Mini Review Article
Protein Folding and Mis-Folding in Connection with Alzheimer’s Disease
Rajesh K. Nayak*
Department of Chemistry, Physics, and Engineering, Cameron University, USA
Rajesh K. Nayak, Department of Chemistry, Physics, and Engineering, Cameron University, 2800 W Gore Blvd, Lawton, OK, 73505 USA.
Received Date:October 02, 2023; Published Date:October 12, 2023
Abstract
Protein folding is one of the most pivotal fields of research of our time in chemistry, physics, molecular biology, biochemistry and computer science. Protein, after being synthesized in ribosome has to fold correctly in order to carry out its biological functions. But, unfortunately after lots of mutations in old age, sometime proteins in cellular milieu do not fold correctly and mis-fold and aggregate. The aggregation process leads to several debilitating neurodegenerative diseases including Alzheimer’s Disease (AD). This review will shed light on protein folding and mis-folding in connection with Alzheimer’s Disease and how Reverse Micelles (RMs) could be used as a simple model system which can mimic cellular environment to probe protein folding.
Keywords:Protein folding; Alzheimer’s Disease; AD; Reverse Micelles; RMs
Introduction
Proteins carry out numerous biological functions inside the cell [1] After being synthesized, proteins must fold three-dimensionally to become biologically active and perform their functions. Unlike in the test tube, where the folding process can occur spontaneously, in the biological cell, most proteins require assistance for proper folding. Molecular chaperones aid in the proper folding of proteins and deter aggregation of improperly folded ones [2] The correctly folded proteins are secreted from the cell and function normally in the extracellular environment. Under certain conditions, the proteins unfold, at least partially, and at this stage these may be prone to aggregation [2,3] This can result in the formation of fibrils and other aggregates that accumulate in the tissue. It is highly likely that these aggregates, as well as plaques (Figure 1), create various pathological conditions such as AD [4] It’s widely accepted that AD is a progressive neurodegenerative disorder, which is believed to happen due to the accumulation of β-amyloid plaques in the brain [2, 5-12].
AD is one of the most deadly and devastating neurodegenerative diseases and it is the most common form of dementia. Experts suggests that more than 35 million worldwide and approximately more than 6 million Americans of all ages may have AD. AD is currently ranked as the 7th leading cause of the death in USA. The death of brain cells and memory function loss associated with AD results from the accumulation of a fragment protein called Aβ protein in the brain (Figure 2) [13] A particular form of these aggregated Aβ proteins is highly ordered and is known as amyloid fibrils [14] Basically, these amyloid fibrils are generated from lower order oligomers which are believed to be toxic mis-folded protein [2] The amyloid β-peptide of 40 and 42 amino acid residues, commonly known as Aβ(1-40) and Aβ(1- 42) monomers, aggregate into insoluble clumps, responsible for synaptic dysfunction in the brains of AD patients [10-12] Aβ(1-42) monomer is more susceptible to aggregation than Aβ(1-40) monomer and it is the primary composition of the plaque.
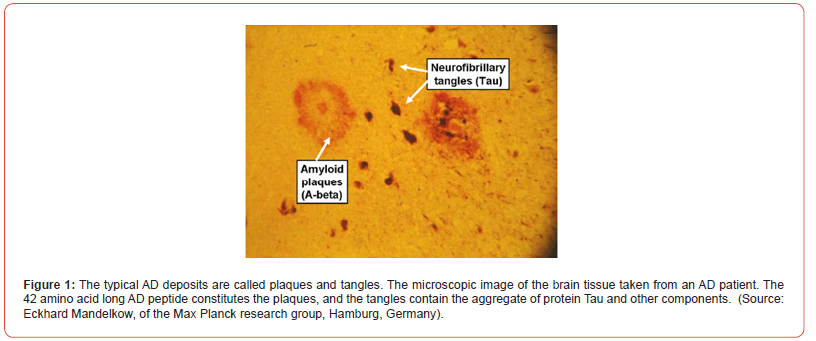
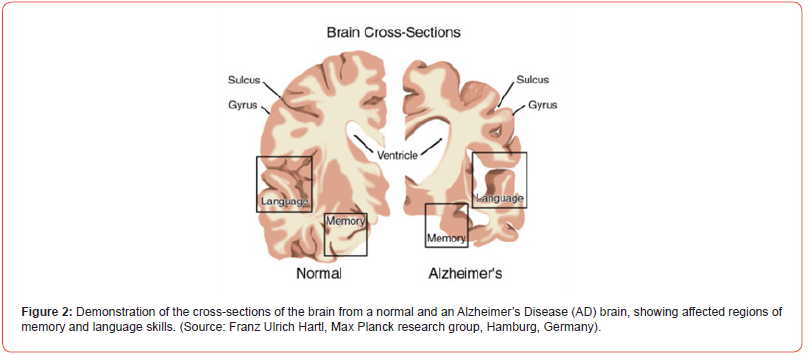
Protein -folding and AD
A clear understanding of monomer aggregation and plaque formation in AD patients requires better understanding of protein conformation and folding/mis-folding dynamics, as well as the mechanisms at the molecular level. Protein folding/mis-folding in biological cells takes place in a highly crowded and confined environment. Crowding and confinement may change some physicochemical properties of the environment, such as the local viscosity of cellular environments [15] Therefore, studies of proteins and peptides in a small confined or inert space that mimics the natural environment of the biological cell, may be more meaningful both for kinetics and structural characterization as well as the aggregation phenomenon in the extracellular space. The reverse micellar environment mimics the crowded conditions that exist in the extracellular spaces in the brain where fibril formation occurs.
