Review Article
Protective Effects of MESNA Against Carotid Artery Ischemia-Reperfusion: A Hypothesis
Merve Mercan1*, Ugochukwu Chukwunyere1, Ahmet Ozer Şehirli2 and Nurettin Abacıoğlu1
1Department of Pharmacology, Faculty of Pharmacy, Near East University, 99138 Nicosia, North Cyprus, Mersin-10, Türkiye
1Department of Pharmacology, Faculty of Dentistry, Near East University, 99138 Nicosia, North Cyprus, Mersin-10, Türkiye
Merve Mercan, Department of Pharmacology, Faculty of Pharmacy, Near East University, North Cyprus, Mersin-10, Türkiye
Received Date:December 07, 2022; Published Date:December 22, 2022
Abstract
Carotid artery ischemia-reperfusion injury is a critical disease whose prevalence is increasing worldwide. It causes intense oxidative stress resulting from the narrowing of the carotid artery due to the lack of oxygen or cholesterol. Although some surgical applications such as endarterectomy are used for its treatment, no effective pharmacological treatment has yet been found. Today, MESNA is administered intravenously to treat hemorrhagic cystitis, which is a known side effect of anticancer drugs such as cyclophosphamide and ifosfamide. According to studies, MESNA acts by first neutralizing free radicals and then eliminating the destructive effects of oxidative stress. In this regard, MESNA is proposed to have protective effects in both carotid artery ischemia-reperfusion injury and distant tissue damage.
Keywords:Ischemia; Reperfusion; MESNA; Oxidative stress
Introduction
The carotid arteries are some of the most important vessels of the body that supply adequate blood to the brain, face, and neck [1, 2]. The right and left carotid arteries split into the internal carotid artery (ICA), which supplies the brain, and the external carotid artery (ECA), which supplies the face and neck [3].
In the event of an arterial occlusion, such as an embolism, blood flow is interrupted, and tissue hypoxia occurs [4]. This condition, which initiates carotid artery ischemia-reperfusion, triggers the formation of free radicals. Free radicals are molecules or atoms that cause cell death and tissue damage. This pathological condition ultimately affects the brain, and over time, distant tissues and organs also begin to be damaged [5]. This leads to many predictable acute injuries to the heart and lungs [6, 7]. The oxidative stress that occurs during reperfusion also affects vital organs such as the brain, heart, and lungs [8]. The antioxidant and anti-inflammatory effects of MESNA may be useful in managing all these conditions [9-11].
Hypothesis
MESNA, which has been shown to protect the brain, heart, and lungs from the deleterious effects of ROS/RNS, oxidative stress, and caspase, may have protective effects against carotid artery ischemia-reperfusion.
Free radicals and ischemia-reperfusion
(ROS) and reactive nitrogen species (RNS), have powerful oxidation and nitrification activities in the human brain [12]. Increased production of ROS and RNS during cerebral ischemia-reperfusion, particularly with blood reflow, results in cell death through DNA damage, protein dysfunction, and lipid peroxidation [5].
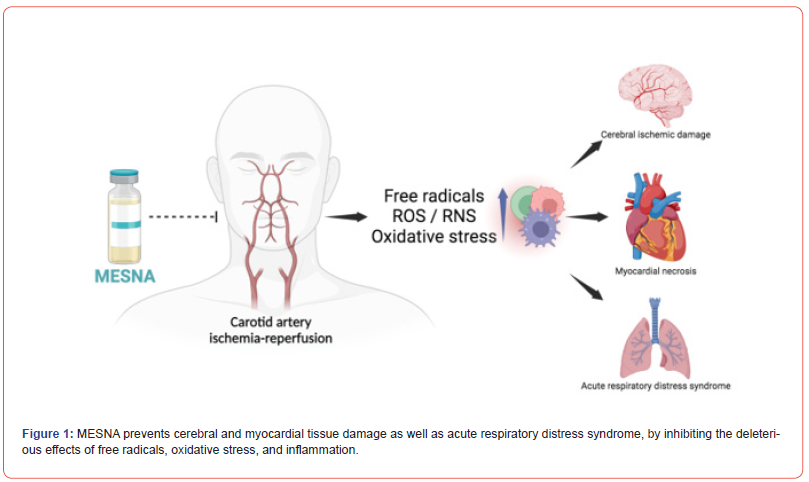
Several life-threatening disorders such as myocardial infarction, ischemic stroke, and acute kidney injury may emerge following carotid artery ischemia-reperfusion, where free radicals are prevalent [13]. Ischemia in the chronic stage can lead to carotid artery disease, which may result in interruptions in the blood flow to the brain and paralysis [14].
