toxic effects of an ectopic injection of recombinant SARS-CoV-2 spike protein, such as related mortality, behavioral fever, skin hemorrhage, renal cell degeneration and necrosis, as well, as the secretion of the above-mentioned proinflammatory cytokines [46]. Ultra-highperformance liquid chromatography analysis identified betulinic and ursolic acids, withaferine, rosmarinic acid and a number of other biologically active molecules in Coronil [46], many of which may possess antiviral activity.
In our work, betolinic acid itself did not show any significant antiviral activity, neither in pseudotype entry inhibition test, nor in the inhibition of SARS-CoV-2 infection in cultured cells. A 30% reduction of measured luciferase signal occurring at high BA concentrations (50 mM) in 293FT cells transduced by either of the pseudotypes is clearly correlated with a 30% reduction of metabolic activity of 293FT cells at the same concentration, and, thus, represents a non-specific effect.
Instead, and quite unexpectedly, betulonic acid demonstrated rather high toxicity in normal, non-transformed cell lines used as virus targets in our experiments (Vero E6 and 293FT), which is contrary to the previously reported toxicity of betulinic and betulonic acids to cancer, but not normal cells [27]. Both findings do not agree with the report of some SARS-CoV-2 inhibitory activity and good toxicity profiles (CC50 > 150 mM) of both betulinic and betulonic acids [30]. The discrepancy in Vero-E6 sensitivity to betulinic and betulonic acids can only be explained by the differences between our and the author`s varieties of this cell line or the varying toxicity of different BA batches.
Meanwhile, currently the data is being accumulated on the toxicity of BA-related compounds to normal cells. Despite being a mitochondrial toxin, betulinic is able to induce a mitochondriaindependent eryptosis of red blood cells, which are lacking these organelles, via the mechanism involving membrane permeabilization [5]. It also triggers apoptosis in differentiated rat PC12 cells, which serve as a model for the normally differentiating brain neurons, via ROS-mediated mitochondrial mechanism [6]. A recent research reports the toxicity of betulin to mouse and fish fibroblasts with CC50 comparable with those reported previously for cancer cell lines, again involving the mitochondrial pathway [7]. Thus, before the concept of lack of BA toxicity to normal cells is carefully addressed, preliminary testing of each particular cell line for BA toxicity is advisable.
Contrary to betulonic acid, its amide 6 (BA8) was lacking significant toxicity in both cell lines (TC50 higher, than 500 mM and 100 mM in VeroE6 and 293FT, respectively). Its virus inhibition activity in both tests employed has also been comparable (IC50 values of 40.8-131 mM and > 10 mM for SARS-CoV-2 and VSV pseudotyped with SARS-CoV-2 spike protein, respectively). This corroborates the validity of pseudotype entry inhibition test as the biologically safe and relevant assay for the coronavirus entry inhibitors, and makes BA8 a suitable candidate for the further modification in order to obtain more powerful molecules of this profile of action.
The last consideration, although not directly linked to the experimental part of this research, is concerned with suitability of different pseudotype platforms for the search of BA-related entry inhibitors. As seen from our and other`s experience, viral pseudotypes are convenient and safe tools for virus entry studies and entry inhibitor search. The two most popular pseudotype platforms exist, with different viral glycoproteins pseudotyping either VSIV capsid (as in our study) or the HIV capsid. Since BA derivatives can (and often do) block HIV capsid maturation, can they be analyzed as possible entry inhibitors using this lentiviral pseudotype platform?
Conclusions
Here, we present a first, to the best of our knowledge, report on the virus entry inhibitory activity of a betulinic acid amide derivative towards SARS-CoV-2 coronavirus and its biosafe model, a recombinant VSV virus pseudotyped with SARS-CoV-2 spike protein. Our future plans include the synthesis of BA-related compound starting from BA8 as a prototype molecule, further dissection of inhibition mechanism involving accurate time-ofaddition experiments, and a paired comparative study of VSV-based and lentiviral pseudotypes as the tools for the study of BA-related entry inhibitors.
Acknowledgements
Concept of the study – AGP, EES, SVC, design of the experiments – MS, OVP, SVC, and AAK, at-bench experimentation – MS, AAK, SC, ANV, PYuA and EF, manuscript preparation – SC, feedback contribution to the manuscript – all authors, overall supervision – AGP. All authors have read and agreed to the published version of the manuscript.”
Funding
This work was supported by the grant # 20-04-60314 from the Russian Foundation for Basic Research (RFBR).
Conflicts of Interest
The authors declare no financial or non-financial interest.