Reverse Micelle Encapsulation as a Model System for Aggregation Studies
Reverse Micelles (RMs) can be used as a simple model system for protein structures and dynamic studies in extracellular spaces. RM is defined as a ternary system, consisting of oil, detergent, and water complexes, that is crowded and confined like a biological membrane (Figure 3) [16-18], The surfactant usually consists of hydrophilic head and hydrophobic tail groups. The surfactant head groups aggregate around a polar water core, whereas hydrophobic tails extend toward the non-polar solvent. The shape of the RMs is often assumed to be spherical and their size is directly proportional to the water-to-surfactant ratio (w0= [water]/ [surfactant]). The RMs will spontaneously form in the solution when the number of surfactant molecules is above the critical micelle concentration (CMC). The radius of the RM and the number of water molecules accessible to the peptide can be varied by manipulating the water loading capacity [15] The needed confinement geometry can be provided by using a RM to confine the peptide, thereby adjusting the ratio of the peptides to water in the encapsulation of peptides in the micellar water core [19].
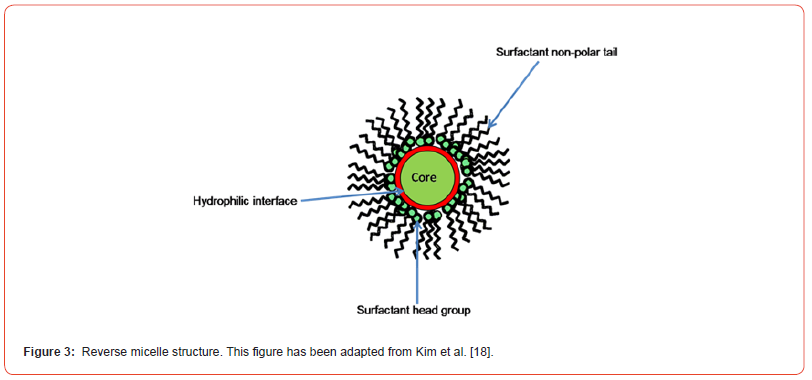
Discussion
Over the years, numerous studies have been carried out to understand amyloid fibril formation in the brains of AD patients in connection with protein folding and mis-folding studies, but several questions remain unanswered or poorly understood. For example: how do the Aβ (1-40) and Aβ (1-42) monomers aggregate? What is the probable kinetic mechanism of monomer aggregation, which is the prelude to fibril formation? Recent FTIR studies have shown that the 40-residue amyloid beta (Aβ) protein forms extended beta- strands in reverse micelles, while an analogue with a scrambled sequence does not (Figure 4) [19].
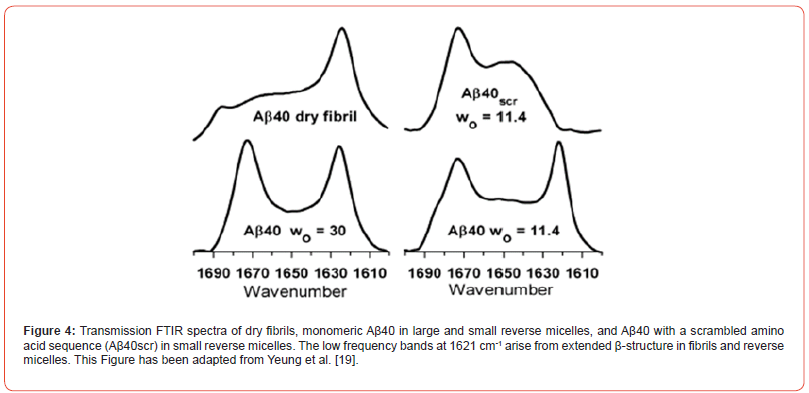
This result suggests that the Aβ sequence is inherently amyloidogenic, and that its amyloidogenicity is enhanced in a crowded, confined membrane-like environment of a reverse micelle [13] This result is significant because it suggests that these factors may nucleate or otherwise promote the formation of amyloid fibrils in the human brain in AD. Important kinetic questions still remain unan swered, such as: whether or not the β-strand observed by the Aβ(1- 40) monomer is the intermediate to fibril formation, or whether the protein folding and unfolding kinetics in the extracellular spaces of the brain proceed through several meta-stable intermediates. Is protein folding simply just a two-state process?