Carotid artery ischemia-reperfusion induced brain damage
Ischemia-reperfusion in the carotid artery reduces cerebral blood flow, and thus, the oxygen levels in some areas of the brain drop. The reperfusion that occurs after ischemia results in oxidative stress in regions with low oxygen levels. However, excessive production of ROS in cells disrupts the endogenous antioxidant system [15, 16].
Reperfusion causes oxidative stress in the region exposed to ischemia, and as a result, the blood-brain barrier is compromised [17], and cerebral edema develops over time. Cerebral ischemia, which worsens with oxidative stress, exacerbates brain injury by altering the neuro-inflammatory pathways [18, 19].
Furthermore, several enzymes are inactivated by superoxide, one of the free radicals that tends to increase during carotid ischemia-reperfusion. Cerebral ischemic damage, vasospasm following a subarachnoid hemorrhage, atherosclerosis, meningitis, stroke, and Alzheimer’s disease or vascular dementia may occur as a result of this vascular dysfunction [20, 21]. All these pathological events occur due to sudden death of brain tissues due to ischemia resulting from reduced blood flow in the carotid artery [22].
Carotid artery ischemia-reperfusion can also affect remote organs. Remote organ injury (ROI) may occur in reperfusion after local ischemia of the carotid artery [23-25]. Ischemia-reperfusion injury also disrupts organ perfusion in distant tissues, causing tissue hypoxia, resulting in cellular damage and/or cell death [26].
Carotid artery ischemia-reperfusion-induced heart and lung damages
Prolonged ischemia resulting from carotid artery ischemiareperfusion can cause myocardial necrosis and cell death due to excessive oxidative stress [27]. ROS adversely affects myocardial calcium and heart rhythm, thus leading to arrhythmia. Also, disruption of vascular blood flow can cause atherosclerosis, a chronic and progressive disease that can trigger myocardial ischemia and infarction [28, 29].
After carotid artery ischemia reperfusion, several reactive oxygen species (ROS) restrict blood flow to the lungs and impair breathing [30, 31]. High levels of ROS contribute to the advancement of acute respiratory distress syndrome (ARDS) as well as lung damage. Oxidative stress triggers the release of cytotoxic substances, which cause pulmonary edema by promoting endothelial and epithelial damage in the lungs [32]. However, different antioxidant mechanisms aid in the reduction of cell damage caused by ROS [28, 33].
Protective effects of MESNA
MESNA is a chemo-protective agent that neutralizes ROS. MESNA suppresses hemorrhagic cystitis by inhibiting the excess hydrogen peroxide produced during treatment with anticancer drugs like Oxazaphosphorine [11, 34, 35]. Because of its direct suppressive action on hydrogen peroxide generation, thiol containing MESNA might be used as an antioxidant to reduce the damaging effects of free radicals [11].
According to studies, MESNA is well tolerated by most patients and can prevent neurotoxicity as well as cell death caused by caspase activation [36]. In physiological states, superoxide dismutase (SOD), glutathione peroxidase (GPX), catalase, and other antioxidant enzymes can protect tissues from ROS cytotoxicity via catalysis, therefore maintaining a neutral equilibrium. During cerebral ischemia-reperfusion, particularly during the reperfusion phase, the generation of free radicals rise, leading to the breakdown of antioxidant systems. However, MESNA has been shown to protect the brain from severe damage due to its antioxidant and anti-inflammatory effects [37]. Furthermore, it has been shown in several studies that the removal of excess ROS from cells successfully prevents the formation of atherosclerotic plaques. Because MESNA reduces ROS, it may also suppress the formation of atherosclerotic plaques.
Conclusion
No proven preventative drugs or measures have been presented in studies until now for carotid artery ischemia-reperfusion. This review proposes that MESNA may play a key protective role in carotid artery ischemia-reperfusion, which can be attributed to its antioxidant effects. Hence, we suggest that further studies be conducted to confirm this hypothesis and extend the research.
Funding
The authors received no financial support for this review.
Ethical approval
This article does not contain any studies with animals performed by any of the authors.
