Research article
Bronchopulmonary Dysplasia Risk Prediction Models among Very Low Birth Weight Infants Receiving Caffeine Therapy
Mounika Polavarapu PhD1*, Praveen Kumar MD2, Hillary Klonoff-Cohen PhD3, Divya Joshi MD4, Ruopeng An PhD5 and Karin Rosenblatt PhD3
1Department of Population Health, University of Toledo, Toledo, Ohio
2Department of Pediatrics, Children’s Hospital of Illinois, Peoria, Illinois
3Department of Kinesiology and Community Health, University of Illinois at Urbana-Champaign, Urbana, Illinois
4Johns Hopkins All Children’s Hospital, St. Petersburg, Florida
5Washington University in St. Louis, St. Louis, Missouri
Mounika Polavarapu, PhD Department of Population Health, University of Toledo, Ohio.
Received Date:February 19, 2024; Published Date:March 01, 2024
Abstract
Objectives: To develop risk prediction models for overall and moderate-severe bronchopulmonary dysplasia (BPD) on Days 1, 7, and 21, and compare these to 2011 NICHD model, in a cohort of VLBW infants all receiving prophylactic caffeine treatment.
Methods: This retrospective chart review included 448 infants born between 2012-2018 with gestational age <30 weeks. Models were constructed using step-wise forward logistic regressions, and predictive performances assessed using c-statistic.
Results: BPD developed in 215 (47.9%) infants. “Gestational age,” “type of ventilation,” and “average FiO2 levels” were predictive of BPD (and moderate-severe BPD) on Days 1 and 7; only the latter two factors were predictive on Day 21. Our model’s performance was comparable to the 2011 NICHD model for Days 7 (p=.07) and 21 (p=.14) but was lower for Day 1 (p<.001).
Conclusion: We developed simple, and reliable models for BPD and moderate-severe BPD that neonatologists could easily implement in fastpaced NICU settings.
Bronchopulmonary Dysplasia Risk Prediction Models among Very Low Birth Weight Infants Receiving Caffeine Therapy
Keywords:BPD; Prediction model; Caffeine treatment; VLBW; Prematurity
Author Contribution Statement: MP: Conceptualization, Methodology, Formal Analysis, Data interpretation, Writing original draft and, Reviewing and editing final draft; PK: Conceptualization, Methodology, Data interpretation, and Critically reviewing and editing final draft; HKC: Data interpretation, Writing original draft and, Critically reviewing and editing final draft; DJ: Methodology, Critically reviewing and editing final draft; RA: Methodology, Critically reviewing and editing final draft; KR: Methodology, Critically reviewing and editing original draft
Introduction
Bronchopulmonary Dysplasia (BPD) is a form of chronic lung disease commonly seen in premature infants that requires oxygen therapy [1]. The oxygen injures the lungs and the airways, leading to inflammation and tissue destruction (dysplasia) in the alveoli. [1] Despite advances in neonatal care, BPD remains a significant cause of morbidity and mortality among very low birth weight infants [2-4]. The prevention and management of BPD pose significant challenges in neonatal care as well as increase public health costs [5].
Identifying infants at high risk of developing BPD is important to optimize their respiratory support, facilitate appropriate followup care to prevent the progression of the disease and its associated complications, and provide families with accurate prognostic information. Several prediction models for BPD have been proposed; although, the most accurate and commonly used one in clinical practice, which has been externally validated, is the 2011 NICHD BPD estimator [6-8]. It has been widely adopted because it categorized BPD by severity, including infants who died, and examined models from day 1 through the first 28 postnatal days [6].
Both theophylline and caffeine are drugs that stimulate breathing efforts and were used to reduce apnea of prematurity beginning in 1970’s and 1980’s [9]. It was determined that caffeine was more easily absorbed and had a longer half-life, allowing for once daily dosage [10]. The American Academy of Pediatrics first began recommending the use of caffeine in 2006 to prevent BPD in infants who were <30 weeks gestational age and/or <1250 grams [11, 12]. The 2011 NICHD BPD estimator was developed based on a 2000-2004 cohort when caffeine was still being investigated for its potential role in the prevention of BPD. Currently, caffeine is one of the most prescribed medications in neonatal intensive care units for preventing BPD and managing apnea of prematurity [13, 14]. However, there is no standardized protocol on optimal dosing and timing of caffeine administration [15]. The Caffeine for Apnea of Prematurity (CAP) Trial suggested a decrease in BPD by 52% with early caffeine treatment from 1-3 days compared to a 23% reduction in risk when administered after Day 3 [16]. Caffeine, initiated within the first 10 days after birth, is one of few drug therapies shown to significantly decrease the risk of BPD in very low birth weight infants 17. Widespread use of caffeine does not prevent BPD in all cases, and, as a result, continued exploration for novel therapeutics is ongoing [17].
