Review Article
Condition Monitoring of Reinforced Concrete Bridge Decks: Current Practices and Future Perspectives
Abdelhady Omar1* and Osama Moselhi2
1Concordia University, Sir George Williams Campus, Canada
2Concordia University, Canada
Abdelhady Omar, Department of Building, Civil and Environmental Engineering, Concordia University, Sir George Williams Campus, 1455 De Maisonneuve Blvd. W., Montréal, QC H3G 1M8, Canada.
Received Date: April 22, 2022; Published Date: May 16, 2022
Abstract
Reinforced concrete bridge decks deteriorate over time primarily due to increasing traffic loads, severe environmental conditions, especially in North America, and deferred maintenance. Condition monitoring of those structures in a timely manner is of a great importance for making informed decisions regarding maintenance, rehabilitation and replacement strategies to preserve their value, maintain their levels of service and safeguard against catastrophic failures. The objective of this paper is to introduce a comprehensive review of the current state of the art on condition monitoring of reinforced concrete bridge decks. Deterioration progression of bridge decks including different types of defects (i.e., cracks, corrosion, delamination, spalling, honeycomb and voids), their associated causes and effects are introduced. Commonly used non-invasive and non-destructive evaluation methods, including digital imaging, ground-penetrating radar, infrared thermography, half-cell potential, electrical resistivity, chain drag & hammer sounding, ultrasonic surface wave, ultrasonic pulse echo and impact echo, are presented with their capabilities and limitations. A bibliometric analysis was conducted to investigate the current trend in condition monitoring. The review also examines the frequency of methods used in condition monitoring and provide a classification of recent studies according to study type (e.g., field or laboratory experiment), joint use (i.e., whether study applied method as a stand-alone or hybridized it with another method) and performance (i.e., whether study investigated performance indicators of the applied methods or not). The key stages in monitoring condition states of bridge decks considering risks are discussed. Current practices, challenges and future perspectives are also highlighted.
Introduction
Civil infrastructure is vital for the quality of life and economic prosperity of any community. Bridges are critical component of that infrastructure due to their function in joining highways and cities, as well as enabling movement between different geographical areas. Due to harsh environmental conditions, such as that in Canada, cycles of freeze and thaw, exposure to de-icing salts, deferred maintenance, increasing traffic volumes and aging, those bridges deteriorate, and, if neglected, their failure can be catastrophic in terms of human life, social, environmental and economic impacts. The collapse of the Concorde Boulevard overpass onto Quebec, Canada, Highway 19 in 2006 is an example of such tragic events. In Canada, bridges experience significant deterioration levels with bridges in Quebec have the highest average age (31.0 years) followed by Nova Scotia (28.6 years). The average age of Canada’s bridges rose from 21.3 in 1985 to 24.5 in 2007; bridges have a mean useful life of 43.3 years. This means that Canada's bridges have passed 57% of their service life [1]. In addition, according to the Canadian Infrastructure Report Card [2], the percentage of bridges in fair to very poor condition has increased from 26% in 2016 to 38% in 2019, alerting transportation agencies and academia to develop smart technology-based methods and practices to ensure safety and preserve the value of these valuable assets.
Specifically, Reinforced Concrete (RC) bridge decks (hereinafter referred to as bridge decks) deteriorate much faster than other bridge elements since they are directly exposed to forces caused by traffic, ambient environmental conditions as well as measures like salting during Winter months. Also, bridge decks are one of the most important components in a bridge system as they provide driving surface and safety for bridge users. In addition, they have major consequences on the bridge's life cycle cost, since maintenance actions of bridge decks account for 50% to 85% of total bridge costs [3]. As a result, improving bridge deck Maintenance, Rehabilitation, and Replacement (MR&R) work can dramatically lower overall bridge expenditures. Condition monitoring of bridge decks becomes increasingly crucial to help highway agencies optimize planning for maintenance strategies and ensure adequate safety and serviceability. However, due to the large number of bridges, it is not feasible for transportation agencies to inspect and assess the condition of all bridges under their responsibility. In addition, the vast majority of current periodic inspections typically involve visual assessments of bridge condition conducted at uniform inspection intervals. These visual inspections are highly subjective, tedious, less reliable and can only detect surface defects. Nevertheless, Non-destructive Evaluation (NDE) methods (e.g., digital imaging, ground-penetrating radar and infrared thermography) provide enhancements to overcome the limitations of visual inspections. On the other hand, these uniform inspections are being applied to all bridges regardless of the significant variation in their age, design, condition and deterioration modes. For example, newly constructed bridges with improved design standards may be much less likely to develop serious damage than older bridges that has less effective durability and a lengthy exposure to harsh service environment [4].
Deterioration Progression
Bridge decks deteriorate due to a combination of factors mainly include increasing traffic loads, ambient environmental conditions, poor-construction and maintenance practices, and aging. This deterioration can lead to many types of defects, e.g., cracks, corrosion, delamination and spalling. The leading cause of deterioration, in reinforced concrete generally, is the corrosion of embedded reinforcing steel (i.e., rebars) [5]. When rebars rust, their volume increases leading to additional stresses on concrete. Such stresses lead to cracks and delamination which, in turn, propagates until it eventually causes spalling of concrete. In addition, corrosion causes the cross-sectional area of rebars to decrease and further loss of bond between rebars and concrete, which can jeopardize the overall structural integrity of the bridge. Corrosion is an electrochemical process in which charges (i.e., electrons) flow from anode (i.e., ferrous ions [Fe++] due to the oxidation of iron) to cathode (i.e., hydroxyl ions [OH-] due to reaction between oxygen and water) to form rust. In the presence of iron with different areas of energy levels, water and oxygen, reinforcing steel is most likely to corrode at a high rate. However, the passive layer (i.e., a thin oxide layer formed on rebars), provided by the high-alkaline environment of concrete (i.e., pH of 13 to 13.5 in case of sound concrete), reduces the corrosion rate significantly [6]. As such, the passive corrosion rate of steel in concrete is typically 0.1 μm/year; without that passive film, reinforcing steel will corrode at rates at least 1,000 times higher [6]. A decrease in concrete alkalinity or presence of considerable concentrations of chloride ions, on the other hand, might disrupt that passive layer. Most concrete combinations include a modest quantity of chlorides; however, the most harmful source of chlorides in concrete are the de-icing salts used in Winter season as a maintenance measure [7]. Acid attack and carbonation can also cause a drop in the pH of the concrete pore solution. As such, when carbon dioxide neutralizes the alkaline pore solution, the passive layer is effectively destroyed once the carbonation depth approaches that of the embedded reinforcement. However, carbonation-induced corrosion is not as common as corrosion induced by chloride ions [6]. Successive freeze-thaw cycles can also increase the risk of corrosion in concrete. For more information, the reader may refer to ACI Committee 222 [6]. It is worth pointing out that delamination and cracking can also be stress-induced from applied loads rather than being corrosion-induced. Other defects such as: subsidence cracks, surface air voids or subsurface voids and honeycomb may be present in bridge decks due to improper design and construction practices, e.g., highly congested reinforcement, low concrete workability and/or segregation during concrete placing. For more information, the reader may refer to ACI Committee 309 [8]. All these defects cause bridge decks to degrade continuously leading to unacceptable Levels of Service (LoS) and increase the risk of failure (likelihood and/or consequences). Causes, effects and interrelationships of common defects in bridge decks are depicted in Figure 1.
NDE Methods
Inspections are essential to assess the condition of highway bridge decks and maintain their safety and serviceability. In addition, monitoring the condition of bridge decks over time has become crucial as it assists in determining the optimal intervention plan and preserve resources. Visual Inspection (VI) is simple but not sufficient as it is subjective, tedious, error-prone and limited to only surface defects. NDE methods, on the other hand, are non-invasive advanced inspections that do not affect the integrity of the member under evaluation. They have different capabilities in detecting surface defects (e.g., cracking, spalling, scaling and pop-outs) and subsurface defects (e.g., corrosion, delamination, voids and honeycomb). NDE methods can be categorized into five main groups as shown in Figure 2: digital imaging, electro-magnetic (e.g., ground-penetrating radar), thermal (e.g., infrared thermography), electro-chemical (e.g., half-cell potential and electrical resistivity), and acoustic (e.g., chain dragging & hammer sounding, ultrasonic surface wave, ultrasonic pulse echo and impact echo). For more information about defects and NDE methods for highway bridge decks, the reader may refer to Abdelkhalek and Zayed [9], Yehia, et al. [10], Omar and Nehdi [11], Abdelkhalek and Zayed [12], Gucunski, et al. [13]. A brief description of these methods is presented through the following subsections. A summary of their strengths and weaknesses is also provided afterwards in Table 1.