Conflict of interest
The authors declare that they have no known competing financial interests or personal relationships that could have appeared to influence the work reported in this paper.
Acknowledgement
None.
References
- Choi IS (2003) Functional vascular anatomy of the head and neck. Interv Neuroradiol. 9(Suppl 2): 29-30.
- Meegalla N, Sood G, Nessel TA, Downs BW (2022) StatPearls [Internet]. StatPearls Publishing; Treasure Island (FL): Anatomy, Head and Neck, Facial Arteries.
- Sethi D, Gofur EM, Munakomi S. Anatomy (2022) Head and Neck, Carotid Arteries. [Updated 2021 Jul 26]. In: StatPearls [Internet]. Treasure Island (FL): StatPearls Publishing.
- DM Yellon, DJ Hausenloy (2007) Myocardial reperfusion injury. N Engl J Med. 357(11): 1121-1135.
- MS Sun, H Jin, X Sun, S Huang, FL Zhang, et al. (2018) Free Radical Damage in Ischemia-Reperfusion Injury: An Obstacle in Acute Ischemic Stroke after Revascularization Therapy. Oxidative Medicine and Cellular Longevity. 3804979.
- Münzel T, Camici GG, Maack C, Bonetti NR, Fuster V, et al. (2017) Impact of oxidative stress on the heart and vasculature: part 2 of a 3-part series. Journal of the American College of Cardiology. 70(2): 212-229.
- Brigham KL (1986) Role of free radicals in lung injury. Chest. 89(6): 859-863.
- CHY Wong, PJ Crack (2008) Modulation of neuroinflammation and vascular response by oxidative stress following cerebral ischemia-reperfusion injury. Med. Chem. 15(1): 1-14.
- Sener G, Sehirli O, Ercan F, Sirvanci S, Gedik N, et al. (2005) Protective effect of MESNA (2-mercaptoethane sulfonate) against hepatic ischemia/reperfusion injury in rats. Surg Today 35(7): 575-580.
- Gressier B, Lebegue S, Brunet C, Luyckx M, Dine T, et al. (1994) Pro-oxidant properties of methotrexate: evaluation and prevention by anti-oxidant drug. Pharmazie. 49(9): 679-681.
- Göksel S, Ozer Sehirli, Gözde Erkanli, Sule Cetinel, Nursal Gedik, et al. (2004) 2-Mercaptoethane sulfonate (MESNA) protects against burn-induced renal injury in rats. Burns. 30(6): 557-564.
- Sergio DM, P Venditti (2020). Evolution of the Knowledge of Free Radicals and Other Oxidants. Oxidative Medicine and Cellular Longevity. 9829176.
- Eltzschig HK, Eckle T (2011) Ischemia and reperfusion—from mechanism to translation. Nature Medicine. 17(11): 1391-1401.
- Sobieszczyk P, Beckman J (2006) Carotid artery disease. Circulation. 114(7): e244-e247.
- Chan PH (1996) Role of oxidants in ischemic brain damage. Stroke 27(6): 1124-1129.
- Chopp M, Chan PH, Hsu CY, Cheung ME, Jacobs TP, et al. (1996) DNA damage and repair in central nervous system injury. National Institute of Neurological Disorders and Stroke workshop summary. Stroke 27(3): 363-369.
- Liquan W, Xiaoxing X, Xiaomin W, Yingze Y, Zhihong J, et al. (2020) Targeting oxidative stress and inflammation to prevent ischemia- reperfusion injury. Mol. Neurosci. 13: 28.
- Jurcau A, Ardelean IA (2021) Molecular pathophysiological mechanisms of ischemia/reperfusion injuries after recanalization therapy for acute ischemic stroke. Integr. Neurosci. 20(3): 727-744.
- Lin L, Wang, X, Yu Z (2016) Ischemia-reperfusion injury in the brain: Mechanisms and potential therapeutic strategies. Pharmacol. 5(4): 213.
- Jung JE, Kim GS, Chen H, Maier CM, Narasimhan P, et al. (2010) Reperfusion and Neurovascular Dysfunction in Stroke: from Basic Mechanisms to Potential Strategies for Neuroprotection. Molecular Neurobiology. 41(2-3): 172-179.
- León-Moreno LC, Castañeda-Arellano R, Rivas-Carrillo JD, Dueñas-Jiménez SH (2020). Challenges and Improvements of Developing an Ischemia Mouse Model Through Bilateral Common Carotid Artery Occlusion. Journal of Stroke and Cerebrovascular Diseases. 104773.