Objective
Our study aimed to develop prediction models for BPD using a recent cohort of infants born between 2012 and 2018, who all received prophylactic caffeine treatment. This addresses a significant gap in the literature by accounting for the now standard use of caffeine therapy in neonatal care. This may provide clinicains with a contemporarily relevant tool in predicting the risk of BPD. The primary aims of this study were to:
(i) develop BPD risk prediction models on Days 1, 7, and 21.
(ii) compare the performance of newly developed models with the 2011 NICHD model for BPD risk prediction.
(iii) develop risk prediction models specifically for moderatesevere BPD on Days 1, 7, and 21.
Methods
Study Design and Data: A retrospective chart review was performed on de-identified data obtained from electronic medical records at a Level III neonatal intensive care unit (NICU) in Illinois. This study received approval from our Institutional Review Board (#00000688).
The inclusion criteria included infants born:
(i) between 2012 and 2018,
(ii) with a gestational age of <30 weeks. Infants with major congenital anomalies (i.e., genetic anomaly, syndrome, or malformation of a primary organ system) and those who died before the diagnosis of BPD were excluded from the study.
Outcome variable: Bronchopulmonary dysplasia (BPD) was defined as infants receiving supplemental oxygen for 28 days or more by the postmenstrual age of 36 weeks [6, 18]. BPD was classified as
(i) mild, when receiving oxygen for >28 days but not at 36 weeks.
(ii) moderate, when receiving oxygen for >28 days and treatment with <30% oxygen at 36 weeks.
(iii) severe, when receiving oxygen for >28 days and treatment with >30% oxygen at 36 weeks [6, 18]. If the baby was discharged or transferred before the time of assessment, the BPD was defined based on the status of oxygen therapy at the time of discharge/transfer.
Predictor variables: Predictor variables were selected a priori based on confirmation in the literature, congruence with biological plausibility, and availability of information approved for release by the Department of Pediatrics. Information about gestational age, birth weight, gender, and race/ethnicity of each infant was obtained from electronic medical records. Gestational age was stratified into two categories, less than or equal to 27 weeks and greater than 27 weeks.
The timing of the first dose of caffeine variable referred to infants receiving caffeine within 24 hours, 48 hours, and >48 hours after birth. The data on respiratory support was collected as none, Continuous Positive Airway Pressure/Biphasic, nasal IMV (Intermittent Mandatory Ventilation), SIMV, Assist control, and high-frequency ventilation. If an infant received greater than one type of respiratory support on the day of assessment, the most aggressive form of ventilation for that day was entered into the dataset. Nasal IMV, CPAP, and biphasic types of respiratory support were grouped as non-invasive, and SIMV, assist control, and highfrequency ventilation types were classified as invasive types of ventilation.
We utilized non-invasive ventilation (as opposed to none) as a reference category to permit a direct comparison between invasive and non-invasive types of ventilation. The average FiO2 levels recorded on the day of risk estimation were used in the model.
Birth weight and average FiO2 levels, were recorded as continuous variables. The remaining variables (i.e., gender, race, maternal chorioamnionitis, antenatal steroids, administration of caffeine within 24 hours, administration of caffeine within 48 hours, and type of respiratory support) were recorded as categorical variables.
Statistical analysis: Descriptive statistics were used to characterize the cases and controls using mean and standard deviation for continuous variables and percentages for categorical variables. All analyses were performed using STATA 17 statistical software.
The aim of the analyses was to identify demographic and clinical variables that could significantly predict the risk of BPD and moderate to severe BPD on Days 1, 7, and 21 postnatal age. Binary logistic regression models were constructed using a stepwise forward selection of variables with exclusion criteria of 0.05 to predict the risk of each outcome. The first step of developing the model was to examine the relationship between BPD and each risk factor (univariate analysis) using the study data. All statistically significant variables at the alpha level of 0.05, along with a priori predictors, were entered into the model. This is contrary to the NICHD Estimator, which based final model selection less on statistical significance and more on how a predictor enhanced the model’s predictive ability.
Missign data: The variable on antenatal steroids had 18% missing data for which multiple imputations method by Chained Equations methods was employed. A logistic regression was employed and five imputed datasets were generated with plausible values derived from the observed data distribution. Subsequently, the results from 5 datasets were combined to obtain final consolidated estimates. Finally, a follow-up sensitivity analysis was perfomed comparing data with and without imputations to evaluate the impact of missing data.
