Research Article
Energetic and Exergetic Analysis of Refrigeration Systems with Low Global Warming Potential
José EAD Pereira1 and Cláudia SSL Casaca2*
1Department of Mechanical Engineering, ISEL - Instituto Superior de Engenharia de Lisboa, Instituto Politécnico de Lisboa, Lisbon, Portugal
2CIMOSM, ISEL–Instituto Superior de Engenharia de Lisboa, Instituto Politécnico de Lisboa, Lisbon, Portugal
Cláudia SSL Casaca, CIMOSM, ISEL–Instituto Superior de Engenharia de Lisboa, Instituto Politécnico de Lisboa, Lisbon, Portugal.
Received Date: December 05, 2022; Published Date: December 14, 2022
Abstract
The present study focuses on an energetic and exergetic analysis of several systems with different refrigerant fluids. It is intended to assess the most efficient technology for the conservation of oranges using positive temperatures with a refrigerating power of 250 kW. The differences that each refrigerant has in a refrigeration system and the impact that the destruction of exergy has on the various components that make up the systems were highlighted.
The installation with R-744 presents the highest percentage of exergy destroyed in the expansion device with 37% and the highest exergy destroyed (43%) in the condenser was presented for the system with R-717. For a refrigerating power of 250 kW at least, which is the most efficient technology for the conservation of oranges, it was inferred that the system with R-717 has the highest coefficient of performance (COP) of all the analyzed systems with 3.38 in conjunction with the highest exergy efficiency (31.1%).
Keywords:Refrigeration system; Refrigerant fluid; ODP; GWP; Energy; Exergy
Introduction
Energy resources are considered increasingly scarce, so there is a need to prioritize new and more efficient techniques. The first law of thermodynamics states that there is conservation of energy during a process and that energy can neither be created nor destroyed [1].
The second law of thermodynamics deals with the quality of energy. It focuses on the degradation of energy during a process (entropy generation) and on the loss of opportunities to perform or conserve mechanical work. The second law of thermodynamics has proved to be a relevant tool in the optimization of complex thermodynamic systems [2]. In this way, it is presented another important concept that is thermodynamic availability, also known as exergy. A change in this availability means a change in the ability of a substance to do useful work. Unavailable energy is the portion of energy that cannot be converted into useful work (even by a heat engine or reversible refrigeration machine) [1].
Evaporation and condensation temperatures also play a role in exergy performance. The higher the evaporation temperature, the greater the energy and exergy efficiency of a refrigeration system. For the condensing temperature, the opposite is evident, that is, the efficiencies decrease the higher the temperature at which the refrigerant condenses [3].
Regarding the optimization of refrigeration systems, the use of ejectors in cycles with R-744 stands out to increase energy efficiency [2]. Studies have shown that the use of ejectors makes it possible to reduce the amount of exergy destroyed in transcritical R-744 systems [2]. Another optimization technique for refrigeration systems will be the implementation of evaporative/adiabatic condensers in order to decrease the condensing temperature [4].
The present study focuses on an energetic and exergetic analysis of several vapor compression systems, looking for the most efficient technology for the conservation of fruit products, namely oranges, with positive conservation temperatures for a refrigerating power of 250 kW.
Methodology and Systems
The use of various refrigerant fluids with zero ozone depletion potential (ODP) and reduced global warming potential (GWP) were studied for an installation with a refrigerating capacity of 250 kW. The European Commission presents some refrigerants that should be used in new installations or for retrofitting [5]. Table 1 shows the refrigerants with GWP below 420 (limit imposed for 2030) and zero ODP recommended by the European Commission. In addition, it was decided to analyze another refrigerant, namely R-515B, since it presents zero ODP, GWP equal to 299 and similar behavior to R-134a [6].
Table 1:Proposed refrigerants for energetic and exergetic analysis.

Figure 1:Common operating scheme (a) and Mollier diagram (b) for each refrigerant..

