Review Article
Pharmacologic And Related Implications of a Drug Accumulation by Melanins
Maria Margarita Salazar Bookaman1* and Popat N Patil2
1Dean, College of Pharmacy. The Central University of Venezuela, Venezuela
1Professor Emeritus of Pharmacology. College of Pharmacy, The Ohio State University, Ohio
Maria Margarita Salazar Bookaman, Dean, College of Pharmacy. The Central University of Venezuela, Venezuela.
Received Date:April 14, 2023; Published Date:April 28, 2023
Abstract
Along with the microscopic morphology of iris, its relationship to melanin, the biosynthesis of the pigment and smooth muscle cells are described. The awareness of terminology like atropine, racemic hyoscyamine, the stereoselective enzymatic degradation is indicated. Localization of drugs by autoradiography in animals and that by human brain neuromelanin is presented. Importantly, pigment dependent differential mydriasis of ephedrine, cocaine, atropine by topical application in light and dark eyes of humans were analyzed. The light color eye is more sensitive to response. As compared to nonpigmented (albino) rabbit iris, the pigmented iris containing melanin accumulated and retained significantly more liposoluble drugs. Either in vivo or in vitro, antimuscarinic activity as well as duration of atropine mydriasis in rabbits was highly co-related with melanin binding of the drug. The bound drug is locally released onto muscarinic receptors of the iris sphincter. In vitro, carbachol, a quaternary drug produced identical contraction of two types of irides. Melanins from (-)-dopa, dopamine and related substances were prepared. In each melanin, the electron spin signal was observed. The binding of labeled drugs to synthetic melanins as well as the competition with various molecules was observed. The molecular steric preference for the synthetic melanin binding was small. When it was compared to (-)-ephedrine, NMR studies of soluble melanin showed higher affinity for atropine binding. (-)-Ephedrine, however, showed low preference for interaction with the soluble pigment when it was compared to its optical isomers. Depending upon the availability of the interacting site on the indol unit of the melanin up to 4 molecules can bind to the unit of the pigment. Melanin pigment from B. thuringiensis without the toxin did not interact with drugs. The importance of drug melanin accumulation in relation to retinopathy, ototoxicity, hair color as well as other conditions is discussed.
Keywords:Melanin pigment; Drug-melanin accumulation; Liposolubility, (-)-ephedrine; Cocaine; Atropine sympathomimetic amines; Ocular drug effects; Duration of mydriasis; Chronic effects; Drug toxicity; Hair colors, Bacterial melanin.
Introduction
Melanins are generally defined as pigments of brown to black color constituted by high molecular weight polymers, synthesized by oxidation of phenols. These pigments are widely distributed in nature. The origin of the pigments in evolution must be very old. In melanocytes, melanin is strongly bound to proteins. Melanins are characterized by stable free radical properties [1-4]. The term Eumelanin includes brown to black color pigment present in skin, hair, feathers, insect cuticle and melanomas. Natural pigments, violet, red to yellow color are known as pheomelanins. These melanins are also formed in melanocytes from the oxidation of tyro sine. Polymerization is catalyzed by tyrosinase. Phaeomelanin polymers contain cysteine residues [4]. Eumelanins are irregular polymers, intertwined, containing 5,6-dihydroxyindol conjugated with proteins. Rheomelanins are soluble pigments of different colors, formed from precursors like catecholamines present in the body [5]. Human substantia nigra contains neuromelanin which appears to have a different origin from eumelanin; probably it is formed from dopamine. Pigment cell melanins are also present in mammal inner ear [6]; however, its function at this level is not well elucidated.
Pharmacokinetics of the ocular medication is markedly affected by the uptake of drugs by the iris and uveal melanin. Many liposoluble pharmacologically active, topically applied or orally administered drugs are accumulated by the pigment. The accumulated drug by the pigment granule can be slowly released onto smooth muscle receptor to produce therapeutic effect. Pigmented iris or the isolated melanin granules have a high capacity to accumulate chloroquine, chlorpromazine, atropine, cocaine, nicotine, and antibiotics. The beautiful microscopic close association of the posterior heavy pigment epithelium, the low pigmented spindle cells of the anterior epithelial layer that is in contact with the dilator smooth muscle has been observed before [Figure 1].
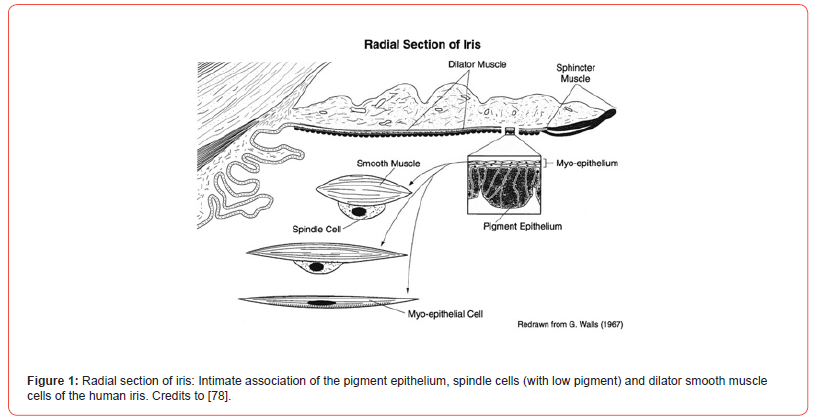
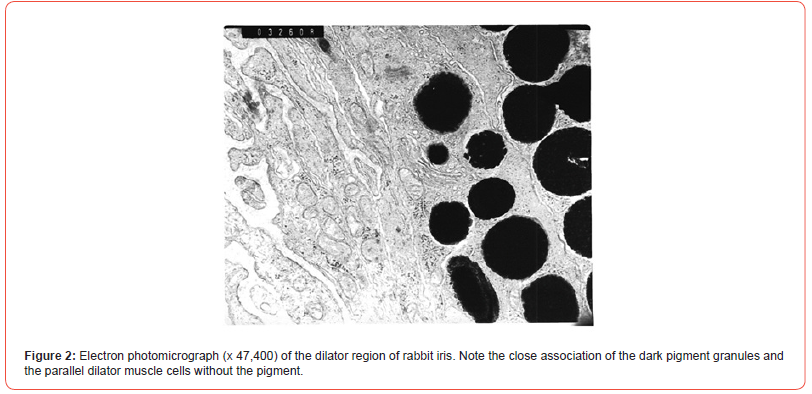
The morphological relationship between the melanin granules of the pigment cells in rabbit and human iris by Electron Microscopy (EM) was examined. The iris of the Dutch belted rabbit was processed for the microscopy by the technician in the Department of Anatomy, the Ohio State University. Ocular globes of 79-year-old diabetic male with Parkinson’s disease were donated by Arizona Lions Eye Bank, Inc., Phoenix, Arizona. The iris sphincter and dilator region were processed and examined for EM as that indicated for the rabbit iris. In both rabbits and humans, a separation of the pigment cell granules and the closely associated smooth muscle cells was visible [Figure 2].
Similar observations with photographs were made at 20 different parts of the iris. Present morphological evidence supports the slow onset of mydriatic effects of atropine or ephedrine and the long duration of action. Even though both mydriatic drugs act at morphologically distinct site and by different pharmacological mechanism of action, the pigment dependent kinetics is applicable to these and other ocular medications. The close morphological association between the pigment cell and smooth muscle cells together with the drug accumulation provide the essential understanding of the pharmacokinetic parameters. Since the human eye contains melanin, the potential drug binding to the pigment and its relevance should be studied. (P.N. Patil, Margarita Salazar-Bookaman and Hitoshi Ishikawa, Electron Microscopy studies of the iris pigment in relation to drug action. College of Pharmacy Research Day. The Poster Presentation. May 19, 2008).