Model validation and performance: The internal validity assessment of our logistic regression models was performed using 1000 bootstrap resamples, the most preferred approach for validating prediction models [19, 20]. This validation was performed by refitting each multivariable model in 1000 bootstrap resamples with replacement. The C-statistic was used to assess discrimination, which represented the area under the receiver operating characteristic curve to evaluate the fit of the final logistic regression and its performance. C-statistic represented the probability that a randomly selected patient who experienced the disease (case) had a higher risk than a patient who did not experience the disease (control).
Results
Between November 1st, 2012 and October 31st, 2018, 448 infants with a gestational age of <30 weeks met the inclusion criteria. The sample consisted of 43.9% female infants. The racial distribution was representative of the population served by the hospital, with the sample consisting of predominantly Caucasians (68.1%), followed by Blacks (24.4%), Asians (1.9%), and others (5.6%). Among the infants in our cohort, 215 (47.9%) infants developed BPD; specifically, 22.3% had mild BPD, 13.2% had moderate BPD, and 12.5% had severe BPD. The mean birth weight was 959.5grams (SD: 260.5), and the average gestational age was 27.1 weeks (SD: 1.8) (Table 1).
Table 1:Infant characteristics and BPD outcomes.
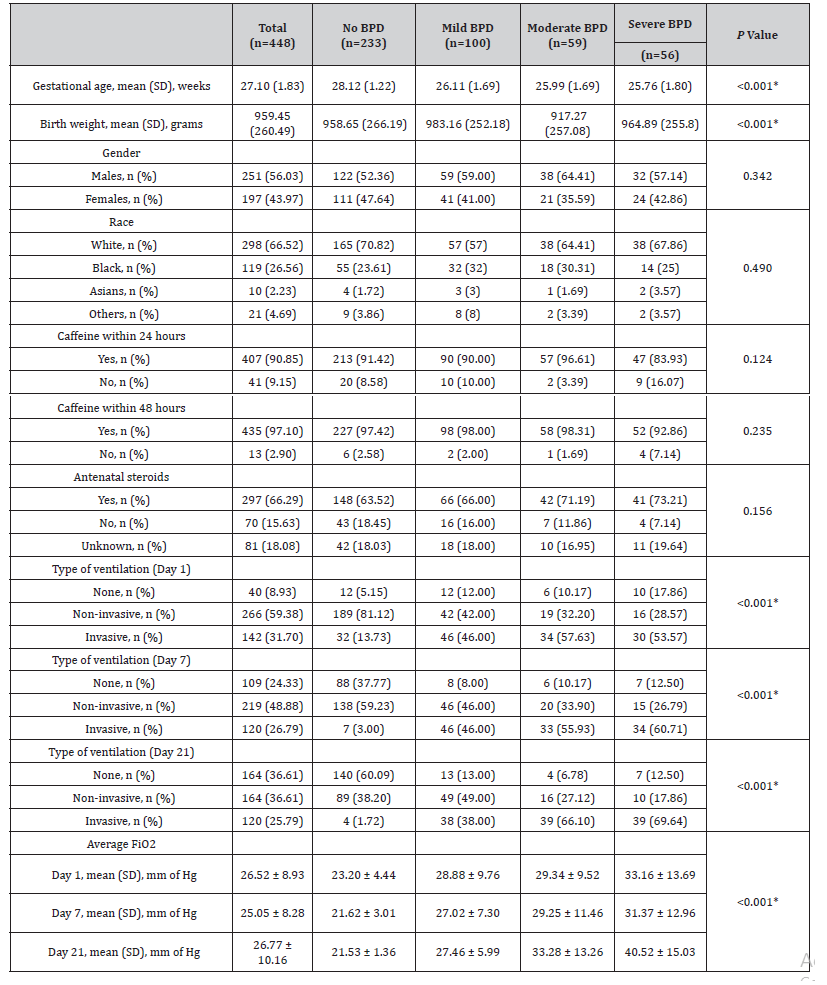
Among the mothers, 7.4% were diagnosed with chorioamnionitis during pregnancy, and 64.2% were known to have received antenatal steroids. Additionally, 89.9% of infants received caffeine within 24 hours, 95.5% within 48 hours, and 100% within day 10.
Prediction Models
Our predictive models were derived from the following covariates:
(i) gestational age
(ii) birth weight
(iii) race
(iv) gender
(v) type of respiratory support
(vi) average FiO2 levels on that day
(vii) caffeine given within 24 hours (for day 1), within 48 hours
(for day 7 and day 21)
(viii) antenatal steroids
(ix) maternal chorioamnionitis. Multiple imputation method was employed for missing data for antenatal steroids.
