Research Article
Sediment Characteristics and Estimation of Toxicity Site Specific Criteria for Sediments of Iranian Coasts, The Persian Gulf
Vahid Aghadadashi1*, Saeideh Molaei2, Ali Mehdinia1 and Hamid Rezai Marnani3
1Department of Marine Living Science, Marine Sciences Research Center, Iranian National Institute for Oceanography and Atmospheric Science, Iran
2Department of Chemistry, Kharazmi University, Iran
3Department of Marine Living Science, Darya Negar Pars Consulting Engineers, Iran
Vahid Aghadadashi, Department of Marine Living Science, Marine Sciences Research Center, Iranian National Institute for Oceanography and Atmospheric Science, Iran.
Received Date: February 16, 2022; Published Date: March 28, 2022
Abstract
Surface sediments from Iranian coasts of the Persian Gulf (n=121) were studied to track the spatial distribution of organic matter, carbonate level and grain size. The theoretical organic carbon (TOC) levels were among the high values ever reported, which suggested that the sediments possibly are dealing with impaired benthic fauna. A significant correlation was observed among water depth, TOM and carbonate levels as well as grainsize of the studied sediments (TOM(%)= 0.111water depth(m) + 0.025 (Sil+Clay) (%) -0.286Carbonate (%) + 15.441, adjusted R2= 0.898, p< 0.01). The studied region covered nearly 1% of the Persian Gulf’s surface area and contained heterogeneous spatial distribution for sedimentological factors. The examination of interpolated maps introduced the Pars Special Economic Energy Zone (PSEEZ) as a region with possibly high burdens of organic carbon and its co-occurring eco-pollutants. Benzo(a)pyrene and naphthalene site specific criteria were mapped. According to the maps, the intermediate zone and the Nayband Bay were much vulnerable against entering pollutants. It could be due to low levels of organic matter in the sediments of these regions. The sediment quality guidelines suggested that the biota of the ecologically and economically hot zone of the Iranian coast (EHZIC)is dealing with Bnzo(a)Pyrene and Naphthalene related adverse hazards, rarely and rarely-occasionally. Indicator kriging analysis and probability map proposed that Naphthalene related chronic toxicities are probable in the EHZIC. The highest probabilities were spatially distributed in the PSEEZ and the Nayband Bay. The ratios of Bezo (a)Pyrene to its site-specific criterion were significantly low and its release the toxic effects of B(a)P was not probable for the biota inhabited.
Keywords: Grainsize; Organic matter; Pars special economic energy zone; Persian gulf; Probability map; Sediment; Monitoring zones; Ecological and economical significance
Introduction
The Persian Gulf as a branch of the Indian Ocean is ecologically vulnerable ecosystem and has approximately two-thirds of the world’s proven oil reservoirs [1-3]. The extensive oil and gas related activities have turned the Persian Gulf to a permanent oil receiving area [4]. Moreover, the Persian Gulf is a well-known instance of the regions heavily subjecting to the shifting baseline syndrome where the accumulation of many small industrial projects leads to major changes [2]. On the other hand, the productivity of the Persian Gulf is essentially sediment-based [5], which can be disturbed by such human mediated alterations. The enrichment of organic matters in sediments can create environmental anomalies. For example, [5] have expressed that oil pollution in the Persian Gulf promotes TOC levels to 0.5-1.5% while its natural background level is 0.5%. Data by [6] showed an increase in TOC to 2.8%, which results in increased primary production that may affect the corals and other reef-building organisms. Thus, the sediment quality in the case of organic enrichment in the Persian Gulf requires special and urgent attention. On the other hand, the grain size of sediments has severe effects on oxidation-reduction status of the sedimentary substrates. As a rule of thumb, finer sediments are associated with lower redox potentials and higher sulfur levels [11]. For instance, sulfur contents up to 1756u
mole and white carpet of Begiatoa sp were detected in the Persian Gulf where the summertime stratification decreased the oxygen supply of previously enriched sediments (Unpublished data). The summertime stratification, however, is dependent to water depth as well. Accordingly, TOC level, grain size and water depth are determinant factors affecting very aspects of sediments and inhabited biota. Moreover, the carbon bearing particles transfer the organic chemicals into sediments [7,8]. The inhabited biota in these enriched sediments, therefore, encounters with multiple co-varying stressors [9,10]. This kind of deposition archives the chemicals for long periods of time and generates potentially long-term eco-hazards. Benzo(a)pyrene for example, can inhibit the role of the bioturbator Tubifex tubifex in sediment biogeochemistry [11].