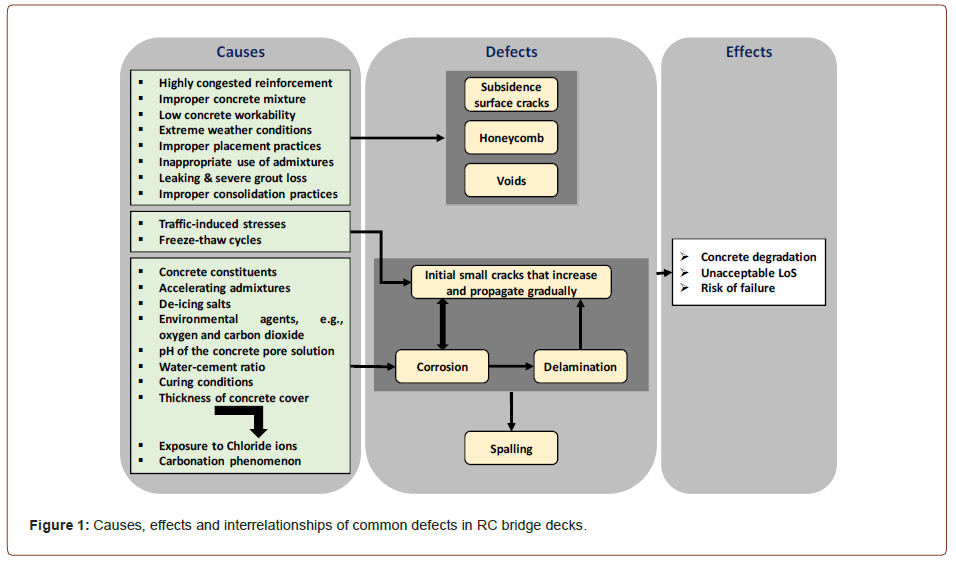
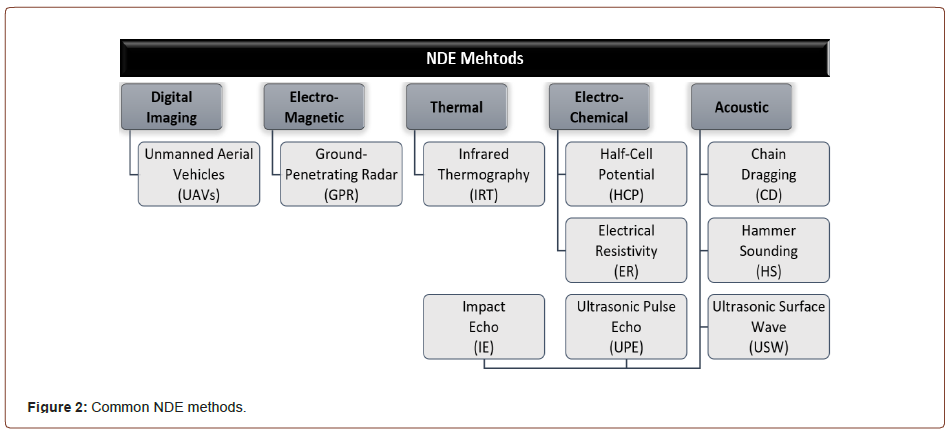
Digital Imaging (DI)
A significant number of inspection images are generated during inspection, due to the wide development and application of visual inspections for civil infrastructures, such as CCTV robots and Unmanned Aerial Vehicles (UAVs). Manual interpretation to get inspection results of these images is required; however, this process is inefficient and ineffective. During last decade, many computer vision techniques have been developed to overcome the limitations of such manual interpretation. Generally, computer vision methods include: image acquisition, image pre-processing, image segmentation, feature extraction, object recognition and structural analysis [14].
For civil infrastructure inspection, various vision-based tasks have been investigated, including automated detection and dimension measurement of concrete cracks, spalling as well as other surface defects [15-20], recognition of damage pattern changes and 3D visualization of cracks [21], and precise crack extraction with lower model computation cost [22]. Segmentation and classification of sewer pipe images to get joints, laterals, and faults for condition evaluation, have also been applied [23-26].
Ground Penetrating Radar (GPR)
GPR is capable of detecting shallow and deep delamination, voids, honeycomb and rebar configuration. It is a rapid technology that employs electromagnetic waves to identify items buried inside structures and create contour maps of subsurface features such as steel reinforcements in reinforced concrete bridge decks. Varying frequency antennas of different types are used to allow different levels of detail and penetration depth. A GPR antenna sends high-frequency electromagnetic waves through the deck or structure. Any reflector, such as rebar (or any other abnormality), reflects a part of the energy back to the surface, where it is collected by the antenna. The remaining GPR energy percolates under this interface, and more energy is reflected back to the receiver from other interfaces until it is diminished [27-29].
Infrared Thermography (IRT)
IRT is an effective method in detecting flaws in concrete such as: overlay debonding and shallow delamination. Infrared (IR) cameras detect temperature variations by measuring the thermal radiation emitted by a body depending on the thermal characteristics of various materials. IRT monitors electromagnetic wave surface radiations linked to temperature fluctuations in the IR wavelength to identify subsurface faults. Variable material characteristics, such as density, thermal conductivity and specific heat capacity, can be used to identify anomalies such as voids and material changes. The resulting heating and cooling behaviour are compared with the surrounding material [30-33].
Half-Cell Potential (HCP)
HCP is a widely used method to evaluate active corrosion in reinforced concrete structures. HCP measurements can be conducted at temperatures higher than 2℃ and should be used on free concrete surface without, for example, an asphalt overlay to avoid erroneous or even impossible measurements. The basic principle is that when a metal is submerged in an electrolyte, the positive ions will resolve, resulting in an excess of negative charges due to oxidation. The positive metal ions will accumulate at the metal-liquid interface. Anions, from the electrolytic solution, are attracted to the positively charged side forming the so-called half-cell. Therefore, if two different metals are submerged into an electrolyte and connected electrically by a wire, galvanic element can be created. The more negative values are indication of corrosion activity [34, 35].
Electrical Resistivity (ER)
ER method is used for moisture and flaw detection, specifically cracks. The amount of water in concrete is an indicator of its corrosion state because the electrical conduction in concrete occurs mainly due to electrolytic current flow through the open pore system. In addition, due to increased porosity, damaged and cracked regions are preferred routes for fluid and ion movement. The higher the electrical resistivity of the concrete is, the lower the corrosion current passing between anodic and cathodic areas of the reinforcement steel will be [13, 36].
Chain Dragging (CD) & Hammer Sounding (HS)
CD and HS are the most frequent inspection procedures utilized by transportation agencies and other bridge owners for detecting delamination in concrete bridge decks. By dragging a chain along the deck or hitting it by a hammer, the sound changes from clear ringing sound (sound deck) to a somewhat muted or hollow sound (delaminated deck) which allow for the mapping of the deck condition [37, 38].
Ultrasonic Surface Waves (USW)
USW is utilized to detect overlay debonding and delamination. USW method is a part of spectral analysis of surface waves method used to evaluate material properties (e.g., elastic modulus). These surface waves are elastic waves that travel along the free surface of a medium and propagates with a velocity dependent on the elastic properties of the medium. The waves propagating in a heterogeneous medium are dispersive; waves of different wavelengths or frequencies travel with different velocities. As a result, monitoring the phase velocity versus frequency relationship can provide information about the subsurface of the bridge deck and existence of anomalies. The presence of delamination is indicated by a change in wave velocity [39, 40].
Ultrasonic Pulse Echo (UPE)
This method uses ultrasonic waves to detect objects, interfaces and anomalies. These waves are generated by exciting a piezoelectric material with a high amplitude pulse that has high voltage and current. The physical principle of this method relies upon measuring the transit time of ultrasonic waves travelling through a material and being reflected to the surface of the tested medium. Since velocity of ultrasonic waves is different in different mediums, the transit time or velocity can be used to detect internal flaws; as a small portion of the radiated energy is reflected back to the surface as the wave interacts with a flaw. Concrete areas with deterioration or cracking have lower velocity [13].
Impact Echo (IE)
IE is used for detecting defects in concrete, primarily delamination. Its physical principle relies upon striking the surface of the tested object by low frequency stress wave and measuring the response at a nearby location. By analyzing the frequency spectrum of the deck’s response to an impact, the position of the reflectors (i.e., flaws in concrete such as voids and delamination) can be identified. In case of sound deck, the dominant reflector will be at the bottom of the deck where there is a contrast in acoustic impedances between concrete and air. However, delaminated areas are recognized as shallow reflectors. In addition, other reflectors may include voids, tendons, supporting structural elements, and so forth [41, 42].
However, each method has its capabilities, drawbacks, limitations, associated uncertainty in measurement and costs. In addition, some methods can be integrated together (i.e., data fusion) to get better evaluations and interpretations. So, these methods should be strategically deployed.
Table 1:Applications, capabilities and limitations of visual inspection and NDE methods.