A total of 6 prediction models were developed for the outcomes of BPD and moderate-severe BPD on 1, 7, and 21 days after birth.
Day 1
Day 1- Prediction of BPD:
The primary predictors on Day 1 for BPD were gestational age, type of ventilation, and average FiO2 values (Table 2a). Infants with gestational age < 27 weeks were 7 times more likely to develop BPD (aOR= 7.20, 95% CI: 4.40-11.77). Additionally, those receiving invasive ventilation on Day 1 were 3.27 times more likely (aOR= 3.27, 95% CI: 1.87-5.73) compared to non-invasive ventilation to develop BPD. Finally, a 10 unit increase in average FiO2 on Day 1 was associated with nearly three times an increase [(aOR 1.11)10 = 2.84] in the likelihood of developing BPD. This model had a good c-statistic value of 0.87. The sensitivity and specificity of the model were 78.60% and 78.54%, respectively.
Day 1- Prediction of moderate-severe BPD:
The predictors estimated on Day 1 for moderate-severe BPD were the same as those for overall BPD (Table 2b). Infants with gestational age <27 weeks were three times more likely to develop moderate-severe BPD (aOR= 3.14, 95% CI: 1.98-4.99), and those receiving invasive ventilation on Day 1 were 2.36 times more likely (aOR= 2.36, 95% CI: 1.45-3.87) compared to non-invasive ventilation to develop moderate-severe BPD. Finally, a 10-unit increase in average FiO2 on Day 1 was associated with 1.63 times more likelihood [(aOR 1.05)10 = 1.63] of developing moderatesevere BPD. This model had a c-statistic value of 0.77.
Table 2:Prediction models on Day 1
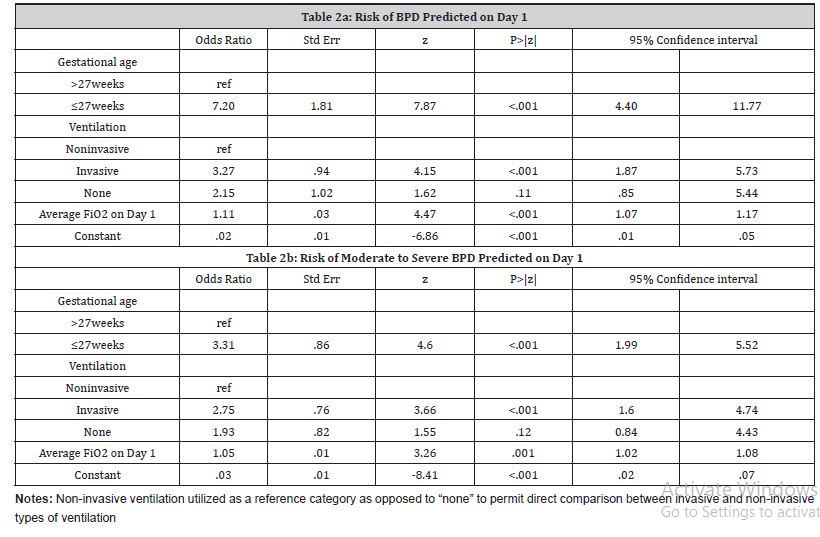
Day 1- Comparison with NICHD model:
The NICHD prediction model that we applied to our sample to predict BPD had a significantly higher c-statistic value (c-statisticNICHD= 0.90) than our model (c-statistic = 0.86) with a p-value <0.001. However, none of the additional variables of race, gender, and birth weight were statistically significant in our cohort.
Day 7
Day 7- Prediction of BPD:
The variables predicting the risk of BPD estimated on Day 7 included the same as on Day 1 (Table 3a):
(i) gestational age of ≤27 weeks (aOR= 3.30, 95% CI: 1.87- 5.82)
(ii) invasive ventilation compared to non-invasive ventilation (aOR= 9.59, 95% CI: 4.01-22.93)
(iii) average FiO2 on Day 7 (aOR= 1.23, 95% CI: 1.12-1.36). For each 10-unit increase in FiO2 values, the risk of developing BPD increased by about eight times [(aOR 1.23)10= 7.93]. The c-statistic value for this model was 0.90. The sensitivity and specificity of the model were 75.35% and 90.56%, respectively.
Day 7- Prediction of moderate-severe BPD:
The factors predictive of the risk of moderate-severe BPD estimated on Day 7 were gestational age, invasive ventilation, and average FiO2 levels (Table 3b). The model had a c-statistic value of 0.81.