The present study is conducted to address the levels and spatial deposition of sedimentological factors in an ecologically and economically hot zone of the Iranian coast (EHZIC) in the Persian Gulf. The results also manipulate to shed light to Nap and B(a)P behaviors in surface sediments of the study region where complicated sources and sinks are available. To do that, Na and B(a)P site specific criteria is calculated and visualized by GIS-based mappings. The criteria can indicate the vulnerability of the area against these eco-pollutants. Then, the results of Na and B(a)P in combination with site specific criteria can be employed to create probability maps if possible. The probability maps delineate useful two-dimensional spaces in which the probability of exceeding or not exceeding of a threshold value is investigated. This helps the scientist to find the spots with high probability of eco-hazards. Hence, the other subject of this article is addressing vulnerability of the examined zones and their probable vulnerability by Na and B(a)P site specific criteria and probability map(s). Monitoring zones; Ecological and economical significance (Figure 1) shows the regional classification of the studied area. The study zone stretches from the Dayyer-Nakhilou National Park to the South-Eastern of the Persian Gulf. The Park is an ecologically hot zone and contains mangrove forest (Mel- Gonzeh) and three miniature islands. The Mel-Gonzeh Forest is the northernmost limit of mangrove distribution in the Persian Gulf and hosts the Avicennia marina vegetation type. Omolgorm, Tahmadoon and Nakhilou are the islands which support the greatest breeding colony of the crab plover (Dromas ardeola) in the Middle East. Sea bed swelling in the Motaf region is something of interest where the Eastern-Western direction of the Persian Gulf suddenly changes to a Southern-Northern direction. The Motaf region also experience extreme current speeds, up to 1.55 m S -1 at maximum level (Figure 2). Some areas of the study region are spawning and nursery grounds for commercially valuable species such as tiger shrimp (Penaeus semisulcatus). These breeding and nursery areas are mainly located between the Dayyer-Nakhilou national Park and the Dayyer fishery port [15]. Corals in the study region are sparsely distributed between the Dayyer port and the Nayband Bay (Figure 1). As a whole, coral communities of the study region are experiencing low threat of the marine pollution [34], the situation is more severe for corals of the Nayband Bay and its adjacent area, the Pars Special Economic Energy Zone (PSEEZ). The PSEEZ covers 100 square kilometers of land at Asaluyeh city and its South-Eastern part leads to entrance of the Nayband Bay where the Iranian first national marine Park has been established in 2004 (Figure 1). The Nayband national marine Park involves coral communities and mangrove forests. It supports a unique coral area in the mainland coastal waters of the Iranian side of the Persian Gulf. In conclusion, the study region contains both industrial centers and key natural habitats and covers nearly 1% of the Persian Gulf’s surface area (Figure 1).