References
- Shintiapina AB, Shul’ts EE, Petrenko NI, Uzenkova NV, Tolstikov GA, et al. (2007) Effect of nitrogen-containing derivatives of the plant triterpenes betulin and glycyrrhetic acid on the growth of MT-4, MOLT-4, CEM, and Hep G2 tumor cells. Bioorg Khim 33(6): 624-628.
- Ebeling S, Naumann K, Pollok S, Wardecki T, Vidal-Y-Sy S, et al. (2014) From a traditional medicinal plant to a rational drug: understanding the clinically proven wound healing efficacy of birch bark extract. PLoS One 9(1): e86147.
- Amiri S, Dastghaib S, Ahmadi M, Mehrbod P, Khadem F, et al. (2020) Betulin and its derivatives as novel compounds with different pharmacological effects. Biotechnol Adv 38: 107409.
- Zhang X, Hu J, Chen Y (2016) Betulinic acid and the pharmacological effects of tumor suppression (Review). Mol Med Rep14(5): 4489-4495.
- Gao M, Lau PM, Kong SK (2014) Mitochondrial toxin betulinic acid induces in vitro eryptosis in human red blood cells through membrane permeabilization. Arch Toxicol 88(3): 755-768.
- Wang X, Lu X, Zhu R, Zhang K, Li S, et al. (2017) Betulinic Acid Induces Apoptosis in Differentiated PC12 Cells Via ROS-Mediated Mitochondrial Pathway. Neurochem Res 42(4): 1130-1140.
- Małaczewska J, Kaczorek-Łukowska E, Kazuń B (2021) High cytotoxicity of betulin towards fish and murine fibroblasts: Is betulin safe for nonneoplastic cells? BMC Vet Res 17(1): 198.
- Alakurtti S, Mäkelä T, Koskimies S, Yli-Kauhaluoma (2006) Pharmacological properties of the ubiquitous natural product betulin. J Eur J Pharm Sci 29(1): 1-13.
- Huang QX, Chen HF, Luo XR, Zhang YX, Yao X, et al. (2018) Structure and Anti-HIV Activity of Betulinic Acid Analogues. Curr Med Sci 38(3): 387-397.
- Chaniad P, Sudsai T, Septama AW, Chukaew A, Tewtrakul S, et al. (2019) Evaluation of Anti-HIV-1 Integrase and Anti-Inflammatory Activities of Compounds from Betula alnoides Buch-Ham. Adv Pharmacol Sci 2019: 2573965.
- Ríos JL, Máñez S (2018) New Pharmacological Opportunities for Betulinic Acid. Planta Med 84(1): 8-19.
- Smith PF, Ogundele A, Forrest A, Wilton J, Salzwedel K, et al. (2007) Phases I and II study of the safety, virologic effect, and pharmacokinetics/pharmacodynamics of single-dose 3-o-(3',3'-dimethylsuccinyl) betulinic acid (bevirimat) against human immunodeficiency virus infection. Antimicrob. Agents Chemother 51(10): 3574-3581.
- Huang L, Chen CH (2002) Molecular Targets of Anti-HIV-1 Triterpenes. Current Drug Targets - Infectious Disord 2(1): 33-36.
- Holz-Smith S L, Sun I C, Jin L, Matthews T J, Lee K H, et al. (2001) Role of human immunodeficiency virus (HIV) type 1 envelope in the anti-HIV activity of the betulinic acid derivative IC9564. Antimicrob Agents Chemother 45(1): 60-66.
- Labrosse B, Treboute C, Alizon M (2000) Sensitivity to a nonpeptidic compound (RPR103611) blocking human immunodeficiency virus type 1 env-mediated fusion depends on sequence and accessibility of the gp41 loop region. J Virol 74(5): 2142-2150.
- Dang Z, Lai W, Qian K, Ho P, Lee KH, et al. (2009) Betulinic acid derivatives as human immunodeficiency virus type 2 (HIV-2) inhibitors. J Med Chem 52(23): 7887-7891.
- Pavlova NI, Savinova OV, Nikolaeva SN, Boreko EI, Flekhter OB, et al. (2003) Antiviral activity of betulin, betulinic and betulonic acids against some enveloped and non-enveloped viruses. Fitoterapia 74(5): 489-492.
- Heidary Navid M, Laszczyk-Lauer MN, Reichling J, Schnitzler P (2014) Pentacyclic triterpenes in birch bark extract inhibit early step of herpes simplex virus type 1 replication. Phytomedicine 21(11): 1273-1280.