Melanocytes
Melanophores in amphibians’ skin are under neuronal and hormonal control. In adults, vertebrate melanocytes show little or no response to circulating hormones. In newborn rabbits, surgical or chemical sympathectomy of iris may affect the development of melanocytes [7]. The pigmented iris binds exogenous tritium labeled norepinephrine. The uptake at low temperature was reduced indicating that it is mainly a neuronal process [8]. If pigmented and albino rabbit’s iris was uniformly labeled with 3H-norepinephrine and this labeled tissue was exposed to a depolarizing concentration of potassium, the amount of norepinephrine released from albino rabbit’s iris was greater than that released from pigmented rabbits’ iris [9]. Melanocytes do not metabolize exogenous catecholamines. In the iris of pigmented and albino rabbits, 3H-isoproterenol O-methylation rates were around 14 and 11 pmol/g/min, respectively. These products, as well as deaminated metabolites may be stored in melanocytes [10,11]. The role of melanocytes in synaptic transmission has not been established. Could the excess neurotransmitter released temporarily bind to melanocytes and from there to be released by drugs or other physiologic conditions affecting process? This important question needs to be answered.
Melanins Biosynthesis
Melanin synthesis from tyrosine occurs in premelanosomes of melanocytes. Tyrosinase is the fundamental enzyme. It is inhibited by diethyl-dithiocarbamate, a compound that acts as a copper chelating agent, while 8-methoxypsoralene increases enzyme activity. It has been used to increase skin pigmentation [12].
[Figure 3] shows the accepted scheme for Raper-Mason pathway of melanin synthesis [13] has updated the pathway. According to this, tyrosine is irreversibly oxidated to (-)-dopa, which is enzymatically transformed in dopaquinone. In the absence of enzyme, dopaquinone undergoes a spontaneous, irreversible and rapid intramolecular change leading to 4,6-dihydroxyindol-2-carboxilic acid (leucodopachrome). Under physiological conditions dopachrome is decarboxylated and it is rearranged to form 5,6-dihydroxyindol, which is rapidly oxidized to a quinone. Indol-5,6-quinone is polymerized to melanin through polyindolquinone consuming one oxygen atom [2]. In nature, several kaleidoscopic modifications have been suggested for the melanin synthesis [14-17]. Incorporation of 14C-Tyrosine in goldfish indolmelanin suggests that polymer turnover could be as long as two years [18]. In humans, chronic topical use of antiglaucoma prostaglandin like drug changes the color of dark pigmented iris. Additional enzymatic mechanism(s) in the synthesis may be involved. The role of prostaglandins in biosynthesis is implicated.
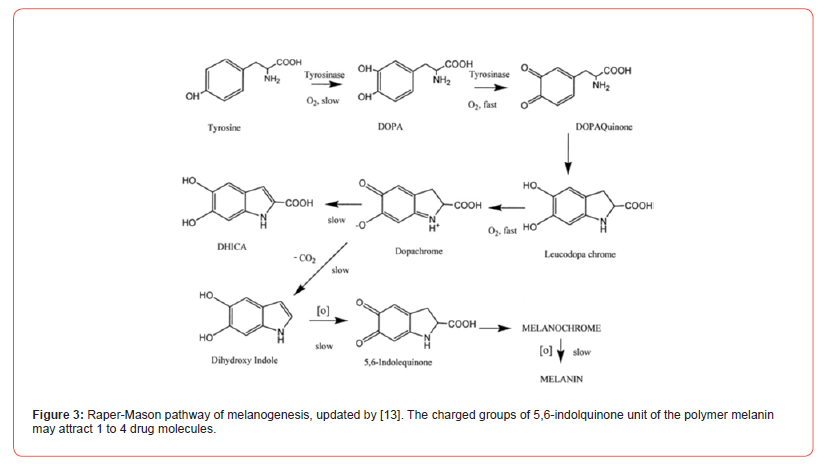
[19] indicated that phaeomelanins are formed in vivo throughout a deviation of eumelanin synthesis pathway involving a reaction between cysteine and dopaquinone. [20] suggested that there are physiological signals regulating eumelanin to phaeomelanin change and these signals are extrinsic to melanocytes. Tyrosinase is the essential enzyme for both types of melanin synthesis, but other proteins related to tyrosinase regulate eumelanin synthesis at steps distal to tyrosinase.
Synthetic melanins are amorphous substances, brown to black in color, obtained by the oxidation of the phenolic compounds. Several investigations have suggested that (-)-dopa derived melanins, natural or synthetic, are irregular polymers constituted by different types of units. According to [21] there are physical and chemical differences between (-)-dopa, dopamine and other precursors derived melanins. [22] investigated the structure using X rays diffraction techniques and he concluded that melanin has a diffuse halo that probably corresponds to an irregular orientation of aromatic groups. If natural melanins, studied in vivo, are regular polymers arranged in a specific way, drastic isolating procedures may alter structural arrangements of indol units. Melanins of high density are responsible for the contrast observed in electronic micrograph of melanin containing tissues. As in natural melanins, free radicals have also been detected in synthetic melanins [23,24] synthesized rheomelanins from several catechols found in plasma. As expected, rheomelanins also show free radical properties.
The biological significance of free radicals in melanins is still unknown. In some animal species a positive correlation between pigment intensity and free radicals’ content was reported [25-27]. Melanin free radical properties seem to be characteristic of the pigment itself since there is no evidence of presence of free radicals in the intermediate products [25]. The direct positive co-relation between the free radical property to that drug binding is unknown.
Dopamine and other substituted dopamine analogs, and by extraction from different tissues containing melanocytes, including substantia nigra of brain. When compared to the iris of albino rabbit, [28] reported a high dopamine content of the pigmented rabbit iris. The amine may function as the precursor of melanin. The binding of drugs to these polymers from different precursors are important to understand the pharmacological and toxicological aspects of the interaction with drugs.
Nomenclature: Atropa belladonna plant contains the potent antimuscarinic alkaloid (-)-hyoscyamine. Atropine is a racemic hyoscyamine. Thus, there are two molecules in atropine. The pharmacological activity is attributed mainly to the natural isomer. The radioactive labelling of the drug does not discriminate against the isomers. Furthermore, the enzymatic degradation of racemic hyoscyamine is stereospecific. So called term atropine esterase is questionable because both isomers are not hydrolyzed. In rabbits, the serum esterase will hydrolyze only (-)-hyoscyamine. Similarly, in rabbits, hydrolysis of (-)-cocaine by the enzyme 3-acylhydrolase is stereospecific [29,30]. For the sake of simplicity, we retained previous terminology in our publications and the review. In isolated tissues studied in physiological salt solution has minor drug effect of residual esterase. In rabbits, the topical mydriatic activity of atropine was markedly influenced by the presence or absence of the esterase enzyme. In humans, there is a related enzyme which metabolizes atropine. The term nonalbino refers to animals with ocular pigment. Albino animals lack melanin pigment in all organs. Dogs or humans with light colored eyes means iris has less pigment. It is not an albino iris. In the text, the terms nonalbino and albino (or nonpigmented) iris are used.
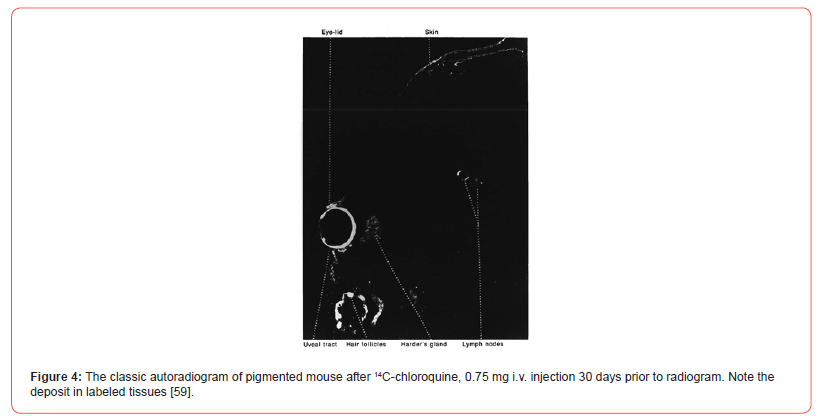
Ullberg (1958) [31] and the group [32-34] studied binding of labeled drugs to tissues in body by autoradiography techniques. These studies were done in black mice, rats, and monkeys. In the case of rodents, studies were done on pregnant and non-pregnant mice. Labeled drugs were dissolved in saline and administered intravenously in the animal tail and through femoral vein in case of a pregnant monkey. Mice were anesthetized and then sacrificed for the investigation. Mice in advanced state of gestation were sacrificed 1 hour after 35S-chlorpromazine administration and fetuses were surgically removed. Another group of mice received 14C-chloroquine and they were sacrificed afterward. The clear localization of drugs in tissues occurred. In the pregnant monkey labelled chloroquine analogue was deposited in both maternal and fetal eyes with the pigment. Specific drug deposits also occurred in mice [Figure 4].