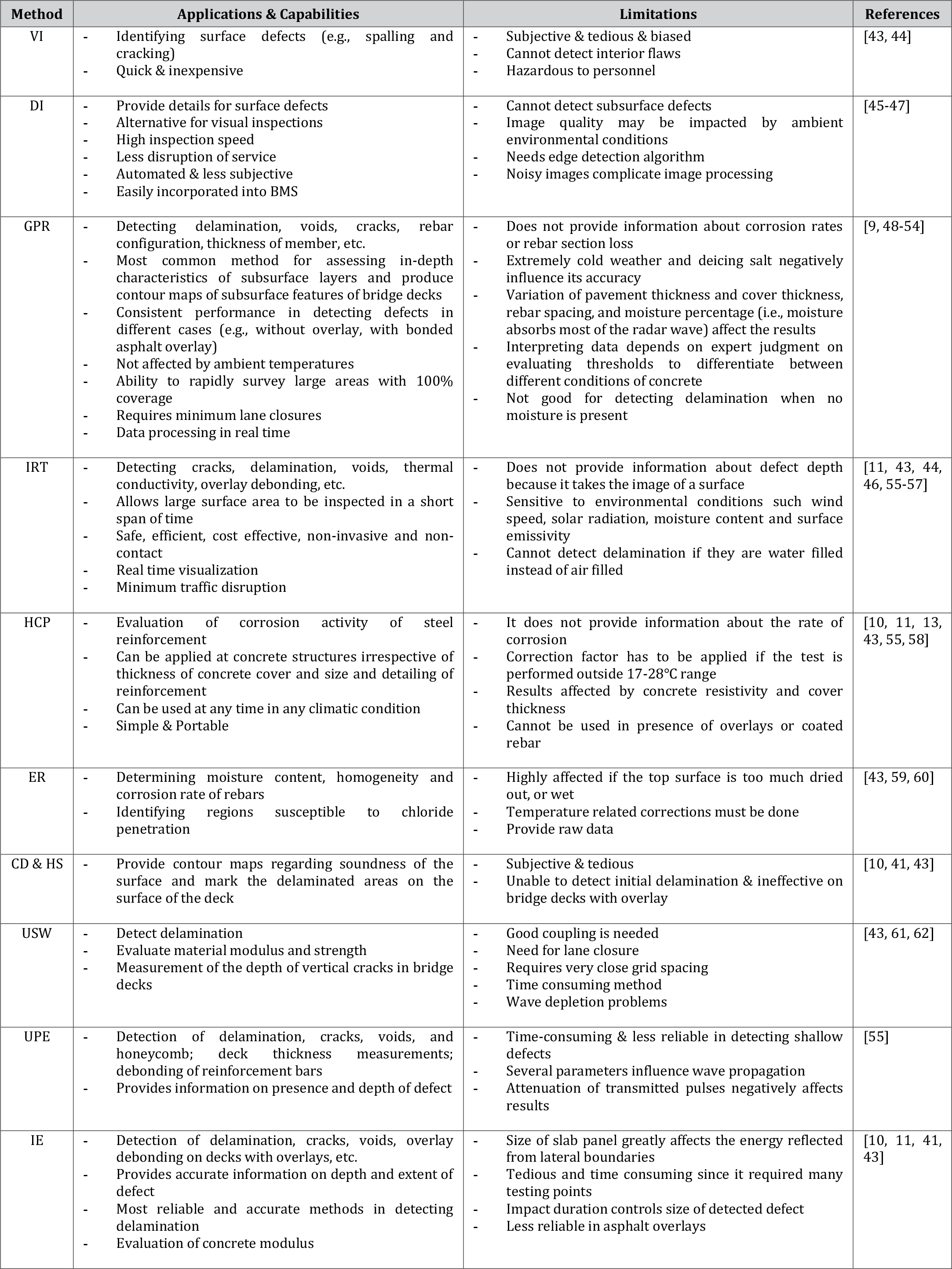
Bibliometric Analysis
In order to investigate research interest and trend in the utilization of NDE methods in condition monitoring of bridge decks, a bibliometric analysis was performed based on the Web of Science [63] database. The following keywords were used in search: “bridge AND deck AND concrete AND (inspection OR condition) AND any of (chain drag, hammer sounding, ultrasonic surface wave, ultrasonic pulse echo, impact echo, half-cell potential, electrical resistivity, ground penetrating radar, infrared thermography, digital imaging, image processing, images, non-destructive, non-invasive, visual inspection, defects, deterioration, cracks, voids, corrosion, delamination, spalling)”. In addition, the search was adjusted to account for abbreviations (e.g., NDE and GPR) and different writing styles of words (e.g., nondestructive and non-destructive & chain drag and chain dragging). Only journal articles and conference proceedings were considered over the period from 2001 to 2022. The results of the analysis are categorized and visualized as follows. Firstly, a bibliometric co-occurrence map was generated using VOSviewer [64] (a free software for constructing and visualizing bibliometric networks). This bibliometric analysis is based on a total of 563 publications. It must be noted that some keywords were matched before generating the final map for the sake of better visualization. For example, ground penetrating radar was replaced with its abbreviation “GPR”. In addition, some keywords were excluded because: their number of occurrences is less than six or they will not add any value to the map as they already understood (e.g., bridge, deck and concrete). The final map is shown in Figure 3. The typeface and circle sizes in the bibliometric co-occurrence maps indicate co-occurrences of keywords in the dataset, while colours and connections between keywords indicate their interrelatedness and clusters. It can be inferred that GPR, IRT, IE and image-based methods are the most dominant inspection methods in developed research. Corrosion, cracking and delamination are the most studied defect types. It can also be inferred that delamination is highly interdependent with GPR, IE, and IRT as indicated from network lines and colours. In addition, corrosion and cracking are more connected to strength, behaviour and durability of concrete.
For more highlighting of the frequencies in applying the specific aforementioned methods, the results of the survey were refined to include only publications related to those methods regardless of the type of defects studied. In other words, some studies that were conducted on specific type of defects (e.g., corrosion) without including any of the mentioned NDE method, were excluded. As a result, a total of 198 publications were obtained. The interest in these methods has been growing over the last two decades as depicted in Figure 4. As such, about 80% of the research in this area was done during the last decade with about 50% over the last five years. The search results were further categorized by method and country, focusing on recent work from 2016 to 2022 as shown in Figure 5. Figure 5 (a) shows the frequency of applying NDE methods in the reviewed studies. It indicates that GPR is the most investigated method followed by IRT and IE; then, DI. Figure 5 (b) depicts the relative number of the reviewed studies by country. It shows that USA has contributed the most in research on NDE methods (45% of the publications), followed by Canada (17%), South Korea (9%) and China (8%).
Employing NDE Methods in Selected Studies
A thorough review was also conducted on only journal articles related to NDE methods to investigate the scope of the study and the shortcomings in the current practices. The results of this review are summarized hereinafter to help future researchers in the field. In this review, firstly, articles published in top-notch journals were selected. Next, the NDE methods and type of defects covered by the scope of each article were highlighted. Then, they were categorized by the type of study into review studies, questionnaire surveys, field tests and lab experiments. Review articles include those publications that have been conducted to investigate the current state of the art in the field. Questionnaire surveys include studies that used questionnaires as a basis for assessing different performance parameters of NDE methods. Field tests include studies that were conducted in the field and verified utilizing core samples. On the other hand, lab experiments include studies that were conducted on specimens with simulated artificial defects. In addition, whether the methods were applied as a stand-alone method or integrated with another technology (i.e., developing hybrid systems for comprehensive monitoring of defects and increasing inspection speed and accuracy) was also recorded. Whether the study investigated the performance parameters (e.g., capability, accuracy, speed, data analysis and cost) of the methods or not was also provided. The summary of this information is provided in Table 2 and Table 3. From Table 2, it can be inferred that GPR, IE and IRT are the most applied methods in the selected studies. In addition, about 37% of the selected studies tested the potential for the joint use of multiple methods, while 22% analyzed the different performance aspects of the methods. Moreover, a total of 48% of the studies were conducted in the field, while only 28% performed in the lab, and 11% conducted in both lab and field. In Table 3, as shown, delamination and corrosion were the most studied defect types. Finally, it should be highlighted that an online sheet was established based on the information in Table 2 and Table 3 accompanied by DOIs for references, to serve as a database for future researchers. Filters can be used to facilitate search. This database is available upon request.
Condition Monitoring Stages
Condition monitoring of bridge decks passes through multiple stages. In each stage, many factors should be considered as shown in Figure 6. The first stage is data acquisition where inspectors use visual inspection and/or any of the aforementioned NDE methods to collect raw data about the current condition of bridge decks. The capability of the method to detect a specific type of defect, its accuracy and precision, service disruption and environmental conditions (e.g., temperature, moisture and visibility conditions) are among the factors that need to be considered when deciding on the most appropriate method(s). Commercial availability needs to be considered as a governing factor. In this stage, multiple methods can also complement each other to improve the condition monitoring process. The collected data are then, at times fused, processed and analyzed to get information. In data processing and analysis stage, specific algorithms, tools and extensive technical experience may be needed. Notably, the cost of data collection and analysis using NDE methods should be justified against the Value of Information (VoI) obtained to quantify their potential benefits.