- Visalli RJ, Ziobrowski H, Badri KR, He JJ, Zhang X, et al. (2015) Ionic derivatives of betulinic acid exhibit antiviral activity against herpes simplex virus type-2 (HSV-2), but not HIV-1 reverse transcriptase. Bioorg Med Chem Lett 25(16): 3168-3171.
- Li Y, Jiang R, Ooi LS, But PP, Ooi VE, et al. (2007) Antiviral triterpenoids from the medicinal plant Schefflera heptaphylla. Phytother Res 21(5): 466-470.
- Hong EH, Song JH, Kang KB, Sung SH, Ko HJ, et al. (2015) Anti-influenza activity of betulinic acid from Zizyphus jujuba on influenza A/PR/8 virus. Biomol Ther (Seoul) 23(4): 345-349.
- Baltina LA, Flekhter OB, Nigmatullina LR, Boreko EI, Pavlova NI, et al. (2003) Lupane triterpenes and derivatives with antiviral activity. Bioorg Med Chem Lett 13(20): 3549-3552.
- Flechter OB, Boreko EI, Nigmatullina LR, Tret’yakova EV, Pavlova NI, et al. (2003) Synthesis and antiviral activity of ureides and carbamates of betulinic acid and its derivatives. Bioorg Chem 29(6): 655-661.
- Kazakova OB, Giniyatullina GV, Yamansarov EY, Tolstikov GA (2010) Betulin and ursolic acid synthetic derivatives as inhibitors of Papilloma virus. Bioorg Med Chem Lett 20(14): 4088-4090.
- Lin CK, Tseng CK, Chen KH, Wu SH, Liaw CC, et al. (2015) Betulinic acid exerts anti-hepatitis C virus activity via the suppression of NF-κB- and MAPK-ERK1/2-mediated COX-2 expression. Br J Pharmacol 172(18): 4481-4492.
- Yao D, Li H, Gou Y, Zhang H, Vlessidis AG, et al. (2009) Betulinic acid-mediated inhibitory effect on hepatitis B virus by suppression of manganese superoxide dismutase expression. FEBS J 276(9): 2599-2614.
- Zhang L, Lin D, Sun X, Curth U, Drosten C, et al. (2020) Crystal structure of SARS-CoV-2 main protease provides a basis for design of improved α-ketoamide inhibitors. Science 368(6489): 409-412.
- Mandal A, Jha AK, Hazra B (2021) Plant Products as Inhibitors of Coronavirus 3CL Protease. Front. Pharmacol 12: 583387.
- Macchiagodena M, Pagliai M, Procacci P (2020) Inhibition of the main protease 3CLPro of the coronavirus disease 19 via structure-based ligand design and molecular modeling.
- Wen CC, Kuo YH, Jan JT, Liang PH, Wang SY, et al. (2007) Specific plant terpenoids and lignoids possess potent antiviral activities against severe acute respiratory syndrome coronavirus. J Med Chem 50(17): 4087-4095.
- Pillaiyar T, Manickam M, Namasivayam V, Hayashi Y, Jung SH, et al. (2016) An overview of severe acute respiratory syndrome-coronavirus (SARS-CoV) 3CL protease inhibitors: peptidomimetics and small molecule chemotherapy. J Med Chem 59(14): 6595-6628.
- Romeo I, Mesiti F, Lupia A, Alcaro S (2021) Current Updates on Naturally Occurring Compounds Recognizing SARS-CoV-2 Druggable Targets. Molecules 26(3): 632.
- Alhadrami HA, Sayed AM, Sharif AM, Azhar EI, Rateb ME, et al. (2021) Olive-Derived Triterpenes Suppress SARS COV-2 Main Protease: A Promising Scaffold for Future Therapeutics. Molecules 26(9): 2654.
- Giofre SV, Napoli E, Iraci N, Speciale A, Cimino F, et al. (2021) CoV-2 key therapeutic targets: An in-silico study through molecular docking and dynamics simulations. Comput Biol Med 134: 104538.
- Wu C, Liu Y, Yang Y, Zhang P, Zhong W, et al. (2020) Analysis of therapeutic targets for SARS-CoV-2 and discovery of potential drugs by computational methods 10(5): 766-788.
- Carino A, Moraca F, Fiorillo B, Marchianò S, Sepe V, et al. (2020) Hijacking SARS-CoV-2/ACE2 Receptor Interaction by Natural and Semi-synthetic Steroidal Agents Acting on Functional Pockets on the Receptor Binding Domain. Front Chem 8: 572885.