History of Drug interaction with melanins and its relevance for drug action
Influence of human variation about pigmentation on drug action was initially suggested by [35], who observed racial differences in topical mydriatic action of several drugs. Initial mydriatic effect was greater in a group of Caucasian individuals, whereas cocaine, euphtalmine, (-)-ephedrine, (±)-ephedrine and (+)-pseudoephedrine were less active in Blacks. These results were supported by [36] who found that ephedrine was not efficacious in Black individuals while it was efficacious in Caucasians with light or clear eyes (less pigment) individuals. [37] proposed that differences in drug effects in pigmented and light eyes was due to the tyrosinase system found in pigmented eyes but not in those of albino. In albino, more neuronal norepinephrine was produced for the action. Investigators reported that poor mydriatic effect of ephedrine in pigmented eyes could be due to a low neuronal norepinephrine content, because of diversion of catecholamine precursors towards melanin synthesis. The hypothesis was questioned. [38] also reported the differential mydriatic action in human subjects of different eye color.
[39] demonstrated that in vitro without cornea albino iris of guinea pig eye was more sensitive than pigmented iris to ephedrine’s mydriatic effect [Figure 5]. Patil et al (1974) found that (-)14C-ephedrine accumulated in greater amount in pigmented guinea pig iris than in nonpigmented albino guinea pig iris. The drug disappeared faster from albino iris than that from pigmented iris. Thus, a correlation between binding of drug to the melanin pigment, a site of loss and the lower mydriatic effect was established. The differential cocaine binding to pigmented and nonpigmented guinea pig iris in vitro was also determined. Labeled cocaine accumulation in the pigment was not affected by chemical sympathectomy or by reserpine pre-treatment [40]. As compared to nonpigmented guinea pig iris, the higher accumulation of labeled (-)-norepinephrine, (±)-epinephrine, (±)-phenylephrine, (±)-octopamine, tyramine and β-phenethylamine in the pigmented iris was observed. The accumulation of some molecules was difficult to wash from the pigmented iris [41].

[24] determined affinity binding constants for sympathomimetic amines to synthetic melanin. According to their results, cocaine had the highest affinity for binding to melanin. The drug binding to synthetic melanins does not show a strict parallelism with high-capacity binding to pigmented iris. In nonphenolic amines liposolubility may play a significant role in drug accumulation in the pigmented iris. Comparing to that observed in vivo, maximum capacity for binding of drugs to synthetic melanins seems to be low.
At the Colorado Springs meeting of pharmaceutics group, Theodore Sokoloski presented a comparative attraction of drugs to synthetic melanins, melanin-granules and neuromelanins of human brain. He also compared the binding of 3H-cocaine to synthetic (-)- dopa melanin to that of natural phaeomelanin of chicken feathers. The latter was supplied by Dr. Miles R. Chedekel of the Department of Chemistry. In K-phosphate buffer, at 4 mg of synthetic (-)-dopa melanin or phaeomelanin, only 1/10th of 3H-cocaine was absorbed by the latter melanin. In other words, a very high amount of the substance was absorbed by the (-)-dopa melanin while little drug was absorbed by phaeomelanin. (T. Sokoloski, M. Salazar-Bookaman, M.R. Chedekel and P.N. Patil 1980s. Attraction of Drugs to synthetic Melanins, Melanin-Granules and Neuromelanin of Human Brain- About 5-page report).
The soluble saturated (-)-dopa melanin prepared in deuterium buffer and stored for about 4 days gave consistent specific measurements. NMR signals of aromatic and N-methyl protons of drugs were quantified in the presence of melanin. Spectral line broadening (an indication of binding) of various drug moieties occurred. Based on the line width measurements of N-methyl protons of ephedrine, two dissociation constants Kd1, 2.08 mM and Kd2 > 20 mM were obtained. The binding constants for atropine melanins complex were Kd1, 0.79 mM and Kd2 > 6mM. The latter drug has more affinity for the pigment than the former. The reactive groups of the indol unit may attract more drug molecules. These studies indicated that atropine and ephedrine compete for at least one common site of the melanin polymer [42]. Similar soluble (-)-dopa melanin in deuterium buffer was prepared and stored for 7 days. So called Kd of (-)-ephedrine was 11.7 mM. The value was higher than before. Under similar conditions, the Kd values of (+)-ephedrine, (+)-pseudoephedrine, and (-)-pseudoephedrine were about 4 mM. Thus, a 3-fold stereoselectivity for latter isomers was demonstrated. Based on stoichiometry, 2-4 molecules of the isomer were postulated to interact with the indol unit. [43]. NMR spectroscopy method is highly sensitive. It is important to note that [44] used a method to deduce that two molecules of cocaine like drugs can interact with the indol unit of hair pigment.
Bovine iris tissues (n=4) incubated with either 3x10-4M (-)-14C-ephedrine or (+)-14C-ephedrine for 15 minutes in physiological salt solution at 37.5°C, washed for 3 minutes in equal volumes of the medium, the tissue accumulated nearly equal amount of each isomer. When further washed for 60 minutes, the accumulation of (-)-14C-ephedrine was reduced by 20.5 ± 7% (S.E.M) and that of the (+)-14C-ephedrine was reduced by 28.5 ± 8% (S.E.M). The difference is not significant. Thus, under these conditions, there was little specificity in the accumulation of the optical isomers of ephedrine by the pigmented bovine iris. The lack of stereoselectivity of accumulation was like that reported for these isomers in pigmented and albino guinea pig iris. The method to detect the stereoselectivity of ephedrine isomers in the soluble melanin by NMR procedure was selective for some ephedrines. Furthermore, binding of norepinephrine stereoisomers of amphetamine to synthetic melanin show very little selectivity [41].
It is well known that chloroquine binds to melanin with a high capacity and affinity. When the drug is systemically administered, chloroquine accumulation may depend on its stereochemistry as suggested by [46]. Toxicity has been observed at retinal level after chronic administration of racemic chloroquine or hydroxychloroquine. If benefit resides in one of the isomers and toxicity in the other one, then it would be worthwhile to administer only one isomer as a therapeutic agent. This needs to be investigated.
Many β-adrenergic receptors antagonists contain an asymmetric center. The blocking action of (+)-isomer for β-adrenergic receptors is less than that of the (-) - isomer. On chronic administration, the drug is markedly accumulated and retained by the ocular pigment. A significant amount of a weakly active isomer may also be accumulated when a racemic β-adrenergic receptor antagonist is administered. The functional significance of long-term drug accumulation in the eye is difficult to quantitate.
[47] reported that when multiple timolol doses were used, a significant amount was accumulated by pigmented ocular tissues of rabbit. The blocker in the corresponding tissues of albino rabbits was very low. These authors explain the prolonged action of timolol. Also, tolerance observed during treatment with this agent probably was associated to drug accumulation in pigmented ocular tissues. Furthermore, [48] reported that in comparison to Caucasians, Black patients require larger timolol doses for reduction of intraocular pressure and a number of these patients were withdrawn from therapy due to an inadequate reduction of intraocular pressure [49]. Reported that although (+)-timolol has less affinity for β-adrenergic receptors it could be a better agent to treat ocular hypertension since it does not have side effects on the pupil size, cornea, bronchi, blood pressure or pulse rate, as it is known with the (-) - isomer. The ocular hypotensive effect of (+)-timolol was observed. The interesting observation needs to be re-examined. Since α-adrenoceptor activity is equal for isomers, the role of adrenoceptors in the regulation of ocular pressure needs to be elucidated.