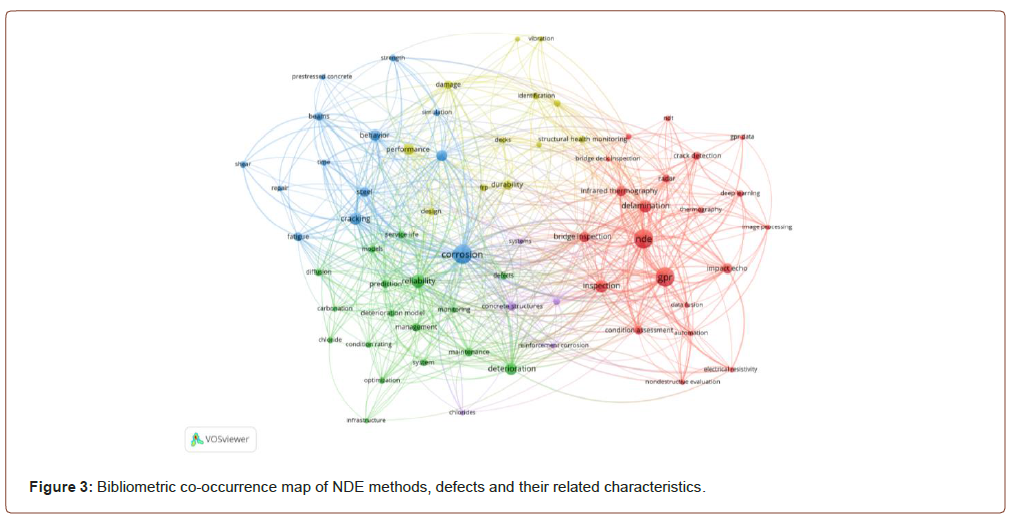
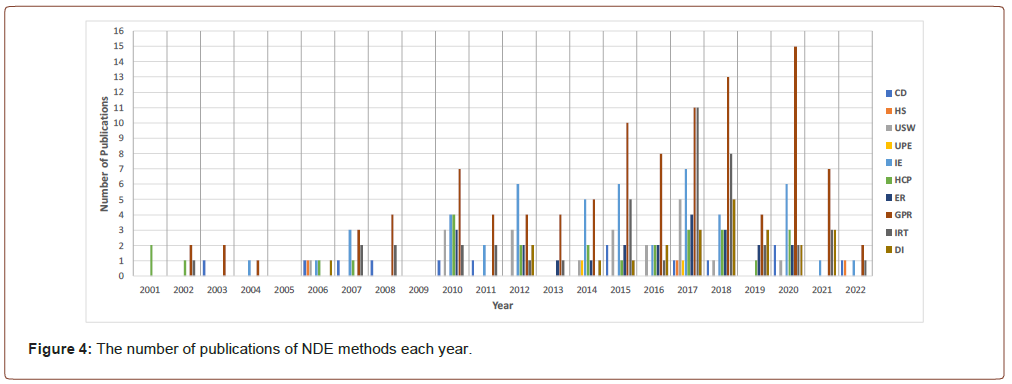
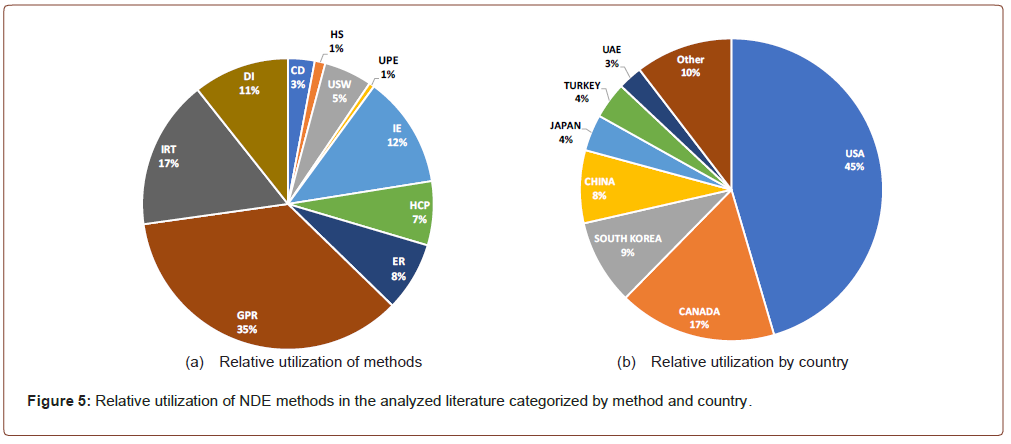
Table 2:Summary of the investigated studies including the applied NDE methods.
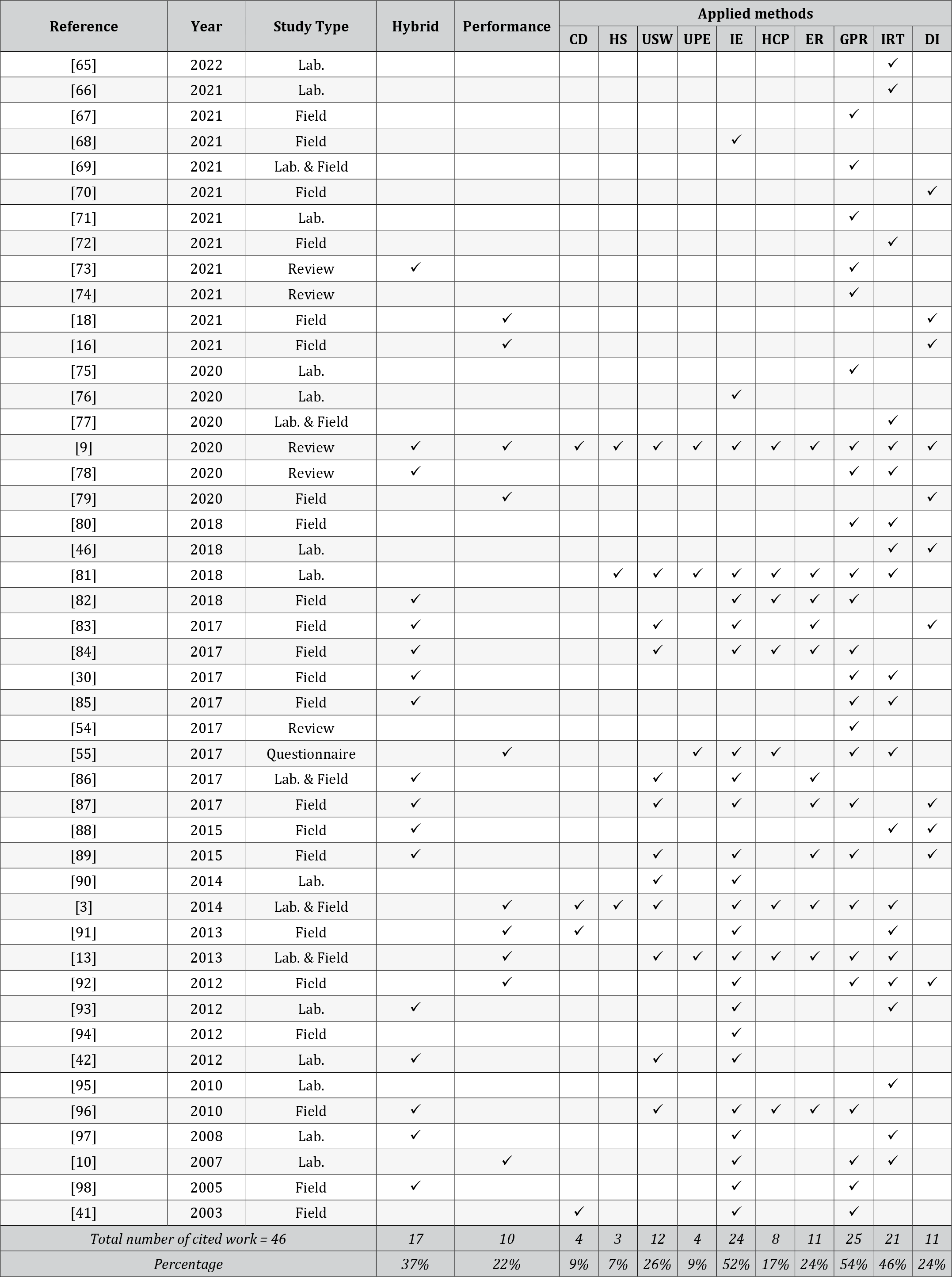
Table 3:Summary of the investigated studies including studied defects.