- Basal WT, Elfiky A, Eid J (2021) Chaga Medicinal Mushroom Inonotus obliquus (Agaricomycetes) Terpenoids May Interfere with SARS-CoV-2 Spike Protein Recognition of the Host Cell: A Molecular Docking Study. Int J Med Mushrooms 23(3): 1-14.
- Zhang J, Lan Y, Sanyal S (2020) Membrane heist: Coronavirus host membrane remodeling during replication. Biochimie 179: 229-236.
- Wolff G, Melia CE, Snijder EJ, Barcena M (2020) Double-Membrane Vesicles as Platforms for Viral Replication. Trends in microbiology 28(12): 1022-1033.
- Dzhemilev UM, Tolstikov GA, Pokrovsky AG, Salakhutdinov NF, Tolstikova TG, et al. Pat WO2008054250A1
- Zuco V, Supino R, Righetti SC, Cleris L, Marchesi E, et al. (2002) Selective cytotoxicity of betulinic acid on tumor cell lines, but not on normal cells. Cancer Lett 175(1): 17-25.
- Melby T, Westby M (2009) Inhibitors of viral entry. Handb Exp Pharmacol (189): 177-202.
- Yang L, Pei RJ, Li H, Ma XN, Zhou Y, et al. (2020) Identification of SARS-CoV-2 entry inhibitors among already approved drugs. Acta Pharmacol Sin 28: 1-7.
- Choudhary S, Malik YS, Tomar S (2020) Identification of SARS-CoV-2 Cell Entry Inhibitors by Drug Repurposing Using in silico Structure-Based Virtual Screening Approach. Front Immunol 11: 1664.
- Balkrishna A, Solleti SK, Verma S, Varshney A (2020) Application of Humanized Zebrafish Model in the Suppression of SARS-CoV-2 Spike Protein Induced Pathology by Tri-Herbal Medicine Coronil via Cytokine Modulation. Molecules 25(21): 5091.
- Balkrishna A, Haldar S, Singh H, Roy P, Varshney A, et al. (2021) Coronil, a Tri-Herbal Formulation, Attenuates Spike-Protein-Mediated SARS-CoV-2 Viral Entry into Human Alveolar Epithelial Cells and Pro-Inflammatory Cytokines Production by Inhibiting Spike Protein-ACE-2 Interaction. J Inflamm Res 14: 869-884.
- Petrenko NI, NV Elantseva, VZ Petukhova, MM Shakirov, EE Shul′ts, et al. (2002) Synthesis of Betulonic Acid Derivatives Containing Amino-Acid Fragments Chem. Nat Compd 38(4): 331-339.
- Antimonova AN, N V Uzenkova, N I Petrenko, M M Shakirov, E E Shul′ts, et al. (2008) Synthesis of betulonic acid amides Chem. Nat Compd 44(3): 327-333.
- Sysolyatin SV, Surmachev VN, Malykhin VV, Kalashnikov AI, Surmacheva IA, et al. (2012) Synthesis of betulin derivatives: N’{N-[3-oxo-20(29)-lupen-28-oyl]-9-aminononanoyl}3-amino-3-phenylpropionic acid. Pharmaceutical Chem Journal 46(8): 474-477.
- Krasutsky PA (2006) Nat Prod Rep 23: 919-942. doi:10.1039/B606816B.
- Kruglova N, Siniavin A, Gushchin V, Mazurov D (2021) Different Neutralization Sensitivity of SARS-CoV-2 Cell-to-Cell and Cell-Free Modes of Infection to Convalescent Sera. Viruses 13(6): 1133.
- Baker R O, Bray M, Huggins J W (2003) Potential antiviral therapeutics for smallpox, monkeypox and other orthopoxvirus infections. Antiviral Research 57(1-2): 13-23.
- Paragas J, Whitehouse C A, Endy T P, Bray M (2004) A simple assay for determining antiviral activity against Crimean-Congo hemorrhagic fever virus. Antiviral Research 62(1): 21-25.
-
Cheresiz SV*, Kononona AA, Skarnovich M, Volkova AN, Poletaeva Yu A, Emaminia F, Pyankov OV, Schultz EE and Pokrovsky AG. An Amide Derivative of Betulonic Acid as a New Inhibitor of Sars-CoV-2 Spike Protein-Mediated Cell Entry and Sars-CoV-2 Infection. Insi in Chem & Biochem. 2(2): 2022. ICBC. MS.ID.000535.
-
Betulonic Acid, Sars-CoV-2 Spike, Protein-Mediated, Cell Entry, Betulinic acid, SARS-CoV-2, Pseudotype, Virus entry, Antivirals.
-
This work is licensed under a Creative Commons Attribution-NonCommercial 4.0 International License.