Figure 1 shows the operating scheme and the Mollier diagram common to all systems. The numbers presented in Figure 1 serves to understand the developed systems. Point 01 represents the saturated steam still in the evaporator while point 001 involves the useful superheating performed still in the evaporator. Number 1 counts the non-useful overheating that occurs in the suction pipes. Points 2 and 2S represent the actual and isentropic discharge values respectively. Number 03 appears at the condenser outlet while point 3 accounts for the subcooling process. Finally, point 4 is after the expansion device.
With the help of Bitzer Software it was possible to select the necessary compressors for the correct operation of each system [7]. Through this software it was possible to determine the isentropic performance of each. Table 2 presents the selected compressors, as well as some important characteristics for thermodynamic analysis, such as the isentropic efficiency and the respective compression power.
Table 2:Compressors selected for each system, depending on the refrigerant.
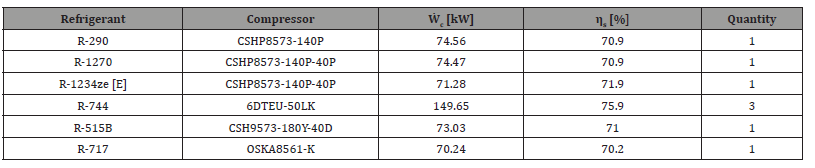
Other important conditions considered in the sizing of the systems were the ambient design, condensation, evaporation, hot and cold source temperatures. For the case developed, it was proposed that the ambient design temperature, 𝑇0, be around 31.8 °C. This value was determined for the city of Faro, in Portugal, with the help of the Software Hourly Analysis Program, provided by ©Carrier and values provided by the National Civil Engineering Laboratory [8].
Conservation at positive temperatures was typified using oranges as a fruit product, with Table 3 showing the main conservation characteristics.
Table 3:Recommended conservation characteristics for oranges [9].

The temperature difference range obtained in the cooling unit varies depending on the application. In wet storage, temperature differences of around 4 K can be used, while for dry storage, differences of 15 K can be verified [10].
The smaller the temperature difference, the smaller the dehumidifying effect caused by the cooling coil. Very high relative humidity (around 90%) show a temperature difference of the order of 4 to 6 K [10]. As the optimal conservation humidity varies between 90% and 95%, it is considered that the evaporation temperature, TE, must be 4 °C lower than the lowest conservation temperature of the fruit, that is, approximately 0 °C.
The refrigerant condensing temperature, as a rule, is between 6 °C and 20 °C above the temperature of the air that comes into contact with the coils [11]. Thus, it was decided to add the average of these values with the ambient design temperature value, resulting in a condensation temperature, TCS, of approximately 45 °C.
For the cold source temperature, TC, 7.22 °C was considered, that is, the highest conservation temperature for oranges, and the hot source temperature, TH, a value of 33 °C was used. Table 4 summarizes these values.
Table 5 presents the systems under study and their characteristics depending on the refrigerant used.
Table 4:Relevant temperatures for exergy analysis.

Table 5:Description of each system depending on the refrigerant.

Thermodynamic Analysis
In compliance with the first law of thermodynamics, an energy analysis was carried out, determining certain parameters to characterize the system. For the determination of the mass flow, m , which circulates in the systems, Equation 1 was used, where the desired cooling capacity, e Q , was considered.

For the compression power, Wc , Equation 2 is presented.

The heat rejected by the condensing unit, Qc , can be found through Equation 3.

The 𝐶𝑂𝑃 is revealed as the quotient between the cooling power and the compression power which, in other words, represents energy efficiency, ηI .

Regarding the second law of thermodynamics, the exergy destroyed in compressors and expansion devices, ψD , can be determined by Equation 5 through the product between the dead state temperature, 𝑇0, and the entropy generated in each process,s g

To calculate the exergy destroyed in evaporative equipment,
ψ


The exergy flow, Δψ , that passes through an equipment or system represents the maximum amount of useful work produced (or the minimum amount of useful work consumed in the case of equipment that absorb energy, such as pumps or compressors).

Knowing the exergy destroyed and the exergy flow that passes through the equipment, it is possible to determine the exergy yield, II η , for the evaporator and condenser (Equation 9).

For the exergy efficiency of the compressor, IIc η , the quotient between the exergy flow and the compression power must be determined, as explained in Equation 10.

In the case of the expansion valve, the performance is zero since the main function of this equipment is to destroy the potential energy convertible into work. It is necessary to know the 𝐶𝑂𝑃 of Carnot, Equation 11, for the determination of the exergy yield, using Equation 12.