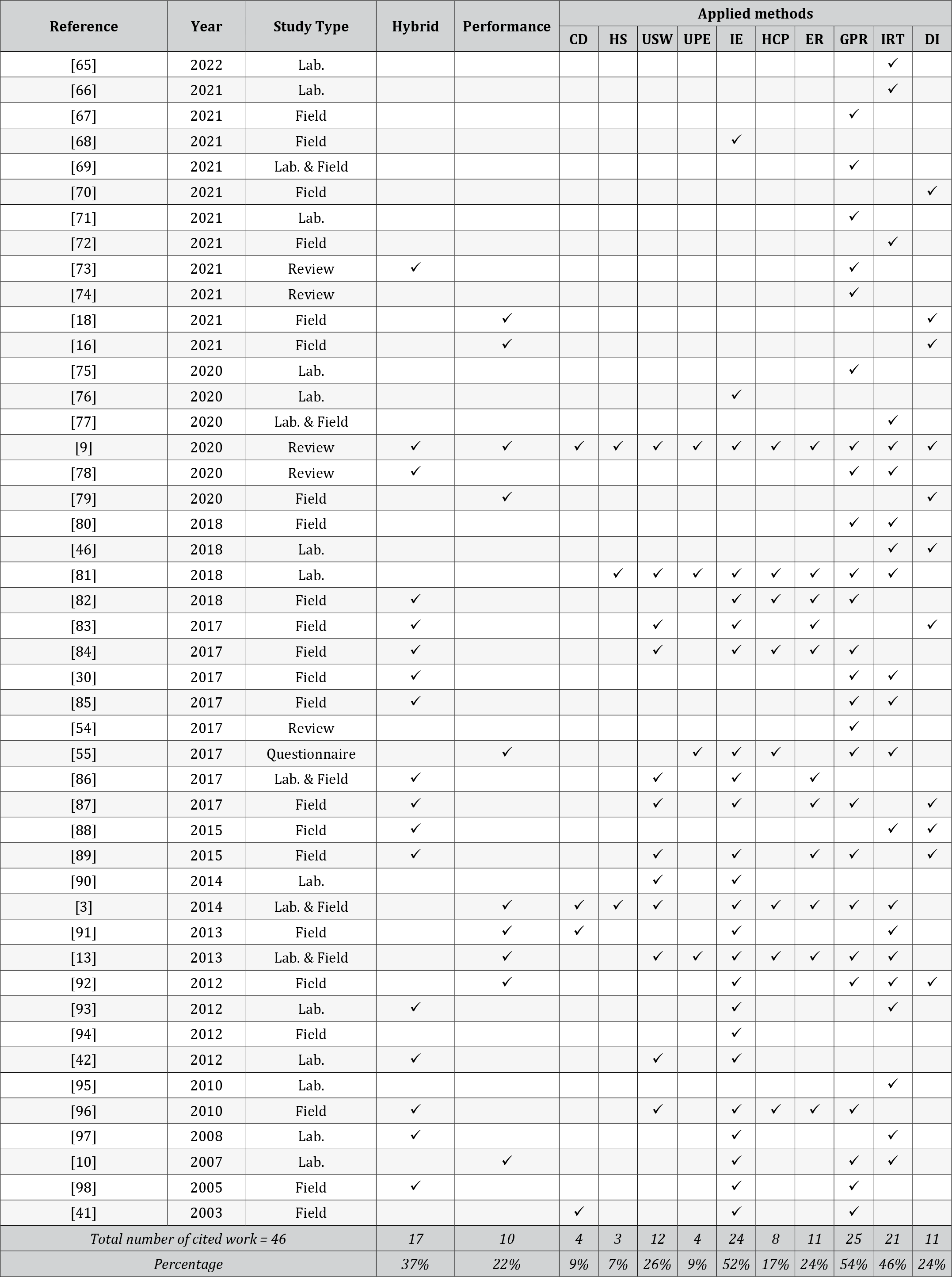
Afterwards, the developed information is visualized and interpreted to communicate the current state of the bridge deck that would trigger an intervention. The level of detail and format of the delivered information should be determined to be understandable to those without extensive experience with NDE methods. The last stage is the decision-making process that intends to achieve targeted objectives (e.g., improving condition, achieving the required LoS and reducing the risk of failure) within the planning constraints (e.g., time, budget and resources). In order to make a reliable decision, more data may be required again.
It is worth pointing out that, in the data acquisition stage, not all NDE methods are suitable for use in any phase of the bridge deck deterioration progression. As such, some methods are suitable in early phases and others are suitable in later phases as shown in Figure 7. For example, at early phases after construction, VI can be used to inspect for subsidence cracks, surface air voids and honeycomb. In addition, ER can be employed to test potential chloride penetration and corrosive environment. Next, when corrosion initiates, HCP and/or GPR can be used to investigate for corrosion existence or corrosion rate. Then, any or all of USW, UPE and IE can be employed for detecting subsurface cracks and initial delamination to medium delamination. When delamination becomes severe, CD & HS can be used. At later phases, when defects become obvious to the surface of the deck, digital images can be used to capture defects and then evaluate their severity after being processed. It can be seen that multiple methods can be used in the same phase and for the same purpose; so, the first two suggested methods in each phase are provided at the bottom of Figure 7. It is also worth noting that the deterioration phases are not sequential, but rather overlapping. As such, delamination may occur before all corrosion ends; and likewise, cracks propagate before and after corrosion.

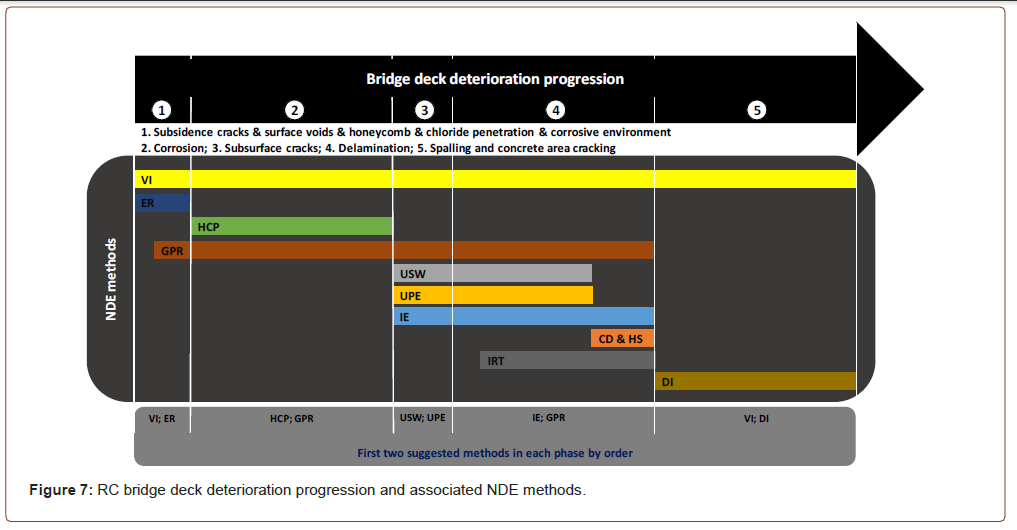
Risk-Based Condition Monitoring
Due to the importance of inspections and their impact on structure safety, serviceability and maintenance decisions, research in this field of inspection planning has been conducted in recent decades, employing various methodologies such as optimization-based methods, reliability theory, and risk analysis [99]. However, determining the inspection time and method have been recognized as a non-trivial problem with several uncertainties and contradicting objectives [100]. The current inspection standards in Canada and USA requires, for almost all bridges, a routine visual inspection should be conducted at fixed intervals regardless of condition state of a bridge, its age or deterioration modes. For example, in Quebec, Canada, three type of inspections are conducted on bridges: (i) routine visual inspection once a year, (ii) general more detailed inspection every two to four years, and (iii) special inspection as necessary [101]. Also, in USA, all bridges are required to be inspected at intervals less than 24 months; exceptions are allowed under certain circumstances according to National Bridge Inspection Standards (NBIS) [102]. Transportation agencies are encouraged to enhance the efficiency of bridge inspections by moving away from the uniform calendar-based inspections to a more rational procedure that relies upon the risks associated with the bridge condition and in-service environment [103, 104]. Optimized condition-monitoring intervals may pave the way for broader application of NDE methods in bridge inspection systems.
Determining the risk associated with each asset is a crucial step in asset management planning due to its role in prioritizing MR&R plans, in addition to creating different budget scenarios and further optimizing funding strategies. The risk of failure is the product of the consequence of failure (i.e., criticality) and the probability of failure (i.e., likelihood of a risk event to occur). This section examines the research carried out on the subject of risk-based condition monitoring. The literature reveals that little work has been done in the area of risk-based condition monitoring. Research in this area has been conducted on different types of structures including: highway bridges [4, 99, 105, 106], water distribution networks [107], sewer pipelines [108] and ships [100, 109]. Research stream in this area can be categorized based on three main concepts: expert judgement, fuzzy sets and VoI. The three categories and associated studies are presented as in Table 4. As shown in Table 4, only one study has been conducted on bridge decks. Expert judgement was utilized in a few studies to analyze the risks associated with the condition of bridge components in terms of reliability, serviceability and performance. Despite the simplicity of this approach, the results are affected by subjectivity, bias and risk attitude (e.g., risk-averse) of decision makers that may result in unnecessary inspections with costly methods. On the other hand, fuzzy sets and VoI approaches are mathematically and computationally intensive, in addition to their need to high quality data. The Probability of Detection (PoD) of the inspection method, i.e., conditional probability of detecting damage, was also introduced in some studies. The higher the accuracy of the inspection method is, the higher the PoD and hence the VoI it can give. However, higher PoD is associated with higher costs of inspection. In addition, all mentioned studies used only lognormal PoD function and have not investigated the effect of other function types.
Table 4:Summary of literature on risk-based condition monitoring.