Table 3:Prediction models on Day 1
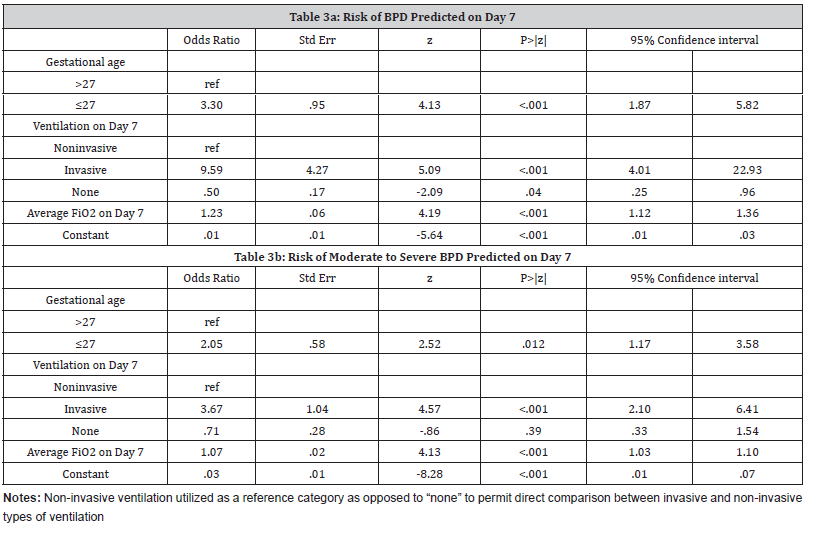
Day 7- Comparison with NICHD model:
One Day 7, there was no significant difference in c-statistic values for our model (c-statistic= 0.90) and the NICHD model (c-statisticNICHD= 0.91, p=.07). Similar to Day 1, none of the additional variables of race, gender, and birth weight were statistically significant in our cohort.
Day 21
Day 21- Prediction of BPD:
On Day 21 assessment, only type of ventilation and average FiO2 levels predicted BPD at 36 weeks PMA (Table 4a). Infants receiving invasive ventilation on Day 21 were 9.3 times more likely to develop BPD compared to those receiving non-invasive ventilation (aOR= 9.29, 95% CI: 2.86-30.20). This model had a c-statistic value of 0.95. The sensitivity and specificity values were 84.19% and 93.99%, respectively.
Day 21- Prediction of moderate-severe BPD:
On day 21, the same two factors, type of ventilation and average FiO2 levels, were predictive of moderate-severe BPD at 36 weeks postmenstrual age (Table 4b). A 10-unit increase in FiO2 values on day 21 was associated with more than four times more likelihood [(aOR 1.16)10 = 4.41] of developing moderate-severe BPD. The c-statistic value of this model was 0.90.
Table 4:Predictive models on Day 21.
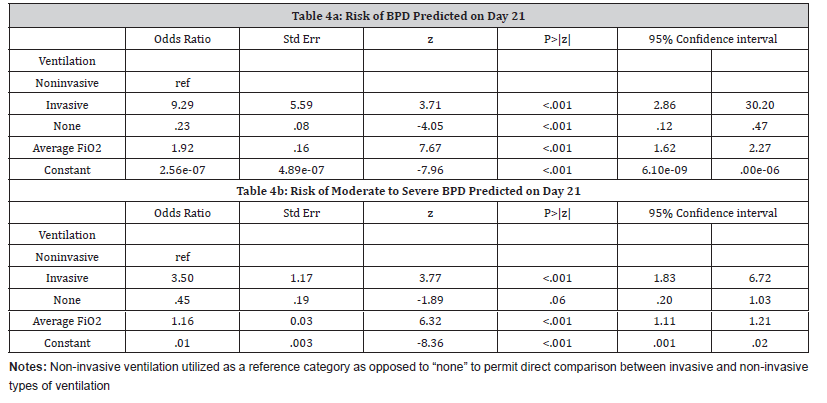
Day 21- Comparison with NICHD model:
Similar to Day 7, on Day 21, the prediction of BPD using the NICHD model (c-statisticNICHD= 0.95) was not statistically different from our model (c-statistic= 0.95, p=.14).
Discussion
Prediction models have a unique role in medical practice, public health, and patient care. Hence, BPD prediction models have the ability to predict the risk of BPD early after birth to determine which infants will benefit most from interventions that may reduce the risk of BPD.