When 3H-atropine accumulation in the pigmented iris was studied, the binding exceeded that observed in the iris from albino rabbit. After 10 minutes of incubation, the accumulated drug ratio of pigmented iris/non pigmented iris varied between 3 and 4-fold. Accumulation of 3H-atropine by human iris and epithelium pigment also compared favorably with pigmented rabbit iris. When 3H-atropine loss from the pigmented rabbit iris was compared to that observed a non-pigmented tissue, little labeled drug was lost from the pigmented rabbit iris for 105 minutes, whereas radioactivity was rapidly lost from albino iris with a t1/2 of ~15 minutes [50]. Note the effect of drug binding on the slope of Schild plot, [Figure 6], and the duration of action of atropine, [Figure 7]. The efflux of tritium labelled atropine from two types of irides also follows a similar pattern. The drug is rapidly lost from albino iris t1/2 ~14 mins while that from the pigmented iris was very slow [Figure 8]. As expected, those ED50 values for carbachol (1.5 - 2.6 μM) are not different in the four types of irises from atropinesterase positive or negative albino and pigmented rabbit. This observation can be explained by the quaternary nature of the molecule, which may not be accumulated by the pigmented iris [51].

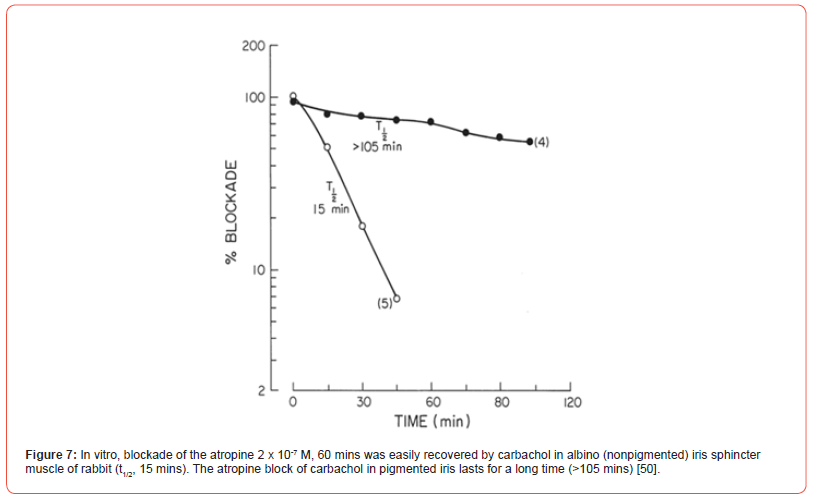
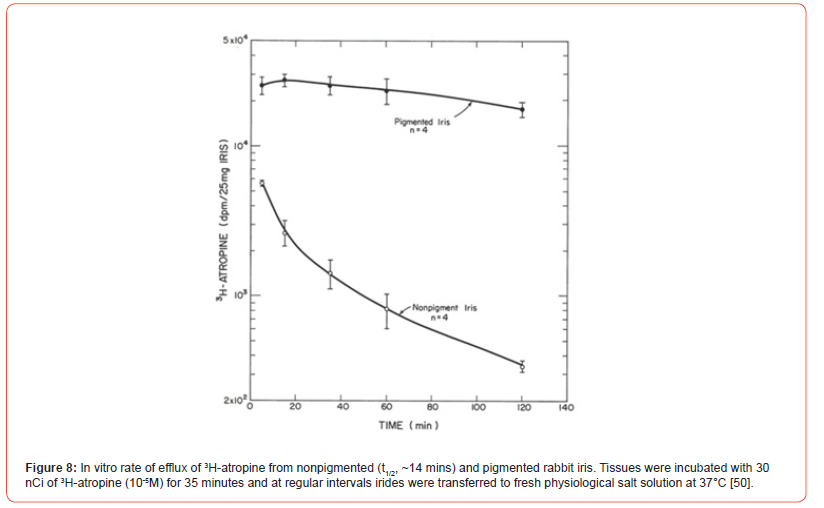
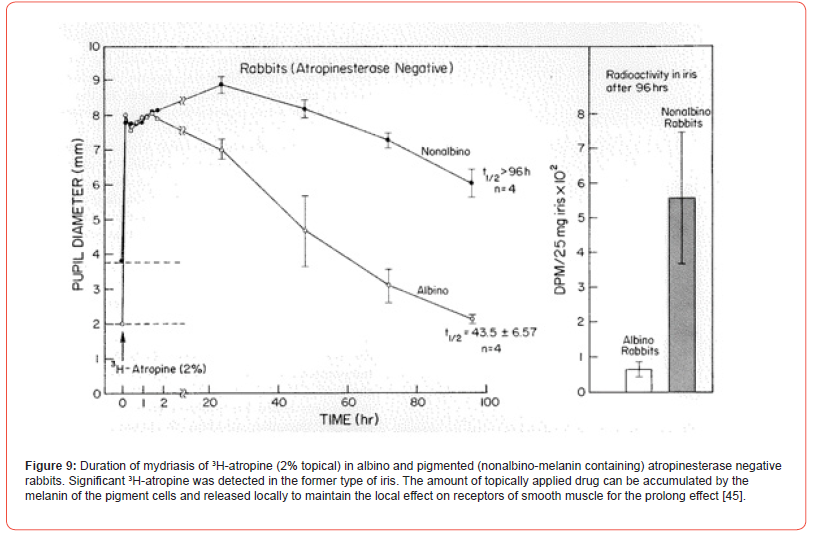
In rabbits, the drug binding to the melanin pigment as well as atropinesterase presence affects the ocular response. After topical application, the duration of atropine mydriasis in rabbits is as follows: Nonalbino atropinesterase negative > Nonalbino atropinesterase positive > Albino atropinesterase negative > Albino atropinesterase positive. Therefore, only atropinesterase negative rabbits were selected for studying the role of melanin in these nonalbino and albino animals. 3H-Atropine drops were applied to the eye. The onset and the duration of mydriasis produced by the labeled drug was recorded. The peak onset of mydriasis in albino rabbits was about 2 hours while that of the nonalbino animals was complete in 20 hours. In the latter, the duration t1/2 was >96 hours. In albino animals, the duration t1/2 was 43 hours and pupillary diameter returned to the original level in 96 hours. The 3H-atropine content of the pigmented iris of nonalbino rabbit at 96th hour was significantly greater than that from the nonpigmented iris of albino rabbits [Figure 9]. Stereoselectivity of muscarinic antagonists has been demonstrated in pigmented and nonpigmented rabbit iris. Based on Kd values, tropicamide isomers are two or three times less effective in the pigmented than in the nonpigmented iris. In vitro (-)-tropicamide blockade against carbachol of iris sphincter from albino rabbit was completely recovered in 15 minutes while that from the pigmented tissue only 45% was recovered [52].
Association of chronic use of chloroquine like drugs in retinopathy is well-known. Several investigators [53-61], and [62] have presented evidence on the nature of significant binding of phenothiazines, chloroquine and hydroxychloroquine to melanin. In different animal species, and by different methods, relationship between melanin binding of drugs to toxicity has been demonstrated. A drug bound to melanin may remain there for a long time (1 year), as reported for chloroquine [55] who studied phenothiazines binding to rabbit ocular structures and he observed an extensive binding in the pigmented tissue with little or no retention of drugs in the corresponding structures of albino rabbits. Potts concluded that phenothiazines concentrate in melanin containing structures of the eye. The affinity of phenothiazines and 4-aminoquinolines for melanin has been accepted as the main factor in the toxic retinopathy induced by these drugs.