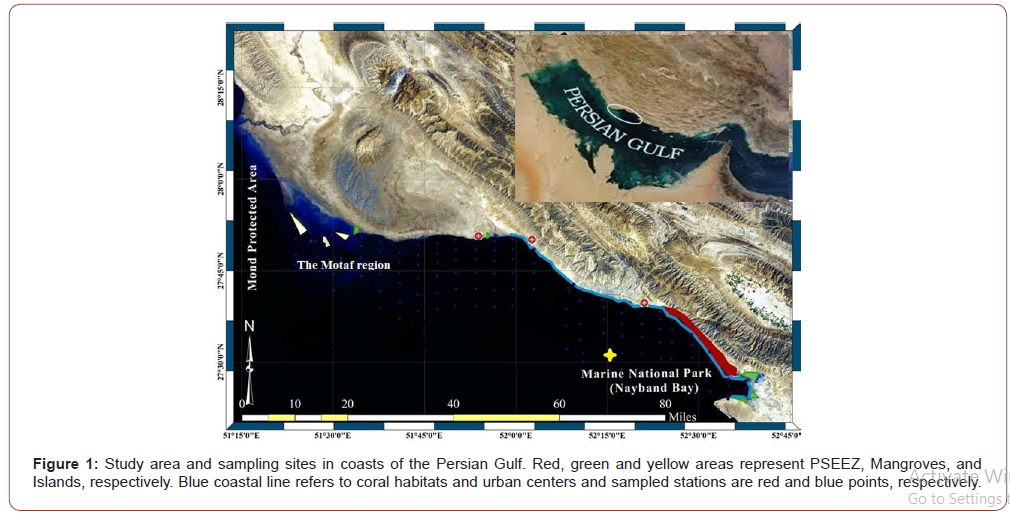
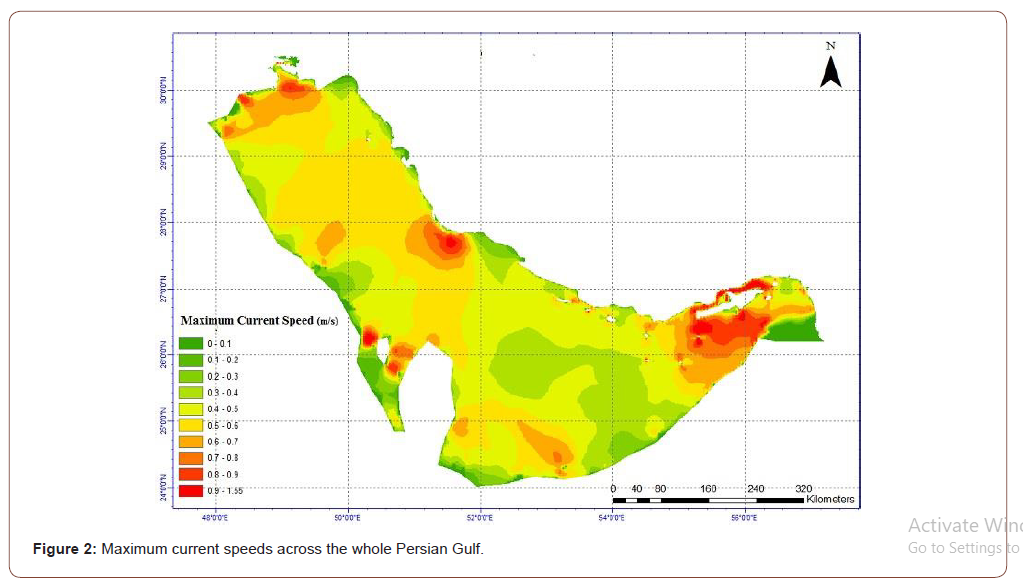
Materials and Methods
Sampling design and pretreatments
The sampling region is between Northern latitude 27°54’57.76” to 27°24’50.52”N and Eastern longitude 51°23’49.97” to 52°40’13.02”E which nearly covers 2800 square kilometers. The region was divided to 24 transects separated by nearly 2.7 nautical miles distances. The first sampling stations of all transects were selected as nearshore stations (~ 0.27-0.8 nautical miles off the shoreline) and the successive stations were sampled at distances equal to 1.62, 3.24, 5.4, 8.1, and 10.8 nautical miles off the shore in average (Figure 1). In summary, the study matrix was formed by sampling from 6 horizontal legs in about 24 vertical transects. However, the plan was not functional for both national Parks and the Parks were sampled sparsely. By doing so, the surface sediments (0-5 cm) from 121 stations were gathered using Van Veen grab and transferred in pre-washed and clean aluminum containers to the laboratory. Besides, vertical changes in some water parameters (salinity, temperature, oxygen level, and density) were recorded using an Ocean Seven 305 plus CTD (IDRONAUT, Italy). Totally, 1564 vertical records for each examined water parameters were registered by over 43 CTD casts. Salinity, temperature, and density profiles of water column subsequently was prepared and used in our analysis. Moreover, the data and maps of wave return periods (25 years) and maximum current speeds of the Persian Gulf were prepared from Darya Negar Pars Consulting Engineers (Tehran, Iran). The water depth at sampling sites was measured onboard by a portable depth sounder (Speedtech, USA). Geographical information of all sampling stations was determined by a global positioning system (Garmin, ETREX 10).