We developed prediction models for BPD for VLBW infants for days 1, 7, and 21 after birth and compared the performance of our model with the most frequently used traditional /classic NICHD model. Our model’s performance was on par with NICHD for Days 7 and 21 but lower for Day 1. Overall, it is apparent that our model is preferable because it is clinically simpler with fewer and readily available parameters, thereby enhancing its feasibility and applicability in a fast-paced clinical setting in the NICU, allowing neonatologists to incorporate the model into their routine practice easily. This echoes the recommendation provided by a recent systematic review and meta-analysis of BPD prediction models, stating that the predictors within the model should be routinely assessed as part of clinical practice and not reliant on factors that may vary with clinical practice, such as changes in weight and fluid intake [8].
Our findings revealed that “gestational age,” “type of ventilation,” and “average FiO2 levels” were predictive of BPD (as well as moderate-severe BPD) on Days 1 and 7. In contrast, only “type of ventilation’ and “average FiO2 levels” were predictive of BPD (as well as moderate-severe BPD) on Day 21. The predictive value of “gestational age” was strongest on day 1 but decreased on Day 7 and disappeared on Day 21. In contrast, the predictive value of “type of ventilation” and “FiO2 values” continued to increase from Day 1 to Day 7 to Day 21.
The 2011 NICHD model that was used in this study was developed in a 2000-2004 cohort prior to the clinical usage of caffeine to prevent BPD [6]. One of the major advantages of our sample is that all the infants received caffeine treatment within 10 days of birth, which is known to decrease the risk of BPD among low-birth-weight infants significantly. Out of the total study sample, 91% of infants received caffeine within 24 hours of birth, 97% received it within 48 hours, and 100% by Day 10. The status of caffeine administration was not reported among infants included in recent prediction models, except for the prediction model developed by Dai and colleagues [21]. They used a machine learning technique to develop their model but reported only 80% of included infants received caffeine therapy [21].
Various prediction models have been published in the literature; however, accurate prediction still remains an enigma due to studies utilizing different timings for evaluation, dissimilar predictors, and non-identical statistical methods [22]. External validation of the existing prediction models published by Onland et al. in 2013 concluded that only two models were possibly clinically relevant with external validity [7]. The first was the NICHD model [6]. The second validated model by Ryan et al. had limited usefulness because it estimated the risk of BPD only on the 4th postnatal day and was only used for infants receiving mechanical ventilation [7, 23].
In 2019, a multicenter study including 16,407 infants weighing 500–1500 grams from the Neocosur Network database proposed BPD prediction models using step-wise logistic regression modeling formoderate–severe BPD or death at four postnatal ages: at birth and at 3, 7, and 14 days 24. Similar to our findings, birthweight, and gestational age had higher contributions to the prediction model at birth, and the length of oxygen therapy and ventilation had the highest contribution to the subsequent models on days 3, 7, and 14 24. One of their limitations was the exclusion of specific respiratory parameters of the fraction of inspired oxygen (FiO2) levels on each day of prediction, which was subsequently addressed in our study [24].
Two risk scores for BPD using multivariate logistic regression models and weights assigned to coefficients were reported in the literature [25, 26]. The risk scoring system by Gursoy and colleagues developed with a cohort from 2006-2009 included birth weight, gestational age, gender, hemodynamically significant patent ductus arteriosus, respiratory distress syndrome, hypotension, and intraventricular hemorrhage as predictors of BPD. [25] Similarly, Jassem-Bobowicz and colleagues proposed a risk score system utilizing data from 278 neonates born between 2013-2017 at a single center [26]. In this model with an AUC of 0.932, four factors were significantly associated with BPD, including gestational age, number of Red Blood Cell transfusions, number of surfactant administrations, and presence of hsPDA [26]. However, unlike our study, these risk scores were not measured at a particular time point(s), thereby not enabling clinicians to perform BPD risk assessment on specific day(s) to make a decision about adopting preventive strategies.
Finally, in 2022, the NICHD developed a new BPD risk estimator using the 2019 NRN BPD definition based on clinical data of 9181 infants born <29 weeks gestation to estimate BPD risk on postnatal days 1, 3, 7, 14, and 28 [27]. Because the 2022 risk estimator has not been externally validated, and our study population was diagnosed with BPD between 2012-2018, we choose to utilize the 2011 NICHD BPD estimator for all comparisons [27]. In fact, the 2011 NICHD BPD estimator has been used repeatedly in clinical practice and research [6, 8].
Strengths and Limitations: Our study has some notable limitations. The cohort was drawn from a single center, raising concerns about generalizability of the prediction model in a different setting. There was also underrepresentation of certain racial-ethnic groups in our cohort, particularly Hispanics and Asians; albeit Blacks were well represented. The study cohort reflected the population served by the hospital. Finally, unlike the 2011 NICHD Neonatal BPD Outcome Estimator, our model has not been externally validated.