Binding of drugs to neuromelanin present in substantia nigra of humans has been less studied. The experimental animals do not have pigment in that brain region. However, [63,64] have reported studies on the binding of agents with psychotropic activity such as imipramine, haloperidol and several phenothiazines, to pigmented human substantia nigra and the nonpigmented tissue. As compared to the latter tissue, they observed a greater accumulation of labeled drugs in the fraction corresponding to substantia nigra [Figure 10]. [65] reported an important finding like it was degeneration of pigmented nerve cells in substantia nigra of patients treated with phenothiazines for a prolonged time and which had developed tardive diskinesia. These drugs may accumulate in the pigment and produce the related toxic effect. The topic has been reviewed [66].
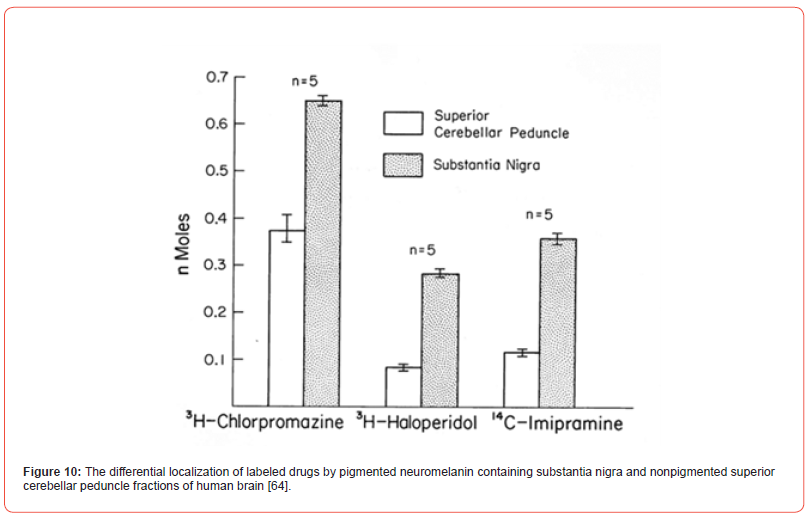
The reports of [65] and [67] findings support depigmentation of substantia nigra and cell degeneration with neurophagia in patients chronically treated with neuroleptic agents. Chlorpromazine has a special affinity for neuromelanin in extrapyramidal neurons.
Since dopamine can be a speculative precursor of neuromelanin, synthetic melanins from (-)-dopa, dopamine, (-)-α-methyl dopa and its optical isomer (+)-α-methyl dopa were prepared. All synthetic melanins exhibited electron spin resonance as observed for the natural melanin. The affinity and capacity of labeled chlorpromazine (3H CPZ) to (-)-dopa melanin was 2.67 x 108 M-1 and 1.56 x 10-8 mol/mg, respectively. The difference in the affinity of the drug binding with other synthetic melanins was small. The affinity and capacity of 3H-chlorpromazine for binding to bovine melanin granules was 3.90 x 108 M-1 and 1.89 x 10-9 mol/mg, respectively. There was a competition between the drugs for the binding. Based on similar affinity constants of chlorpromazine with various melanins, we concluded that the chemical nature of the binding indol unit of the melanins may be similar. The association of the drug binding to melanins onto toxicity to nearby tissue structure was indicated. In 1976, Hermann Blaschko, of Oxford, called “the association by content.” The positive useful drug association with melanins leading to atropine mydriasis during certain pathological conditions is also implicated.
Pure water-soluble bacterial melanin has been isolated from Bacillus thuringiensis and partially characterized by [68]. This pigment was donated by Professor Ashot Saghiyan of Yerevan, Armenia. The bacterial melanin was examined for its drug interacting characteristics by 400MHz NMR spectroscopy. In D2O buffer 1-3 mM melanin showed characteristic signals at 0.7 – 2.5 ppm attributed to aliphatic groups and dense overlapping signals at 2.5 – 4.5 ppm arising from heteroaliphatic groups. When ephedrine or atropine ≤3mM was combined with melanin, the spectral characteristics of the pigment or the aromatic or N-methyl group signals of the drugs were not altered. This finding contrasts with that reported for (-)-dopa melanin. The pigment lacks significant interaction with the drugs, perhaps due to low content of paramagnetic centers. Pharmacologic evaluation of the melanin (≤0.3 mg/ml) on the isolated frog skeletal muscle, rat aorta, vas deferens, guinea pig ileum, tracheal smooth muscle and heart, did not interfere with the activities of agonists. On the ileum, however, the bacterial melanin produced small contraction. Thus, physical and physiological properties of two types of melanins differ. Vertebrates and bacteria need two different types of melanins for different reasons. Many new functions and uses of melanins have been discovered. At Ohio State University, the observations were presented at the College Research Day on May 11, 2006. The title of the poster was “Comparative NMR studies of the drug interaction with synthetic dopa melanin and the new melanin from Bacillus thuringiensis. M. Salazar-Bookaman, J. Fowble, A. Saghiyan and P.N. Patil. Binding of chloroquine and some antibiotics to melanocytes of inner ear has been reported [69-71]. Binding of drugs to melanin and its role in ototoxicity could be important not only for these agents mentioned before but also for other agents for which ototoxicity has been demonstrated.
We would like to add importance of these studies of binding of drugs of abuse such as cocaine, amphetamines, morphine and analogs, codeine, and marihuana, among others, in forensic toxicology [44]. Due to the presence of these drugs in hair in a relatively short time – 30 minutes for cocaine, hair analysis is being used as evidence in forensic cases [72,73] and specific studies on the mechanism of incorporation of drugs in hair [74]. Even nicotine from smoke can be absorbed by hair.
Some Melanin-pigment related observations
The isolated left and right iris-sphincter of belted rabbit were set up in vitro as indicated in previous publication from our laboratory. The muscarinic agonist carbachol, 10-5 M, which produced nearly 90% of the contraction of the tissue, was used as control. Each iris was exposed to 2 x 10-7 M of atropine or methyl atropine for 1 hour. The response to carbachol was completely blocked. Tissues were similarly washed every 30 minutes and antimuscarinic effect or the response to carbachol was retested. In 30 minutes, the recovery to carbachol in both irides was ~30%. After 60 minutes, there was a gradual recovery of response to carbachol which was greater than 11% in the tissue treated with the quaternary analog than that with atropine. The observation indirectly supports that the quaternary analog is not significantly absorbed by the pigment cell as that of liposoluble atropine which was difficult to wash off the tissue. The retention of tertiary atropine in the pigment cell close to muscarinic receptor produces a prolonged blockade. (P.N. Patil, In vitro rates of recovery of antimuscarinic effects of atropine sulfate and its quaternary analog atropine methyl bromide in sphincter of pigmented rabbit irides. Expts February 11, 12 & 13, 1987, in 3 pairs of rabbit irides in vitro).
The post junctional α-adrenoceptor of albino and pigmented rabbits as well as that of pigmented human dilator muscle were examined. Since the agonist disposition in the tissue obscures the dose response curve, the EC50 of (-)-norepinephrine in the tissue was compared after blocking the drug disposition. The EC50 values of (-)-norepinephrine in the albino rabbit, pigmented rabbit, and human iris dilator did not differ and ranged from 99 to 195 nM. Under these conditions, EC50 values of phenylephrine were 710 nM in both irises of albino and pigmented rabbit. The reactivity of the dilator α-adrenoceptor may be similar. In human iris dilator, the EC50 of phenylephrine was 1220 nM. In this muscle, the partial agonist activity of oxymetazoline was 48% and 36% for p-aminoclonidine. The dissociation constant, KB, of prazosin in albino iris dilator was 8.6 nM. The competitive α-adrenoceptor blocker prazosin was 100 and ~10 fold less active in pigmented rabbit and human iris, respectively. Drug uptake by melanin may obscure with the quantitation [75]. In relation to the chronic use of the α-adrenoceptor blocker to reduce ocular pressure, a floppy iris syndrome was reported. On chronic use, the binding of the drug to ocular melanins was implicated [76].