Results and Discussion
Through the data collected during the selection of compressors and the sizing of each system, it was possible to determine, using Equations 1, 2, 3, and 4, the COP of each system depending on the refrigerant. Of all the systems, it is evident that the one using the R-717 exhibits the highest COP with 3.38.
Figure 2:COP of systems depending on refrigerant.
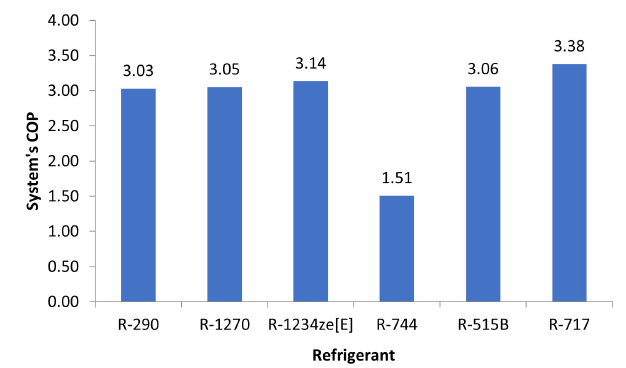
One of the most important factors to highlight from this analysis is the impact that temperatures have on the exergy yield, regardless of the equipment or system. Take the explicit example of Figure 3, where it is possible to observe the variation of the exergy efficiency of an evaporator depending on the cold temperature. If the cold temperature is equal to the evaporation temperature, the exergy efficiency of the unit will be equal to 100% since there are no heat exchanges considering that the temperatures are the same. On the other hand, if the cold source temperature is equal to the dead state temperature (which in this case is the design ambient temperature) then the equipment efficiency will be zero given that the useful work potential of a dead state system is equal to zero. Thus, it appears that there is an applicable range of temperatures for calculating the exergy yield of the systems (Figure 3).
Figure 3:Exergy efficiency of an evaporator depending on the temperature variation of the cold source.<,/p>
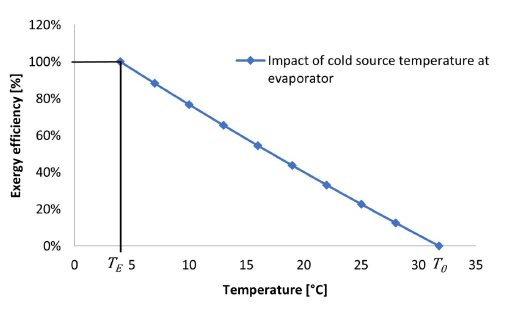
Figure 4:Exergy destroyed in each system, depending on the refrigerant<,/p>
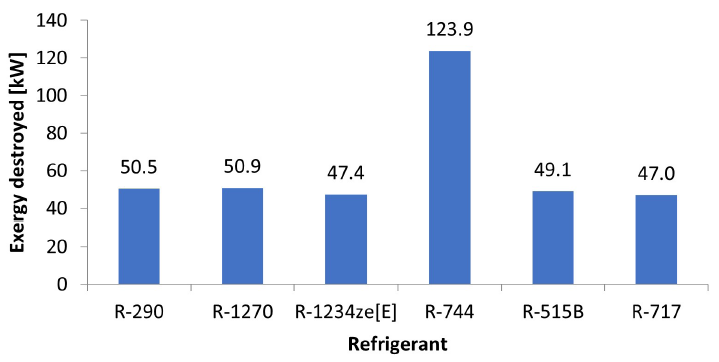
In the case of condensers (or Gas Coolers in the case of systems with R-744), the temperature range is between the dead state temperature and the temperature of the hot source. Note that the hot source temperature cannot be higher than the condensing temperature, as in this situation the system does not have the capacity to reject heat to the surrounding environment. The results of the exergetic analysis reveal that the systems present different results among themselves, despite the condensation and evaporation temperatures remaining unchanged (as well as the hot and cold source temperatures). It shows that the thermodynamic characteristics of each refrigerant play a key role in the behavior of each system. Of all refrigerants, the R-717 stands out with the lowest exergy destroyed with 47 kW in contrast to the 123.9 kW destroyed by R-744, as shown in Figure 4.
The exergy values destroyed in each equipment are shown in Figure 5. The results obtained for R-290, R-1270, R-1234ze[E] and R-515B are identical to each other. For compressors there is a destroyed exergy between 38% and 40%, for evaporators between 14% and 15%, for condensers between 26% and 28% and for expansion devices between 18% and 19%.
Figure 5:Exergy destroyed in each component depending on the refrigerant used/p>
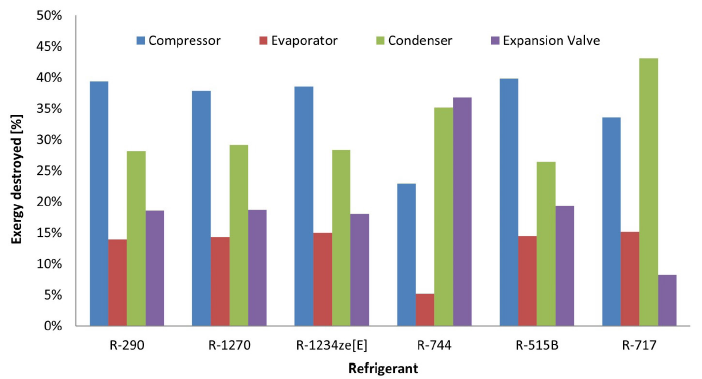
The biggest disparities are revealed in refrigerants R-744 and R-717. In case of R-744, there is the highest exergy destroyed among all refrigerants in the expansion device with 37%. This is one of the main reasons why studies were developed on the impact of ejectors and expanders for R-744 to reduce the potential for degraded useful work in the system [2]. It is evident that for each refrigerant there must be a different approach to maximize its efficiency. In contrast, the system with R-717 has the lowest exergy destroyed in the expansion device, but has the highest exergy destroyed in the condenser with 43%.
This analysis justifies the reason for using evaporative condensers in R-717 systems with the intention of lowering the condensing temperature. The lower the condensing temperature, the greater the energetic and exergetic efficiency of the system [4]. Regarding the exergy yield of the systems (Table 6), the system with R-717 stands out with 31.1% and that of R-744 with 13.9%. It is evident that the most efficient system, for the range of temperatures under study, both in terms of energy and exergy, is R-717.
Table 6:Exergy efficiency of the various systems and main components..