There are many strengths of our study. One of the primary advantages of our prediction model is its ease of use for clinicians, particularly with the inclusion of a maximum of three variables. Second, our cohort reflects current clinical practices where all infants received caffeine as prophylactic treatment for BPD. Third, we estimated the risk of both overall BPD as well as moderate-severe BPD on days 1, 7, and 21. While it may not always be possible to completely prevent BPD from occurring in these infants, identifying those at risk of moderate-severe BPD could enable pediatricians to reduce the severity of the disease and its associated complications through early intervention/prevention.
Finally, this study addressed all methodological shortcomings reported in a recent systematic review of existing prediction models for BPD in preterm infants [28]. First, the sample size was fairly large. Second, the missing data were handled using multiple imputation methods to avoid a biased performance model. Third, the selection of predictor variables was not based on univariate analysis but rather using a forward selection logistic regression model. Once externally validated, our approach to include only the significant variables selected in a step-wise regression simplified the model for incorporation in clinical use. Fourth, we reported the discrimination and calibration performance of all the models developed in the study. Finally, the internal validity of the models was assessed using the preferred bootstrapping method.
Conclusion
We focused on developing a simple, reliable, and interpretable model that neonatologists could easily implement in the NICU. To ensure our model was contemporary, we based our choice of infant cohorts on current clinical practices (e.g., receiving caffeine.) The ultimate goal of this proposed model is to inform clinical decisions and treatment and, most importantly, improve VLBW infant outcomes.
In the future, the risk prediction models should take into account the treatments and interventions (e.g., surfactant) that are administered to premature infants in the subsequent days following their birth and be updated based on infant’s response to such treatment. Furthermore, studies should also investigate the appropriate therapeutic window (timing, dosage) for caffeine treatment. Finally, long-term consequences of this treatment in VLBW infants should be examined during adolescence and adulthood.
Data availability statement
De-identified data that support the findings of this study are available on request from the corresponding author, [MP] and approval from the institutional review board.
Ethics approval statement
This study received approval from the Institutional Review Board (#00000688)
Funding
None.
Acknowledgment
None.
Conflict of Interest
The authors have no conflicts of interest to declare.
References
- The American Lung Association (2023) (n.d.). Learn about bronchopulmonary dysplasia (BPD).
- Sahni M, Mowes AK (2022) Bronchopulmonary Dysplasia. In: StatPearls. Treasure Island (FL): StatPearls.
- Geetha O, Rajadurai VS, Anand AJ, Rowena Dela Puerta, Bin Huey Quek, et al. (2021) New BPD-prevalence and risk factors for bronchopulmonary dysplasia/mortality in extremely low gestational age infants ≤28 weeks. J Perinatol 41(8): 1943-1950.
- Tracy MK, Berkelhamer SK (2019) Bronchopulmonary Dysplasia and Pulmonary Outcomes of Prematurity. Pediatr Ann 48(4): e148-e153.
- Bonadies L, Zaramella P, Porzionato A, Perilongo G, Muraca M, et al. (2020) Present and Future of Bronchopulmonary Dysplasia. J Clin Med 9(5): 1539.
- Laughon MM, Langer JC, Bose CL, P Brian Smith, Namasivayam Ambalavanan, et al. (2011) Prediction of bronchopulmonary dysplasia by postnatal age in extremely premature infants. Am J Respir Crit Care Med. 183(12): 1715-1722.
- Onland W, Debray TP, Laughon MM, Martijn Miedema, Filip Cools, et al. (2013) Clinical prediction models for bronchopulmonary dysplasia: a systematic review and external validation study. BMC Pediatr 13:207.
- Kwok TC, Batey N, Luu KL, Prayle A, Sharkey D, et al. (2023) Bronchopulmonary dysplasia prediction models: a systematic review and meta-analysis with validation. Pediatr Res 94(1): 43-54.
- Henderson-Smart DJ, De Paoli AG (2010) Prophylactic methylxanthine for prevention of apnoea in preterm infants. Cochrane Database Syst Rev 2010(12): CD000432.
- Henderson-Smart DJ, Steer PA. Caffeine versus theophylline for apnea in preterm infants. Cochrane Database Syst Rev 2000(2): CD000273.
- Finer N, Higgins R, Kattwinkel J, Martin R (2006) Summary proceedings from the apnea-of-prematurity group. Pediatrics 117(3 Pt 2): S47-S51.