Albino and non-albino or pigmented rabbits, three each in relatively low light had an average pupil diameter of 5 mm and 5.4 mm, respectively. Physostigmine sulfate, 0.25%, was applied topically to both eyes. The onset of pupillary constriction of the albino rabbit was in 5 minutes and a complete miosis to 2.25 mm (6 observations) was observed in 15 minutes. The onset of pupillary miosis was greater than 10 minutes and at least 25 minutes were required for ~2.75 mm of maximum miosis in pigmented iris (6 observations). Is there a difference in corneal thickness? The reason(s) for the differential onset of the effect were not explored. The phenomenon is interesting. (Margarita Salazar and P.N. Patil. Differential miotic effects of topical physostigmine sulfate, 0.25% to Albino and pigmented rabbits. Unpublished observations 1980s).
Few adult dogs with bicolor iris were tested for the ocular drug effects. A photograph of a German Shepard is presented [Figure11]. The topical physostigmine 0.2% to both eyes produced differential miotic effects in light and brown color eye [Figure 12]. During miosis, topical atropine sulfate, 1% caused differential mydriatic effect. In a separate test, morphine sulfate 3 mg/kg, subcutaneously developed an equal miosis in both eyes of the dog [Figure 13]. The drug is known to act on the morphine receptor of Edinger Westphal Nucleus in the brain to activate the oculomotor nerve, so equal effects in both eyes were expected. The poster was presented at the FASEB meeting in Washington, D.C. (FASEB J. Vol. 4, A1128, 1990).
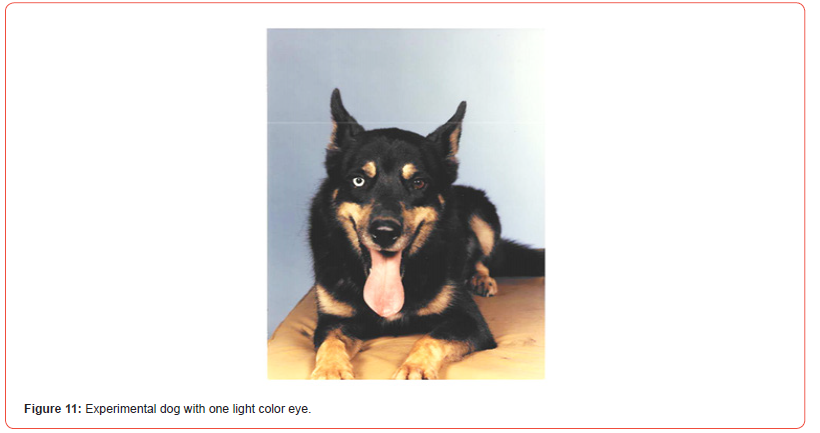
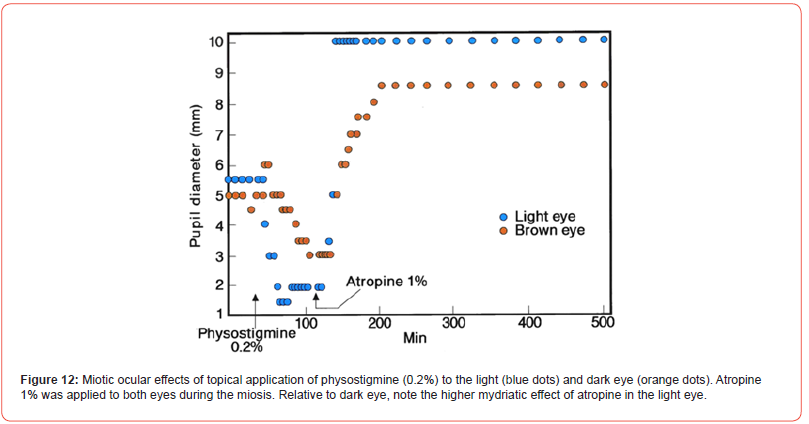
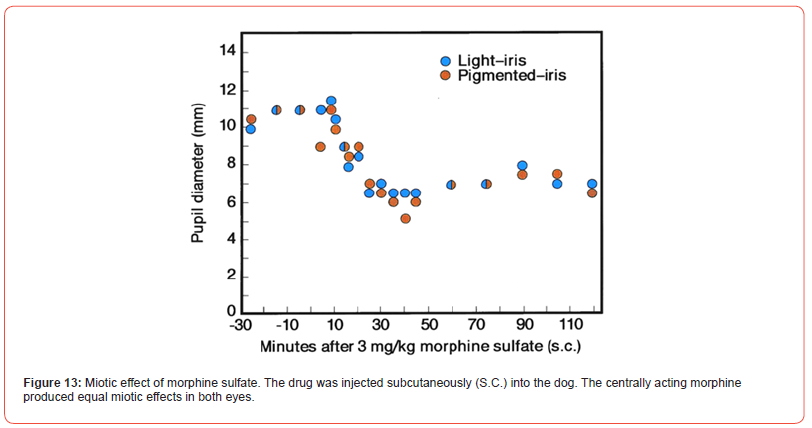
Ager Susan and Stephanie Sinclair in The Perils of Pale, National Geographic 231 #6 P. 70-91, 2017 reported several photographs and writing on albinism in children and families in India, Panama, 1:70 and Africa, 1:10,000. In the World 1:40,000 is the frequency of OCA-2-Albinism- the most common condition in which only a small amount of melanin may be produced. If exposed to too much sun, dark skin patch may result in cancer. The document contains interesting photograph and related information of the Indian family. Social and scientific implications are thought provoking.
Recently, [77] reported on the chemistry of popular hair products. As early as 1907, p-phenylenediamine was introduced for creating hair color. Many products contain an alkalizing agent and an oxidizer like hydrogen peroxide. The mixture is applied for 30-45 minutes to hair so that color of the melanin is altered. The turnover of melanins is very slow. The chemical reaction is presented [Figure 14].

Different hair colors are created by adding other couplers like 4-chlororesorcinol. Some amine products can cause contact allergic reactions and are prohibited for application to eyelashes or eyebrows. Addition of endocrine disrupting chemicals as parabens, fragrance and diethyl phthalate to the product created another problem. P-Phenylenediamine and related chemicals have been shown to cause cancer. Dopamine is oxidized to synthetic melanin called “polydopamine” where the classic indolequinone unit to hydroxyindole to dopamine are linked. The color is brown. The product applied to blond hair turns brown. In addition to the use, the safety of natural products was explored.
The scientists at Proctor & Gamble Co. in Cincinnati, Ohio headed by Tomohiro Hakozaki found old emulsifiers that were used in skincare products, abolished the skin pigment spots in humans. Excess melanocyte dendrites are blocked. Sucrose dilaurate and sucrose laurate in a fixed ratio as useful emulsifiers with niacinamide and vitamin B3 is recently approved for the purpose (Advertorial Science, March 18, 2022). This review presents a gener al view of all types of melanin in all types of organisms. Melanin is frequently considered just an animal cutaneous pigment and is treated separately from similar fungal or bacterial pigments. Similarities concerning the phenol precursors and common patterns in the formation routes are discussed. All melanins are formed in a first enzymatically controlled phase, generally a phenolase, and a second phase characterized by an uncontrolled polymerization of the oxidized intermediates. In that second phase, quinones derived from phenol oxidation play a crucial role. Concerning functions, all melanins show a common feature, a protective role, but they are not merely photoprotective pigments against UV sunlight. In pathogenic microorganisms, melanization becomes a virulence factor since melanin protects microbial cells from defense mechanisms in the infected host. In turn, some melanins are formed in tissues where sunlight radiation is not a potential threat. Then, their redox, metal chelating, or free radical scavenging properties are more important than light absorption capacity. These pigments sometimes behave as a double-edged sword, and inhibition of melanogenesis is desirable in different cells. Melanin biochemistry is an active field of research from dermatological, biomedical, cosmetical, and microbiological points of view, as well as fruit technology.