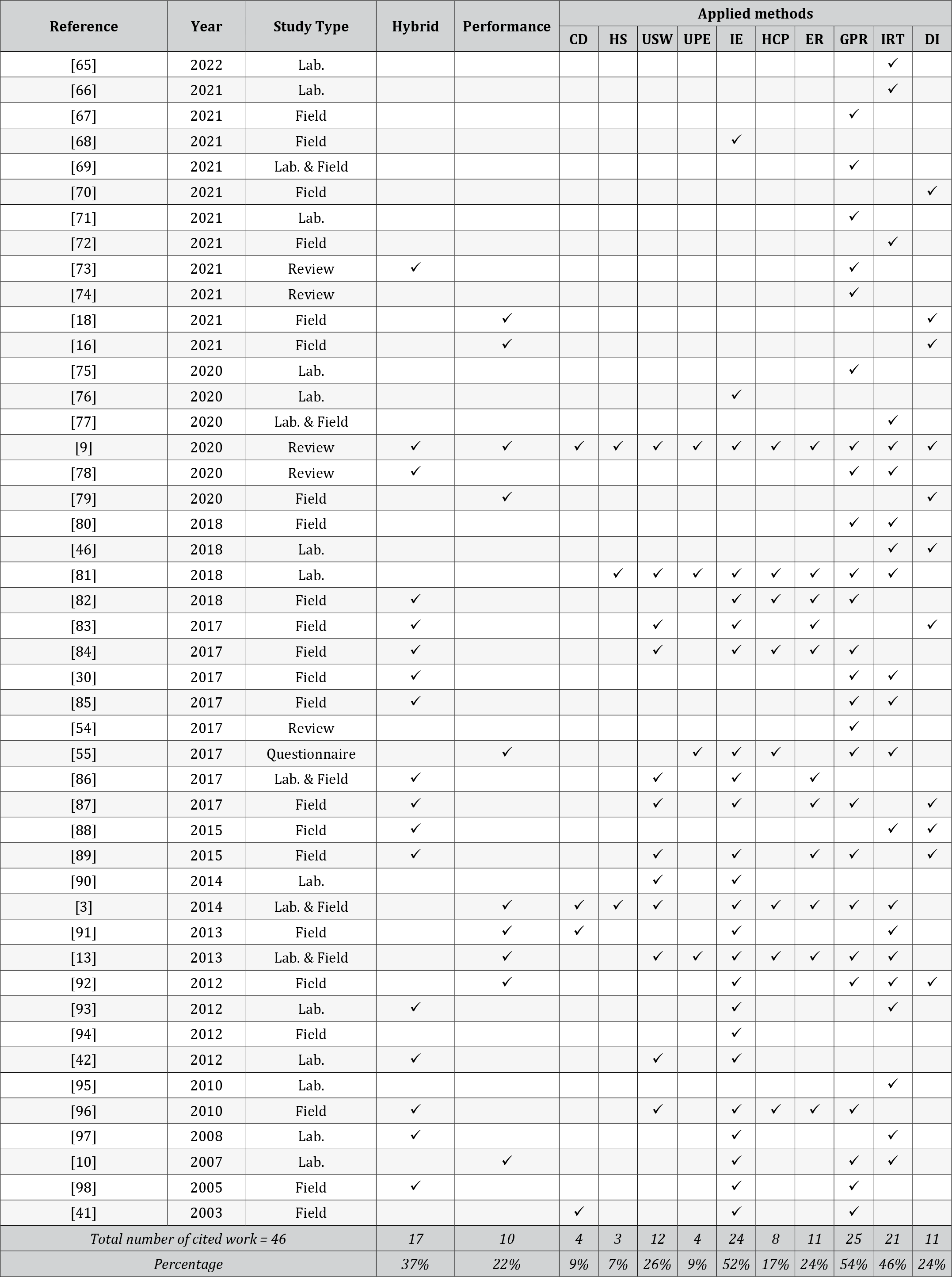
Conclusions and Future Perspectives
Condition monitoring of bridges is indispensable for making reliable intervention plans, improving bridge safety and serviceability, and preserving municipal assets. This paper introduces a comprehensive review on the current state-of-the-art literature pertinent to condition monitoring of RC bridge deck. Different types of defects, NDE methods accompanied by their capabilities and limitations, and risk-based condition monitoring are analyzed. Despite the contributions made by previous studies, there are still several limitations and areas for improvement that can be drawn as follow.
- There is a large number of deteriorating bridges with diverse characteristics and other external factors that affect their deterioration process. However, these bridges need to be prioritized for condition monitoring and, further, for intervention strategies, since it is not possible for transportation agencies to conduct condition monitoring using NDE methods for their whole inventory.
- Current research on condition monitoring utilizing NDE methods is technology focused and there is a need for a problem-based methodology to strategically deploy NDE methods. This problem-based methodology should consider three different types of features: (i) technology-related features such as capability, accuracy, speed of data collection, type and value of information obtained, need for service disruption, etc.; (ii) bridge deck-related features such as age, material, traffic volume, environmental conditions, deterioration modes, etc.; and (iii) planning-related objectives and constraints such as risk of failure, required levels of service, budget allocation and available municipal resources. In addition, costs and uncertainties associated with the application of these methods should be justified against their added value.
- Current calendar-based inspection intervals have many limitations as reported in the literature and highlighted in this review. Accordingly, a condition-driven methodology is required to determine optimal intervals. This may also assist broader application of NDE methods and reduce unnecessary inspections.
Acknowledgment
None.
Conflict of Interest
None.
Conflict of Interest
No conflict of interest.
References
- Statistics Canada (2008) Age of Public Infrastructure: A Provincial Perspective.
- Canadian Infrastructure Report Card (2019) Retrieved Oct 15, 2021.
- Gucunski Nenad, Nazarian Soheil, Imani Arezoo, Azari Hoda (2014) Performance of NDT technologies in detection and characterization of reinforced concrete deck deterioration. Paper presented at the Geo-Congress 2014: Geo-characterization and Modeling for Sustainability.
- Washer Glenn, Connor Robert, Nasrollahi Massoud, Provines Jason (2016a) New Framework for Risk-Based Inspection of Highway Bridges. Journal of Bridge Engineering, 21(4): 8.
- PCA (2002) Types and Causes of Concrete Deterioration. IS536, Portland Cement Association, Skokie, Illinois.
- ACI Committee 222 (2001) Protection of metals in concrete against corrosion.
- COST 509 (1997) Corrosion and protection of metals in contact with concrete: European Commission, Directorate-General for Research Innovation.
- ACI Committee 309 (1998) Identification and control of visible effects of consolidation on formed concrete surfaces.
- Abdelkhalek Sherif, Zayed Tarek (2018) Performance assessment model of non-destructive technologies in inspecting concrete bridge decks. Structure and Infrastructure Engineering.
- Abdelkhalek Sherif, Zayed Tarek (2020) Comprehensive Inspection System for Concrete Bridge Deck Application: Current Situation and Future Needs. Journal of Performance of Constructed Facilities, 34(5): 03120001.
- Gucunski Nenad, Imani Arezoo, Romero Francisco, Nazarian Soheil, Yuan Deren, et al. (2013) The Second Strategic Highway Research Program (SHRP 2): Nondestructive testing to identify concrete bridge deck deterioration: Transportation Research Board, WASHINGTON DC, USA.
- Omar Tarek, Nehdi Moncef L (2018) Condition assessment of reinforced concrete bridges: Current practice and research challenges. Infrastructures, 3(3): 36.
- Yehia Sherif, Abudayyeh Osama, Nabulsi Saleh, Abdelqader Ikhlas (2007) Detection of common defects in concrete bridge decks using nondestructive evaluation techniques. Journal of Bridge Engineering, 12(2): 215-225.
- Koch Christian, Georgieva Kristina, Kasireddy Varun, Akinci Burcu, Fieguth Paul (2015) A review on computer vision-based defect detection and condition assessment of concrete and asphalt civil infrastructure. Advanced engineering informatics, 29(2): 196-210.
- Abdelkader Eslam Mohammed, Moselhi Osama, Marzouk Mohamed, Zayed Tarek (2020b) A multi-objective invasive weed optimization method for segmentation of distress images.
- Abdelkader Eslam Mohammed, Moselhi Osama, Marzouk Mohamed, Zayed Tarek (2021a) Entropy-Based Automated Method for Detection and Assessment of Spalling Severities in Reinforced Concrete Bridges. Journal of Performance of Constructed Facilities, 35(1): 04020132.
- Abdelkader Eslam Mohammed, Moselh, Osama, Marzouk Mohamed, Zayed Tarek (2021b) Hybrid Elman Neural Network and an Invasive Weed Optimization Method for Bridge Defect Recognition. Transportation Research Record, 2675(3): 167-199.
- Lins Romulo Gonçalves, Givigi Sidney N (2016) Automatic crack detection and measurement based on image analysis. Ieee Transactions on Instrumentation and Measurement, 65(3): 583-590.
- Liu Chun, Tang Chao-Sheng, Shi Bin, Suo Wen-Bin (2013) Automatic quantification of crack patterns by image processing. Computers Geosciences, 57, 77-80.
- Yu Seung-Nam, Jang Jae-Ho, Han Chang-Soo (2007) Auto inspection system using a mobile robot for detecting concrete cracks in a tunnel. Automation in Construction, 16(3): 255-261.
- Adhikari RS, Moselhi O, Bagchi A (2014) Image-based retrieval of concrete crack properties for bridge inspection. Automation in Construction, 39: 180-194.
- Iyer Shivprakash, Sinha Sunil K (2006) Segmentation of pipe images for crack detection in buried sewers. Computer‐Aided Civil and Infrastructure Engineering, 21(6): 395-410.
- Moselhi Osama, Shehab-Eldeen Tariq (1999) Automated detection of surface defects in water and sewer pipes. Automation in Construction, 8(5): 581-588.
- Sinha Sunil K, Fieguth Paul W (2006a) Morphological segmentation and classification of underground pipe images. Machine Vision and Applications, 17(1): 21-31.