- Schmidt B, Roberts RS, Davis P (2006) Caffeine therapy for apnea of prematurity. N Engl J Med 354(20): 2112-2121.
- Kumar VHS, Lipshultz SE (2019) Caffeine and Clinical Outcomes in Premature Neonates. Children (Basel) 6(11): 118.
- Long JY, Guo HL, He X, Ya-Hui Hu, Ying Xia, et al. (2021) Caffeine for the Pharmacological Treatment of Apnea of Prematurity in the NICU: Dose Selection Conundrum, Therapeutic Drug Monitoring and Genetic Factors. Front Pharmacol 12: 681842.
- Moschino L, Zivanovic S, Hartley C, Trevisanuto D, Baraldi E, et al. (2020) Caffeine in preterm infants: ¿where are we in 2020? ERJ Open Res 6(1): 00330-2019.
- Davis PG, Schmidt B, Roberts RS, Lex W Doyle, Elizabeth Asztalos, et al. (2010) Caffeine for Apnea of Prematurity trial: benefits may vary in subgroups. J Pediatr 156(3): 382-387.
- Jensen EA (2020) What is bronchopulmonary dysplasia and does caffeine prevent it?. Semin Fetal Neonatal Med 25(6): 101176.
- Davidson LM, Berkelhamer SK (2017) Bronchopulmonary Dysplasia: Chronic Lung Disease of Infancy and Long-Term Pulmonary Outcomes. J Clin Med 6(1): 4.
- Steyerberg EW, Harrell FE Jr, Borsboom GJ, Eijkemans MJ, Vergouwe Y, et al. (2001) Internal validation of predictive models: efficiency of some procedures for logistic regression analysis. J Clin Epidemiol 54(8): 774-781.
- Steyerberg EW, Harrell FE Jr (2016) Prediction models need appropriate internal, internal-external, and external validation. J Clin Epidemiol 69: 245-247.
- Dai D, Chen H, Dong X, Jinglong Chen, Mei Mei, et al. (2021) Bronchopulmonary Dysplasia Predicted by Developing a Machine Learning Model of Genetic and Clinical Information. Front Genet 12: 689071.
- Shim SY, Yun JY, Cho SJ, Kim MH, Park EA, et al. (2021) The Prediction of Bronchopulmonary Dysplasia in Very Low Birth Weight Infants through Clinical Indicators within 1 Hour of Delivery. J Korean Med Sci 36(11): e81.
- Ryan SW, Wild NJ, Arthur RJ, Shaw BN (1994) Prediction of chronic neonatal lung disease in very low birthweight neonates using clinical and radiological variables. Arch Dis Child Fetal Neonatal Ed 71(1): F36-F39.
- Valenzuela-Stutman D, Marshall G, Tapia JL, Gonzalo Mariani, Aldo Bancalari, et al. (2019) Bronchopulmonary dysplasia: risk prediction models for very-low- birth-weight infants. J Perinatol 39(9): 1275-1281.
- Gursoy T, Hayran M, Derin H, Ovali F (2015) A clinical scoring system to predict the development of bronchopulmonary dysplasia. Am J Perinatol 32(7): 659-666.
- Jassem-Bobowicz JM, Klasa-Mazurkiewicz D, Żawrocki A (2021) Prediction Model for Bronchopulmonary Dysplasia in Preterm Newborns. Children (Basel) 8(10): 886.
- Greenberg RG, McDonald SA, Laughon MM, et al. (2022) Online clinical tool to estimate risk of bronchopulmonary dysplasia in extremely preterm infants. Arch Dis Child Fetal Neonatal Ed. fetalneonatal-2021-323573.
- Peng HB, Zhan YL, Chen Y, Zhen-Chao Jin, Fang Liu, et al. (2022) Prediction Models for Bronchopulmonary Dysplasia in Preterm Infants: A Systematic Review. Front Pediatr 10: 856159.
-
Mounika Polavarapu PhD*, Praveen Kumar MD, Hillary Klonoff-Cohen PhD, Divya Joshi MD, Ruopeng An PhD and Karin Rosenblatt PhD. Bronchopulmonary Dysplasia Risk Prediction Models among Very Low Birth Weight Infants Receiving Caffeine Therapy. Glob J of Ped & Neonatol Car. 4(4): 2024. GJPNC.MS.ID.000592.
BPD, Prediction model, Caffeine treatment, VLBW, Prematurity, Methodology, Birth weight, FiO2 level, Medical practice, Hypotension
-
This work is licensed under a Creative Commons Attribution-NonCommercial 4.0 International License.