In laboratory, the sediments were kept frozen at -20 ºC. Then, the frigid sediments were put into a freeze drier (Operon set, 5503-Korean) to lyophilize at -54 ºC and 70 mTorr pressure (72h). The vacuum dried sediments then stored at -20ºC in dark until analysis.
Sedimentology experiments
Sedimentology experiments were performed for organic matter and grainsize assessments. Total Organic Matter (TOM) and carbonate levels were evaluated using Sequential Loss On Ignition method (SLOI) [12,13]. For TOM analysis, 1.5-2 g of dried sediments (< 250 μm, 105º C, 7 h) was weighted after placing them in a furnace (NABERTHERM, P330, Germany) at 550◦C for 4 h. The resultant baked sediments were reheated to 950 ºC (2 h) and the relative weight losses were transformed to carbonate levels of the sediments by molar ratios [12,13].
Granulometric analyses were performed using 2 g of homogenized and dried sediments soaked by 20 mL of 3% tetra sodium diphosphate decahydrate. Each of the soaked sediments were stirred by means of magnet bars for 12 h in order to disintegrate the colloidal particles. The slurries were put into a laser scattering particle size distribution analyzer HORIBA, LA-950 (Horiba Europe GmbH, Germany) and the size distributions of examined sediments were determined [3].
In the case of TOM and grainsize analysis, appropriate replications were run to ensure the accuracy of observed data.
Naphthalene and B(a)P Analysis
Naphthalene and B(a)P levels were determined according to method mentioned by [3]. Briefly, all sediment samples (4g) were spiked with 100 ng/g surrogate standards of naphthalene-d8, and Perylene-d12 and extracted by a microwave sample extraction system (Ethos 1, Operon, USA). Activated copper wires were added to remove the sulfur content of the samples. Each extract was cleaned up by silica/alumina column chromatography recommended for marine sediments [4]. Prior to instrumental analysis, 100 μL of p-terphenyl-d14 (100 μg L-) was added as injection standard. GC-MSD analysis was performed using a model 6890N GC system equipped with a 5973-mass detector and a HP-5 fused silica capillary column (30 m × 0.25 mm × 0.25 μm). The mass spectrometer was operated in the selective ion monitoring (SIM) mode and M/ Z= 128 and M/Z= 256 was extracted as Nap and B(a)P, respectively. The concentration of PAHs and quality assurance were assessed according to [3].
Statistics and data presentation
Statistical parameters, distribution, Coefficient of Variations (CV(%)), and correlation/regression analysis of the dataset were assessed using SPSS 19.0. Since the dataset was not normally distributed, non-parametric analysis was performed and statistical significance set at p<0.05. Geographical mappings were also performed to depict spatial deposition of sedimentological factors in the region. The results were visualized using the software package of ESRI®ArcMAPTM 10.3 and a deterministic interpolator i.e, the Inverse Distance Squared Weighting. In order to assess protection status of benthic life from Naphthalene’s chronic toxicity, probability map of Naphthalene’s chronic toxicity was prepared using Indicator Kriging.