- Sinha Sunil K, Fieguth Paul W (2006b) Segmentation of buried concrete pipe images. Automation in Construction, 15(1): 47-57.
- Annan AP (2009) Electromagnetic principles of ground penetrating radar. Ground penetrating radar: theory and applications, 1: 1-37.
- Barnes Christopher L, Trottier Jean-François (2000) Ground-penetrating radar for network-level concrete deck repair management. Journal of transportation engineering, 126(3): 257-262.
- Maser Kenneth R (1996) Condition assessment of transportation infrastructure using ground-penetrating radar. Journal of Infrastructure Systems, 2(2): 94-101.
- Balaras C Argitiou, Argiriou AA (2002) Infrared thermography for building diagnostics. Energy and buildings, 34(2): 171-183.
- Dabous Saleh Abu, Yaghi Salam, Alkass Sabah, Moselhi Osama (2017) Concrete bridge deck condition assessment using IR Thermography and Ground Penetrating Radar technologies. Automation in Construction, 81: 340-354.
- Garrido Iván, Lagüela Susana, Arias Pedro (2018) Infrared thermography’s application to infrastructure inspections. Infrastructures, 3(3): 35.
- Washer Glenn, Bolleni Naveen, Fenwick Richard (2010a) Thermographic imaging of subsurface deterioration in concrete bridges. Transportation Research Record, 2201(1): 27-33.
- Elsener Bernhard, Andrade C, Gulikers J, Polder R, Raupach M (2003) Half-cell potential measurements–potential mapping on reinforced concrete structures. RILEM TC 154 EMC.
- Gu Ping, Beaudoin James Joseph (1998) Obtaining effective half-cell potential measurements in reinforced concrete structures (Vol. 18).
- Gucunski Nenad, Romero Francisco, Kruschwitz Sabine, Feldmann Ruediger, Parvardeh Hooman (2011) Comprehensive bridge deck deterioration mapping of nine bridges by nondestructive evaluation technologies.
- Bien Jan, Kaminski Tomasz, Kuzawa Mieszko (2019) Taxonomy of non-destructive field tests of bridge materials and structures. Archives of Civil and Mechanical Engineering, 19(4): 1353-1367.
- Zhang Gang, Harichandran Ronald S, Ramuhalli Pradeep (2012) An automatic impact-based delamination detection system for concrete bridge decks. NDT e International, 45(1): 120-127.
- Nazarian Soheil, Stokoe II Kenneth H, Hudson W Ronald (1983) Use of spectral analysis of surface waves method for determination of moduli and thicknesses of pavement systems.
- Azari Hoda, Yuan Deren, Nazarian Soheil, Gucunski Nenad (2012) Sonic methods to detect delamination in concrete bridge decks: Impact of testing configuration and data analysis approach. Transportation Research Record, 2292(1): 113-124.
- Scott M, Rezaizadeh A, Delahaza A, Santos CG, Moore M, Graybeal B, Washer G (2003) A comparison of nondestructive evaluation methods for bridge deck assessment. NDT e International, 36(4): 245-255.
- Web of Science (2022) Retrieved Apr 18, 2022.
- VOSviewer (2022) Visualizing Scientific Landscapes.
- Abdallah Abdelrahman M, Atadero Rebecca A, Ozbek Mehmet E (2021) A Comprehensive Uncertainty-Based Framework for Inspection Planning of Highway Bridges. Infrastructures, 6(2): 27.
- Yang David Y, Frangopol, Dan M. (2018) Probabilistic optimization framework for inspection/repair planning of fatigue-critical details using dynamic Bayesian networks. Computers Structures, 198, 40-50.
- Quebec Ministry of Transport (2012) Structure Inspection Manual.
- FHWA Federal Highway Administration (2021a) National Bridge Inspection Standards.
- Alampall, S, Cox WR, Healy RJ, Herrmann A, Kerley MT, et al. (2009) White Paper on Bridge Inspection and Rating. Journal of Bridge Engineering, 14(1): 1-5.
- FHWA, Federal Highway Administration (2021b) Proposed Changes to the National Bridge Inspection Standards (NBIS).
- Chung Hsin-Yang, Manuel Lance, Frank Karl H (2006) Optimal inspection scheduling of steel bridges using nondestructive testing techniques. Journal of Bridge Engineering, 11(3): 305-319.
- Washer Glenn, Connor Robert, Nasrollahi Massoud, Reising Rebecca (2016b) Verification of the Framework for Risk-Based Bridge Inspection. Journal of Bridge Engineering, 21(4): 04015078.
- Osman Hesham, Atef Ahmed, Moselhi Osama (2012) Optimizing inspection policies for buried municipal pipe infrastructure. Journal of Performance of Constructed Facilities, 26(3): 345-352.
- Elmasry Mohamed, Zayed Tarek, Hawari Alaa (2019) Multi-objective optimization model for inspection scheduling of sewer pipelines. Journal of construction Engineering and Management, 145(2): 04018129.
- Omar Tarek, Nehdi Moncef L, Zayed Tarek (2018) Infrared thermography model for automated detection of delamination in RC bridge decks. Construction and Building Materials, 168, 313-327.
- Rehman Sardar Kashif Ur, Ibrahim Zainah, Memon Shazim Ali, Jameel Mohammed (2016) Nondestructive test methods for concrete bridges: A review. Construction and Building Materials 107: 58-86.
- Hiasa Shuhei, Karaaslan Enes, Shattenkirk Wesley, Mildner Chase, Catbas F Necati (2018) Bridge inspection and condition assessment using image-based technologies with UAVs. Paper presented at the Structures Congress 2018: Bridges, Transportation Structures, and Nonbuilding Structures.
- Mohan Arun, Poobal Sumathi (2018) Crack detection using image processing: A critical review and analysis. Alexandria Engineering Journal, 57(2): 787-798.
- Morgenthal Guido, Hallermann Norman, Kersten Jens, Taraben Jakob, Debus Paul, et al. (2019) Framework for automated UAS-based structural condition assessment of bridges. Automation in Construction, 97: 77-95.
- Prasanna Prateek, Dana Kristin, Gucunski Nenad, Basily Basily B, La Hung M, et al. (2016) Automated Crack Detection on Concrete Bridges. IEEE Transactions on Automation Science and Engineering, 13(2): 591-599.
- Abouhamad Mona, Dawood Thikra, Jabri Ahmad, Alsharqawi Mohammed, Zayed Tarek. (2017) Corrosiveness mapping of bridge decks using image-based analysis of GPR data. Automation in Construction, 80: 104-117.
- Alani Amir M, Aboutalebi Morteza, Kilic Gokhan (2013) Applications of ground penetrating radar (GPR) in bridge deck monitoring and assessment. Journal of Applied Geophysics, 97: 45-54.
- Chang Che Way, Lin Chen Hua, Lien Hung Sheng (2009) Measurement radius of reinforcing steel bar in concrete using digital image GPR. Construction and Building Materials, 23(2): 1057-1063.
- Dinh Kien, Zayed Tarek (2016) GPR-based fuzzy model for bridge deck corrosiveness index. Journal of Performance of Constructed Facilities, 30(4): 04015069.
- Orlando Luciana, Pezone Alessia, Colucci Alessandro (2010) Modeling and testing of high frequency GPR data for evaluation of structural deformation. NDT e International, 43(3): 216-230.
- Washer Glenn (2003) Nondestructive evaluation of highway bridges in the United States. Non-Destructive Testing in Civil Engineering.
- Yehia Sherif, Qaddoumi Nasser, Farrag Sharef, Hamzeh L (2014) Investigation of concrete mix variations and environmental conditions on defect detection ability using GPR. NDT e International, 65, 35-46.
- Ellenberg Andrew, Kontsos Ahsas, Moon Franklin, Bartoli Ivan (2016) Bridge deck delamination identification from unmanned aerial vehicle infrared imagery. Automation in Construction, 72: 155-165.
- Omar Tarek, Nehdi Moncef L, Zayed Tarek (2017b) Performance of NDT techniques in appraising condition of reinforced concrete bridge decks. Journal of Performance of Constructed Facilities, 31(6): 04017104.
- Clemea, GG, Jackson, DONALD R, Crawford, GARY C. (1992) Benefits of using half-cell potential measurements in condition surveys of concrete bridge decks. Transportation Research Record, 46-46.
- McCann DM, Forde MC (2001) Review of NDT methods in the assessment of concrete and masonry structures. NDT e International, 34(2): 71-84.
- Ryan Eric, Burdette Edwin, Ankabrandt Ryan, Nidiffer Robert, Buchanan Brian (2014) Comparison of two methods to assess the resistance of concrete to chloride ion penetration. Journal of materials in civil engineering, 26(4): 698-704.
- Afshari AA, Frazer DG, Creese RC (2020) Ultrasonic techniques for the bonding of rebar in concrete structures Structural Materials Technology (pp. 3-8): CRC Press.
- Krause Martin, Milmann Boris, Mielentz Frank, Streicher Doreen, Redmer Bernhard, et al. (2008) Ultrasonic imaging methods for investigation of post-tensioned concrete structures: a study of interfaces at artificial grouting faults and its verification. Journal of Nondestructive Evaluation, 27(1-3): 67-82.