In the case of the exergy efficiency of each component, there is no evidence of major discrepancies between the refrigerants except for, once again, R-744 and R-717. In this case, the R-744 presents the best exergy efficiency in the compressor and in the evaporator with
81.1% and 77.4, respectively followed by the system with R-717 with 77.7% in the compressor and 75.4% in the evaporator. On the other hand, the two systems present the worst exergy performance in the condenser due to the high discharge temperatures that characterize both refrigerants.Conclusion
TDuring the analysis carried out on vapor compression systems for the conservation of fruit products with positive conservation temperatures and cooling capacity of 250 kW, it is inferred that the system with R-717 presents the best energy and exergy performance of the remaining systems with different analyzed refrigerants with 3.38 and 31.1%, respectively.
The R-717 system exhibits the lowest exergy destroyed with 47 kW, while the R-744 system has a higher 76.9 kW amount of exergy destroyed.
The exergy destroyed in the various components that make up each system allows inferring that each refrigerant has different thermodynamic properties, highlighting R-717 and R-744. In the case of R-717, the expansion valve represents only 8% of the 47 kW destroyed in this system, while the condenser represents 47%. In relation to R-744, the exergy destroyed in the expansion valve takes values around 37%, so it makes sense to use ejectors or expanders for the expansion process to increase the exergy efficiency of the systems.
In this way, the system with R-717 is the choice that stands out as the most efficient technology for the conservation of oranges. To enhance the efficiency of this system, an evaporative or adiabatic condenser could be used in order to lower the condensing temperature with the disadvantage of water consumption involved. In addition, one must consider the fact that R-717 is a toxic and flammable refrigerant that has a corrosive behavior in contact with equipment made of copper.
Acknowledgement
None.
Conflict of Interest
No conflict of interest.
References
- MA Boles, YA Çengel (2009) Thermodynamics An Engineering Approach, Mc Graw-Hill.
- JYGY Tao Bai (2016) Advanced exergy analyses of an ejector expansion transcritical CO2 refrigeration system. Energy Conversion and Management 126: 850-861.
- RSHMJU Ahamed (2011) A review on exergy analysis of vapor compression refrigeration system. Renewable and Sustainable Energy Reviews 15(3): 1593-1600.
- VK GUPTA, M PRASAD (1984) Economic Cooling Water Rate For Two-Stage R-12, R-22 And R-717 Refrigerating systems.,” Heat Recovery Systems 4(3): 141-148.
- European Commission “Climate-friendly alternatives to HFCs,” European Commission.
- Honeywell (2021) SOLSTICE N15 (R-515B) - Technical Data Sheet.
- Bitzer, Bitzer Software, v6.17.8: Rev2725.
- Carrier, Hourly Analysis Program, v4.80.
- Engineering ToolBox (2002) Fruits and Vegetables - Optimal Storage Conditions. Engineering ToolBox.
- ASHRAE (2018) Handbook - Refrigeration (SI Edition), Atlanta: ASHRAE.
- H Acül (2008) Air Cooled Condensers and Their Effect on Energy Efficiency. FRITERM.
-
José EAD Pereira and Cláudia SSL Casaca*. Energetic and Exergetic Analysis of Refrigeration Systems with Low Global Warming Potential. Glob J Eng Sci. 10(4): 2022. GJES.MS.ID.000744.
-
Energetic, Exergetic, Refrigeration systems, Global Warming, Thermodynamics
-
This work is licensed under a Creative Commons Attribution-NonCommercial 4.0 International License.