According to the literature published on melanin and our own experience, it looks like that binding of drugs to melanin has become an important topic for drug kinetics and the influence of drugs in melanin system is also becoming an important issue in therapeutics. The role of neuromelanin in the brain is to be determined.
Acknowledgement
Authors want to acknowledge Ms Jessica Carvajal for the preparation of figures for this publication. The help from the faculty colleague Liva Rakotondraibe in a preparation of a figure is appreciated.
Conflict of Interest
None..
References
- Mason HS (1953) The structure of melanins. In, Pigment Cell Growth. Gordon, M. (ed.), Academic Press, New York pp: 277-303.
- Seiji M (1967) Melanogenesis. In Ultrastructure of Normal and Abnormal Skin. Zelickson, A (ed.), Lea & Febiger, Philadelphia pp: 183-201.
- Swartz HM (1972) Cells and Tissues. In, Biological Applications of Electron Spin Resonance. Swartz, H.M., Bolton, J.R. and Borg, D.C. (eds.), Wiley-Interscience, New York pp: 155-195.
- Thomson RH (1974) The pigments of reddish hairs and feathers. Argewandte Chemie 13(5): 305-312.
- Hegedus ZL, Altschule MD (1970) Studies on rheomelanins. I. The formation of rheomelanins in human-blood plasma from catecholamines, from L-dopa and from some other derivatives. Arch. Int. Physiol. Biochem 78(3): 443-459.
- Cherubino M (1972) Light, camouflage, structure and control of the melanocytes in the inner ear. In, Research Progress in Organic, Biological and Medicinal Chemistry, Vol. III, Parte II. Gallo, U. and Santamaria, L. (eds.), American Elsevier Publishing Co., Inc pp: 754-763.
- Brini A (1973) Hypochromia of the rabbit iris induced by 6-hydroxy-dopamine. Invest. Ophthal 12(4): 312-313.
- Kim MY, Sokoloski TD, Patil PN (1984) Kinetics of the efflux of 3H-norepinephrine from rabbit irides. Naunyn-Schmiedeberg’s Arch. Pharmacol 328(2): 142-147.
- Farah MB, Patil PN (1979) Release and distribution of [3H]-norepinephrine in non-pigmented and pigmented rabbit iris. Proc. Natl. Acad. Sci 76(5): 2447-2449.
- Patil PN, Trendelenburg U (1982) The extraneuronal uptake and metabolism of 3H-isoprenaline in the rabbit iris. Naunyn-Schmiedeberg’s Arch. Pharmacol 318(3): 158-165.
- Fleig HA, Patil PN, Krieglstein GK (1987) The neuronal and extraneuronal uptake and metabolism of 3H-(-)-noradrenaline in the rabbit iris. Internac. Ophthal 10(1): 15-22.
- Lerner AB, Fitzpatrick TB (1953) The control of melanogenesis in human pigment cells. In, Pigment Cell Growth. Gordon, M. (ed.), Academic Press, New York pp: 319-333.
- Kamakshi R (2012) Fairness via formulations: A review of cosmetic skin-lightening ingredients. J. Cosmet. Sci 63(1): 43–54.
- Nicolaus RA (1962) Biogenesis of melanins. Rass. Med. , 9, Suppl. 1 (Citado por Blois, M.S., 1971).
- Axelrod J, Lerner AB (1963) O-methylation in the conversion of tyrosine to melanin. Biochem. Biophys. Acta 71: 650-655.
- Sugumaran M (1991) Molecular mechanisms for mammalian melanogenesis. Comparison with insect cuticular sclerotization. FEBS Letters 293(1-3): 233-239.
- Solano F (2014) Melanins: Skin pigments and much more. Types, structural models, biological functions, and formation routes. New J. Science V pp: 28.
- Chavin W (1971) Approaches to the study of melanocyte and melanophore. En, Biology of Normal and Abnormal Melanocytes. Kawamura, T., Fitzpatrick, T.B. and Seiji, M. (eds.). University Park Press, Baltimore pp: 77-98.
- Misuraca G, Nicolaus RA, Prota G, Chiara G (1969) A cytochemical study of phaeomelanin formation in feather papillae of New Hampshire chick embryos. Experientia 25(9): 920-922.
- Kobayashi T, Vieira WD, Potter B, Sakai C, Imokawa G, et al. (1995) Modulation of melanogenic protein expression during the switch from eu- to phaeomelanogenesis. J. Cell. Sci 108(Pt 6): 2301-2309.
- Binns F, King JAG, Mishra SN, Percival A, Robson NC, et al. (1970) Studies related to the chemistry of melanin. XIII. Studies on the structure of dopamine melanin. J. Chem. Soc 15: 2063-2070.
- Thathachari YT (1973) Structure of melanins. In, The Pigment Cell, Vol. 1, Mechanisms of Pigmentation. Riley, V. (ed.), Karger, Basilea pp: 158-164.
- Adams M, Blois MS, Sands RH (1958) Paramagnetic resonance spectra of some semiquinone free radicals. J. Chem. Phys 28: 774-776.
- Shimada K, Baweja R, Sokoloski T, Patil PN (1976) Binding characteristics of drugs to synthetic levodopa melanin. J. Pharm. Sci 65(7): 1057-1060.
- Mason HS, Ingram DJE, Allen B (1960) The free radical property of melanins. Arch. Biochem. Biophys 86: 225-230.
- Bolt AG (1967) Interactions between human melanoprotein and chlorpromazine derivatives. I. Isolation and purification of human melanoprotein from hair and melanoma tissue. Life Sci 6(12): 1277-1283.
- Van Woert MH, Prasad KN, Borg DC (1967) Spectroscopic studies of substantia nigra pigment in human subjects. J. Neurochem 14(7): 707-716.
- Ruffolo RR, Patil PN (1974) Catecholamine content of pigmented and nonpigmented tissues of rabbit. Europ. J. Pharmacol 25(2): 255-258.
- Werner G (1965) Fermentdefekte als ursache unterschiedlicher mydriatischer wirksamkeit von atropine and cocaine bei kaninchen. Naunym-Schmiedeberg’s Arch. Pharmacol. Expt. Pathol 251: 320-334.
- Van Zutphen LFM (1972) Qualitative and quantitative detection of atropinesterase and cocainesterase in two breeds of rabbits. Enzymologia 42: 201-217.
- Ullberg S (1958) Autoradiographic studies on the distribution of labeled drug in the body. In, Proceedings of the Second United Nations Conference on the Peaceful Uses of Atomic Energy, Vol. 24, Geneva pp: 248-254.
- Lindquist NG (1973) Accumulation of drugs on melanin. Acta Radiol 325: 1-92.
- Dencker L, Lindquist NG, Ullberg S (1973) Mechanism of drug induced otic lesions. Role of drug accumulation on the melanin of the inner ear. Experientia 29(11): 1362-1363.
- Dencker L, Lindquist NG, Ullberg (1975) Distribution of an 125I-labelled chloroquine analogue in a pregnant Macaca monkey. Toxicology 5(2): 255-264.
- Chen KK, Poth EJ (1929) Racial differences as illustrated by mydriatic action of cocaine, euphthalmine and ephedrine. J. Pharmacol. Exp. Ther 36(3): 429-445.
- Barbee RF, Smith WO (1957) A comparative study of mydriatic and cycloplegic agents in human subjects without eye disease. Amer. J. Ophthal 44(5 Pt 1): 617-622.
- Angenent WJ, Koelle GB (1953) A possible enzymatic basis for the differential action of mydriatics on light and dark irides. J. Physiol. (London) 119(1): 102-117.
- Obianwu MO, Rand MJ (1965) The relationship between the mydriatic action of ephedrine and the colour of the iris. Brit. J. Ophthal 49(5): 264-270.
- Seidehamel RJ, Tye A, Patil PN (1970) An analysis of ephedrine in relationship to iris pigmentation in the guinea pig eye in vitro. J. Pharmacol. Exp. Ther 171(2): 205-13.