- Taffe Alexander (2006) Validation for thickness measurement in civil engineering with ultrasonic echo.
- Raja Babar Nasim Khan, Miramini Saeed, Duffield Colin, Sofi Massoud, Zhang Lihai (2022) Infrared thermography detection of delamination in bottom of concrete bridge decks. Structural Control and Health Monitoring, 29(3): e2886.
- Huh Jungwon, Doan Nhu Son, Lee Bang Yeon, Haldar Achintya (2021) A comprehensive study on identification of both deck and soffit defects in concrete bridge decks through thermographic investigation of shaded side under natural conditions. Construction and Building Materials, 303, 124452.
- Ata Mohamed, Abouhamad Mona, Hassanien Serror Mohammed, Marzouk Mohamed (2021) Data Acquisition and Structural Analysis for Bridge Deck Condition Assessment Using Ground Penetration Radar. Journal of Performance of Constructed Facilities, 35(5): 04021064.
- Sengupta Agnimitra, Ilgin Guler S, Shokouhi Parisa (2021) Interpreting Impact Echo Data to Predict Condition Rating of Concrete Bridge Decks: A Machine-Learning Approach. Journal of Bridge Engineering, 26(8): 04021044.
- Kumar Vivek, Morris Isabel M, Lopez Santiago A, Glisic Branko (2021) Identifying Spatial and Temporal Variations in Concrete Bridges with Ground Penetrating Radar Attributes. Remote Sensing, 13(9): 1846.
- Jeong Euiseok, Seo Junwon, Wacker James (2021) Grayscale Drone Inspection Image Enhancement Framework for Advanced Bridge Defect Measurement. Transportation Research Record, 2675(8): 603-612.
- Dinh Kien, Gucunski Nenad (2021) Factors affecting the detectability of concrete delamination in GPR images. Construction and Building Materials, 274: 121837.
- Pozzer Sandra, Rezazadeh Azar Ehsan, Dalla Rosa Francisco, Chamberlain Pravia, Zacarias Martin (2021) Semantic segmentation of defects in infrared thermographic images of highly damaged concrete structures. Journal of Performance of Constructed Facilities, 35(1): 04020131.
- Tešić Ksenija, Baričević Ana, Serdar Marijana (2021) Non-Destructive Corrosion Inspection of Reinforced Concrete Using Ground-Penetrating Radar: A Review. Materials, 14(4): 975.
- Solla Mercedes, Pérez-Gracia Vega, Fontul Simona (2021) A review of GPR application on transport infrastructures: troubleshooting and best practices. Remote Sensing, 13(4): 672.
- Abdelkader Eslam Mohammed, Marzouk Mohamed, Zayed Tarek (2020a) A self-adaptive exhaustive search optimization-based method for restoration of bridge defects images. International Journal of Machine Learning and Cybernetics, 11(8): 1659-1716.
- Dorafshan Sattar, Azari Hoda (2020) Deep learning models for bridge deck evaluation using impact echo. Construction and Building Materials, 263: 120109.
- Cheng Chongsheng, Shang Zhexiong, Shen Zhigang. (2020) Automatic delamination segmentation for bridge deck based on encoder-decoder deep learning through UAV-based thermography. NDT e International, 116: 102341.
- Dabous Saleh Abu, Feroz Sainab (2020) Condition monitoring of bridges with non-contact testing technologies. Automation in Construction, 116, 103224.
- Sultan Ali A, Washer Glenn A (2018) Comparison of two nondestructive evaluation technologies for the condition assessment of bridge decks. Transportation Research Record, 2672(41): 113-122.
- Lin Shibin, Meng Dewei, Choi Hajin, Shams Sadegh, Azari Hoda (2018) Laboratory assessment of nine methods for nondestructive evaluation of concrete bridge decks with overlays. Construction and Building Materials, 188, 966-982.
- Ahmed Marwa, Moselhi Osama, Bhowmick Anjan (2018) Two-tier data fusion method for bridge condition assessment. Canadian Journal of Civil Engineering, 45(3): 197-214.
- Kim Jinyoung, Gucunski Nenad, Duong Trung H, Dinh Kien (2017) Three-dimensional visualization and presentation of bridge deck condition based on multiple NDE data. Journal of infrastructure systems, 23(3): B4016012.
- Gucunski Nenad, Pailes Brian, Kim Jinyoung, Azari Hoda, Dinh Kien (2017b) Capture and quantification of deterioration progression in concrete bridge decks through periodical NDE surveys. Journal of infrastructure systems, 23(1): B4016005.
- Omar Tarek, Nehdi Moncef L, Zayed Tarek (2017a) Integrated condition rating model for reinforced concrete bridge decks. Journal of Performance of Constructed Facilities, 31(5): 04017090.
- La Hung M, Gucunski Nenad, Dana Kristin, Kee Seong‐Hoon (2017) Development of an autonomous bridge deck inspection robotic system. Journal of Field Robotics, 34(8): 1489-1504.
- Gucunski Nenad, Basily Basily, Kim Jinyoung, Yi Jingang, Duong Trung, et al. (2017a) RABIT: Implementation, performance validation and integration with other robotic platforms for improved management of bridge decks. International Journal of Intelligent Robotics and Applications, 1(3): 271-286.
- Vaghefi Khatereh, Ahlborn Theresa M, Harris Devin K, Brooks Colin N (2015) Combined imaging technologies for concrete bridge deck condition assessment. Journal of Performance of Constructed Facilities, 29(4): 04014102.
- Gucunski Nenad, Kee SH, La H, Basily B, Maher A, Ghasemi H (2015) Implementation of a fully autonomous platform for assessment of concrete bridge decks RABIT. Paper presented at the Structures Congress 2015.
- Azari Hoda, Nazarian Soheil, Yuan Deren (2014) Assessing sensitivity of impact echo and ultrasonic surface waves methods for nondestructive evaluation of concrete structures. Construction and Building Materials, 71: 384-391.
- Oh Taekeun, Kee Seong-Hoon, Arndt Ralf W, Popovics John S, Zhu Jinying (2013) Comparison of NDT methods for assessment of a concrete bridge deck. Journal of Engineering Mechanics, 139(3): 305-314.
- Vaghefi Khatereh, Oats Renee C, Harris Devin K, Ahlborn Theresa M, Brooks Colin N, et al. (2012) Evaluation of commercially available remote sensors for highway bridge condition assessment. Journal of Bridge Engineering, 17(6): 886-895.
- Kee Seong-Hoon, Oh Taekeun, Popovics John S, Arndt Ralf W, Zhu Jinying (2012) Nondestructive bridge deck testing with air-coupled impact-echo and infrared thermography. Journal of Bridge Engineering, 17(6): 928-939.
- Gucunski Nenad, Yan Michelle, Wang Zhe, Fang Tong, Maher Ali (2012) Rapid bridge deck condition assessment using three-dimensional visualization of impact echo data. Journal of infrastructure systems, 18(1): 12-24.
- Washer Glenn, Fenwick Richard, Bolleni Naveen (2010b) Effects of solar loading on infrared imaging of subsurface features in concrete. Journal of Bridge Engineering, 15(4): 384-390.
- Gucunski Nenad, Romero Francisco, Kruschwitz Sabine, Feldmann Ruediger, Abu-Hawash Ahmad, et al. (2010) Multiple complementary nondestructive evaluation technologies for condition assessment of concrete bridge decks. Transportation Research Record, 2201(1): 34-44.
- Cheng Chia-Chi, Cheng Tao-Ming, Chiang Chih-Hung (2008) Defect detection of concrete structures using both infrared thermography and elastic waves. Automation in Construction, 18(1): 87-92.
- Gucunski Nenad, Romero FA, Shokouhi P, Makresias J (2005) Complementary impact echo and ground penetrating radar evaluation of bridge decks on I-84 interchange in Connecticut. Paper presented at the Earthquake Engineering and Soil Dynamics.
- Li Gang, He Shuanhai, Ju Yongfeng, Du Kai (2014) Long-distance precision inspection method for bridge cracks with image processing. Automation in Construction, 41, 83-95.
- Li Mengxing, Anderson Neil, Sneed Lesley, Maerz Norbert (2016) Application of ultrasonic surface wave techniques for concrete bridge deck condition assessment. Journal of Applied Geophysics, 126, 148-157.
- Liu Hai, Lu Hantao, Lin Jianying, Han Feng, Liu Chao, et al. (2020) Penetration properties of ground penetrating radar waves through rebar grids. IEEE Geoscience and Remote Sensing Letters, 18(7): 1199-1203.
- Liu Yan, Frangopol Dan M (2019) Utility and information analysis for optimum inspection of fatigue-sensitive structures. Journal of Structural Engineering, 145(2): 04018251.
-
Abdelhady Omar* and Osama Moselhi. Condition Monitoring of Reinforced Concrete Bridge Decks: Current Practices and Future Perspectives. Cur Trends Civil & Struct Eng. 8(4): 2022. CTCSE.MS.ID.000695. DOI: 10.33552/CTCSE.2022.08.000695.
-
Reinforced concrete, Bridge decks, Rehabilitation, Traffic loads, Cracks, Corrosion, Delamination, Surface radiations
-
This work is licensed under a Creative Commons Attribution-NonCommercial 4.0 International License.