Previously, Kim et al [20] conducted time-resolved Fluorescence Resonance Energy Transfer (FRET) to study the amyloid aggregation of Aβ(11-25) proteins (Figure 7). Kim et al [20] hypothesized that the amyloid peptides undergo multi-step conformational changes during self-assembling, such as random coil(monomers), collapsed coil(multimers), micellar structures, and extended β-sheets in fibrils.
The above experimental studies clearly suggest that the protein mis-folding and aggregation in confined reverse micellar environments may be possible reasons for the synaptic disfunction in the brain of AD patients.
Conclusion
In summary, the review article has shed light on amyloid β-peptide, Aβ (1-40) and Aβ (1- 42) folding, misfolding and aggregation in connection with Alzheimer’s Disease. The review further suggests that the reverse micelle can be used as a simple model system, which can mimic the biological and cellular environment where protein resides. Protein folding dynamics can be investigated using RMs to unravel the mystery of this dangerous neurodegenerative Alzheimer’s Disease.
Acknowledgment
The authors would like to thank Oklahoma-IDeA Network of Biomedical Research Excellence (OK-INBRE) research grant for funding.
Conflict of Interest
No Conflict of interest.
References
- Dobson C (2003) Protein folding and misfolding. Nature 426(6968): 884-890.
- William E Balch, Richard I Morimoto, Andrew Dillin, Jeffery W Kelly (2008) Adapting proteostasis for disease intervention Science 319(5865): 916-919.
- Dobson CM (1999) Protein misfolding, evolution and disease. Trends Biochem. Sci. 24(9): 329-332.
- Konstanze F Winklhofer, Jörg Tatzelt, Christian Haass (2008) The two faces of protein misfolding: gain- and loss-of-function in neurodegenerative diseases. EMBO journal 27(2): 336-349.
- John J Balbach, Yoshitaka Ishii, Oleg N Antzutkin, Richard D Leapman, Nancy W Rizzo, et al. (2000) Amyloid Fibril Formation by Aβ16-22, a Seven-Residue Fragment of the Alzheimer’s β-Amyloid Peptide, and Structural Characterization by Solid State NMR. Biochemistry 39: 13748-13759.
- Abelson JN, Simon MI, Wetzel R (1999) Amyloid, prions, and other protein aggregates. Academic Press 309: 3-820.
- Kelly JW (1998) The alternative conformations of amyloidogenic proteins and their multi-step assembly pathways. Curr Opin Struct Biol 8(1): 101-106.
- Li H, Rahimi F, Sinha S, Maiti P, Bitan G (2009) Amyloids and Protein Aggregation–Analytical Methods. Encyclopedia of Analytical Chemistry.
- Christopher A Ross, Michelle A Poirier (2004) Protein aggregation and neurodegenerative disease. Nat Med 10: S10-S17.
- Christian Haass, Dennis J Selkoe (2007) Soluble protein oligomers in neurodegeneration: lessons from the Alzheimer's amyloid beta-peptide. Nat Rev Mol Cell Biol 8(2): 101-112.
- Fabrizio Chiti, Massimo Stefani, Niccolò Taddei, Giampietro Ramponi, Christopher M Dobson, et al. (2003) Rationalization of the effects of mutations on peptide and protein aggregation rates. Nature 424(6950): 805-808.
- Ghosh P, Kumar A, Datta B Rangachari V (2010) In Proceedings of the First ACM International Conference on Bioinformatics and Computational Biology. ACM 451.
- Massimo Stefani, Christopher M Dobson (2003) Protein aggregation and aggregate toxicity: new insights into protein folding, misfolding diseases and biological evolution. J Mol Med 81(11): 678-699.
- Oleg N Antzutkin, John J Balbach, Robert Tycko (2003) Site-Specific Identification of Non-β-Strand Conformations in Alzheimer's β-Amyloid Fibrils by Solid-State NMR. Biophys J 84(5): 3326-3335.
- Mukherjee S, Chowdhury P, Gai FJ (2009) Phys. Chem. B 113: 531-535.
- Nancy E Levinger, Laura A Swafford (2009) Ultrafast dynamics in reverse micelles. Rev Phys Chem. 60: 385-406.
- Nancy E Levinger (2000) Ultrafast Dynamics in Reverse Micelles, Microemulsions, and Vesicles. Current opinion in colloid & interface science 5(1): 118-124.
- Daniel Kim, Hannah Spoon, Rajesh K, Nayak (2023) Insi in Chem& Biochem 2.
- Yeung PSW, Axelsen PH (2012) J. Am Chem Soc 134: 6061-6063.
- Kim J, Lee M (2004) Biochem. Biophys. Commun 316: 393-397.
-
Rajesh K. Nayak*. Protein Folding and Mis-Folding in Connection with Alzheimer’s Disease. Insi in Chem & Biochem. 2(5): 2023. ICBC. MS.ID.000549.
-
Reverse micelles, Confined environment, RM, AOT
-
This work is licensed under a Creative Commons Attribution-NonCommercial 4.0 International License.