- Flumignan CDQ, Flumignan RLG, Navarro TP (2017). Extracranial carotid stenosis: evidence based review. Revista do Colegio Brasileiro de Cirurgioes. 44(3): 293-301.
- Carden DL, Granger DN (2000) Pathophysiology of ischemia-reperfusion injury. J Pathol. 190(3): 255-266.
- Esme H, Fidan H, Koken T, Solak O (2006) Effect of lung ischemia-reperfusion on oxidative stress parameters of remote tissues. Eur J CardioCardio-Thoracic Surg. 29(3): 294-298.
- Santora RJ, Lie ML, Grigoryev DN, Nasir O, Moore FA, et al. (2010) Therapeutic distant organ effects of regional hypothermia during mesenteric ischemia-reperfusion injury. J Vasc Surg. 52(4): 1003–1014.
- Weyker PD, Webb CAJ, Kiamanesh D, Flynn BC (2012). Lung Ischemia Reperfusion Injury. Seminars in Cardiothoracic and Vascular Anesthesia, 17(1): 28-43.
- Kibel A, Lukinac AM, Dambic V, Juric I, Selthofer-Relatic K, et al. (2020). Oxidative Stress in Ischemic Heart Disease. Oxidative medicine and cellular longevity. 6627144.
- Michael AG, G García-Cardeña (2013) Vascular endothelium, hemodynamics, and the pathobiology of atherosclerosis. Cardiovascular Pathology. 22(1): 9-15.
- Sima P, Vannucci L, Vetvicka V (2018) Atherosclerosis as autoimmune disease. Annals of Translational Medicine. 6(7): 116.
- Willem A den H, JF Gielis, JY Lin, PE Van Schil, LJ De Windt, et al. (2010) Lung ischemia-reperfusion injury: a molecular and clinical view on a complex patho- physiological process. Am J Physiol Heart Circ Physiol. 299(5): H1283-H1299.
- de Perrot M, Liu M, Waddell TK, Keshavjee S (2003) Ischemia- reperfusion-induced lung injury. Am J Respir Crit Care Med. 167(4): 490-511.
- Kellner M, Noonepalle S, Lu Q, Srivastava A, Zemskov E, et al. (2017) ROS Signaling in the Pathogenesis of Acute Lung Injury (ALI) and Acute Respiratory Distress Syndrome (ARDS). Advances in experimental medicine and biology.967: 105-137.
- Ryrfeldt A, Bannenberg G, Moldeus P (1993) Free radicals and lung disease. Br Med Bull. 49(3): 588-603.
- Gressier B, Lebegue N, Brunet C, Luyckx M, Dine T, et al. (1995) Scavenging of reactive oxygen species by letosteine, a molecule with two blocked-SH groups. Comparison with free –SH drugs. Pharm World Sci. 17(3): 76-80.
- Umemura T, Hasegawa R, Sai-Kato K, Nishikawa A, Furukawa F, et al. (1996) Prevention by 2-mercaptoethane sulfonate and N-acetylcysteine of renal oxidative damage in rats treated with ferric nitrilotriacetate. Jpn J Cancer Res. 87(9): 882-886.
- Saadati H, Noroozzadeh S, Esmaeili H, Amirshahrokhi K, Shadman J, et al. (2020). The Neuroprotective Effect of Mesna on Cisplatin-Induced Neurotoxicity: Behavioral, Electrophysiological, and Molecular Studies. Neurotoxicity Research. 39(3): 826-840.
- Yilmaz ER, Kertmen H, Gürer B, Kanat MA, Arikok AT, et al. (2013). The protective effect of 2-mercaptoethane sulfonate (MESNA) against traumatic brain injury in rats. Acta Neurochirurgica. 155(1): 141-149.
-
Merve Mercan, Ugochukwu Chukwunyere, Ahmet Ozer Şehirli and Nurettin Abacıoğlu. Protective Effects of MESNA Against Carotid Artery Ischemia-Reperfusion: A Hypothesis. Arch Phar & Pharmacol Res. 3(2): 2022. APPR.MS.ID.000559.
-
Ischemia; Reperfusion; MESNA; Oxidative stress; Vascular dementia; Reactive oxygen species; External carotid artery
-
This work is licensed under a Creative Commons Attribution-NonCommercial 4.0 International License.