- Patil PN (1972) Cocaine-binding by the pigmented and non-pigmented iris and its relevance to mydriatic effect. Invest. Ophthal 11(9): 739-746.
- Patil PN, Jacobowitz D (1974) Unequal accumulation of adrenergic drugs by pigmented and nonpigmented iris. Amer. J. Ophthalmol 78(3): 470-477.
- Salazar-Bookaman MM, Fowble J, Weber P, Patil PN (1989) Investigation by NMR spectroscopy of the interaction between synthetic soluble (-)-dopa melanin and drugs. Naunyn-Schmiedeberg’s Arch. Pharmacol 340(5): 576-82.
- Salazar-Bookaman MM, Fowble JW, Patil PN (1992) Stoichiometry of interaction between soluble (-)-dopa melanin and enantiomers of ephedrine by NMR spectroscopy. Chirality 4(7): 423-426.
- Borges CR, Roberts JC, Wilkin DG, Rollins DR (2003) Cocaine, benzoylecgonine, amphetamine, and N-acetylamphetamine binding to melanin subtypes. J. Anal. Toxicol 27(3): 125-134.
- Salazar M, Patil PN (1976) An explanation for the long duration of mydriatic effect of atropine in eye. Invest. Ophthal 15(8): 671-673.
- Fieger H, Iredale J, Wainer IW (1993) Enantioselective determination of hydroxychloroquine and its major metabolites in urine and the reversal in the (+)/ (-)-hydroxychloroquine ratio. Chirality 5(2): 65-70.
- Salminen L, Urtti A (1984) Disposition of ophthalmic timolol in treated and untreated rabbit eyes. A multiple and single doses study. Exp. Eye Res 38(2): 203-206.
- Katz IM, Berger ET (1979) Effects of iris pigmentation on response of ocular pressure to timolol. Survey. Ophthal 23(6): 395-398.
- Chiou GCY (1990) Development of D-timolol for the treatment of glaucoma and ocular hypertension. J. Ocular Pharmacol 6(1): 67-74.
- Salazar M, Shimada K, Patil PN (1976) Iris pigmentation and atropine mydriasis. J. Pharmacol. Exp. Ther 197(1): 79-88.
- Akesson C, Swanson C, Patil PN (1983) Muscarinic receptor of rabbit irides. Naunyn-Schmiedeberg’s Arch. Pharmacol 322(2): 104-110.
- Patil PN (1978) Antimuscarinic effects of stereoisomers of tropicamide on rabbit iris sphincter. Invest. Ophthal. Vis. Sci 17(1): 65-68.
- Goar EL, Fletcher MC (1957) Toxic corioretinopathy following use of NP-207. Am. J. Ophthal 44(5 Pt 1): 603-608.
- Scott AW (1963) Retinal pigmentation in a patient receiving thioridazine. Arch. Ophthal 70: 775-778.
- Potts AM (1964) The reaction of uveal pigment in vitro with polycyclic compounds. Invest. Ophthal 3: 405-416.
- Blois MS (1965) On chlorpromazine binding in vivo. J. Invest. Dermatol 45(6): 475-481.
- Blois MS (1968) Melanin binding properties of iodoquine. J. Invest. Dermatol 50: 250-253.
- Sidall JR (1966) Ocular toxic changes associated with chlorpromazine and thioridazine. Canad. J. Ophthal 1(3):190-198.
- Lindquist NG, Ullberg S (1972) The melanin affinity of chloroquine and chlorpromazine studied by the whole-body autoradiography. Acta Pharmacol. Toxicol 2: Suppl 2: 1-32.
- Cameron ME, Lawrence JM, Olrich JG (1972) Thioridazine (Mellaril) retinopathy. Brit. J. Ophthal 56(2): 131-134.
- Legros J, Rosner I, Berger C (1973) Retinal toxicity of chlorpromazine in the rat. Toxicol. Appl. Pharmacol 26(3): 459-465.
- Jagadeesh JM, Lee HC, Salazar-Bookaman M (1980) Influence of chlorpromazine in the rabbit electroretinogram. Invest. Ophthal. Vis. Sci 19(12):1449-1456.
- Salazar M, Rahwan R, Patil PN (1976) Binding of 14C-imipramine by pigmented and non-pigmented tissues. Eur. J. Pharmacol 38(2): 233-241.
- Salazar M, Sokoloski TD, Patil PN (1978) Binding of dopaminergic drugs by the neuromelanin of the substantia nigra, synthetic melanin and melanin granules. Fed. Proc 37(10): 2403-2407.
- Christensen E, Moller JE, Faurbye A (1970) Neuropathological investigation of 28 brains from patients with diskynesia. Acta Physiol. Scand 46(1): 14-23.
- Dayhaw-Barker P (2002) Retinal pigment epithelium melanin and ocular toxicity. Int. J. Toxicol 21(6): 451-454.
- Forrest FM (1974) Evolutionary origin of extrapyramidal disorders in drug treated mental patients, its significance and the role of neuromelanin. In, Advances in Biochemical Pharmacology, Vol. 9, Phenothiazines, Forrest, I.S., Carr, C.J. and Usdin, E. (eds.). Raven Press, New York pp: 255-268.
- Aghajanyan AE, Hambardzuman AA, Hovsepyan AS, Asaturian RA, Vardoyan AA, et al. (2005) Isolation, purification, and physiochemical characterization of water-soluble Bacillus thuringiensis melanin pigment cell. Res 18(2): 130-135.
- Stupp H, Kupper K, Lagler F, Sous H, Quanta M, et al. (1973) Inner ear concentrations and ototoxicity of different antibiotics in local and systemic application. Audiology 12(5): 350-363.
- Harpur ES, D´Arcy PFD (1975) The influence of pigmentation of rats and guinea pigs on the ototoxicity of kanamycin and neomycin. Experientia 31(11): 1323-1324.
- Tran Ba Huy P, Meulemans A, Wassef M, Manuel C, Sterkers O, et al. (1983) Gentamycin persistence in rat endolymph and perilymph after a two-day constant infusion. Antimicrob. Agents Chemother 23(2): 344-346.
- Nakahara Y, Takahashi K, Kikura R (1995) Hair analysis for drug abuse. X. Effect of physicochemical properties of drugs on the incorporation rates into hair. Biol. Pharm. Bull 18(9): 1223-1227.
- Joseph RE, Tsung-Ping S, Cone EJ (1996) In vitro binding studies of drugs to hair: Influence of melanin and lipids on cocaine binding to Caucasoid and Africoid hair. Anal. Toxicol 20(6): 338-344.
- Cone EJ (1996) Mechanisms of drug incorporation into hair. Ther. Drug Monitor 18(4): 438-443.
- Ishikawa H, Miller DD, PN Patil (1996) Comparison of post-junctional α-adrenoceptors in iris dilator muscle of humans and albino and pigmented rabbits. Naumyn-Schmiedeberg’s Arch. Pharmacol 354(6): 765-772.
- Goseki T, K Shimizu, H Nishimoto, S Uga, N Nemoto, et al. (2008) Possible mechanism of intraoperative floppy iris syndrome: a clinicopathological study. Brit.J. Ophthalmol 92(8): 1156-1157.
- Bomgardner MM (2020) Hair color chemistry gets a fresh look. C & en 98(17): 29-33.
- Walls GL (1967) The vertebrate eye and its adaptive radiation, Hafner Publishing Co. New York p: 15.
-
Maria Margarita Salazar Bookaman* and Popat N Patil. Pharmacologic And Related Implications of a Drug Accumulation by Melanins. Arch Phar & Pharmacol Res. 3(4): 2023. APPR.MS.ID.000568.
-
Microscopic morphology, Terminology, Drugs, Retinopathy, (-)-Ephedrine, Tyrosinase, Polymerization, Electron Microscopy (EM)
-
This work is licensed under a Creative Commons Attribution-NonCommercial 4.0 International License.