Results and Discussion
Sedimentology and the basin conditions
Among the factors studied, water depth was a matter of interest due to its high level of variability (CV(%)= 81%) which confirms a topographically heterogeneous seabed in the EHZIC. The range of water depth varies here between 0.7m to 75m which covers subtidal to offshore areas and reaches to mean and median levels of 28.7 m and 20m, respectively (Table 1). In opposite to a general pattern of landward decreasing in water depth, the water depth increased in a West to East trend and decreased toward the Nayband national Park (Figure 3). Although the Persian Gulf is a shallow water basin, the Iranian side of the basin is significantly deeper than its Arabian side [3,6,23]. For instance, having the water depth equal to 75m in about 10.8 nautical miles off the shore at station 101 (Figure 1), the yellow asteroid) which corresponds to a percent slop of 0.35% (tg θ= 0.0035, θ= 0.21º) is routinely observed in the Iranian side of the Persian Gulf. This kind of water depth variation emphasizes that the topography of the study area is heterogeneous and the EHZIC has comparatively a shelvy structure.
It is worth noting that the general pattern of water flow in the Persian Gulf is to the Iranian shores. The collision of water currents with these shelvy and deep coasts thus underpins the settlement of relatively fine particles (sandy mud and mud) in the region of research [14,15,16]. The Spearman’s correlation analysis reveals a significant positive association between the water depth and the fine fraction distribution in the studied areas (r= 0.585, p<0.01). On the other hand, the buried particles experience higher pressure levels in the PSEEZ, too. As the equation 1 shows, the pressure levels on sediments are dependent to water density (p) and in particular, water depth values (h(m)) where P0 is local atmospheric pressure:
P= P0 + pgh [Equation 1, g= 10m s-2]According to our calculations, the pressure levels on PSEEZ’s sediments for example are about 5.72 atm which is 3.17 and 2.91 times more than imposed pressures to the sediments of the Nayband Bay and the NWL, respectively. The higher pressure can compress the sediments more effectively. Thus, it can limit the rate of solvent exchange for eco-pollutants in the deeper areas. Additionally, the higher depth of the PSEEZ can form thermal stratification during summertime which affects the very aspects of the region’s sediments. This can possibly keep the buried carbon and its co-varying stressors away from chemical oxidation. This in turn archives the chemicals for long periods of time and generates potentially long-term eco-hazards in most parts of the EHZIC.
In highly energetic environments, the sediments tend to be coarse grain. In low energy environments however, a significant deposition of fine sediments is common which affects the sediments and biota inhabited. According to the results, silt and clay size sediments (above 4Ø or <63 μm) in the EHZIC cover 0.75% to 100% of the stations examined and make the sedimentary bed inevitably fine in average (62.5%). Comparison of median (70.13%) and mean levels as well as examination of related skewness graph propose that there is an obvious bias to the settlement of finer sediments in the EHZIC. The fine fraction, however, shows a variability of 60% in the studied sediments (Table 1). Here, (Figure 3 ) demonstrates that the sediments from the Nay band Bay (a semi-enclosed and shallow Bay) and the Western limits of the EHZIC (NWL; 51º4500E to 51º2300E) are composed of coarser sediments meanwhile the intermediate zone and the PSEEZ tell another story. As we mentioned, the Motaf region is a part of NWL, and its hydrography is governed by the sediment discharge from the Mand River. The Mand River discharges its inputs into the Persian Gulf at 14 nautical miles farther than the NWL. On the other hand, most of sands from the Mand River is carried towards the South by longshore currents and subsequently deposited in the Motaf region. The higher current speeds in the Motaf region can also prevent the deposition of fine particles in there (Figure 3).
Table 1:Descriptive statistics for sediment properties in studied zones.

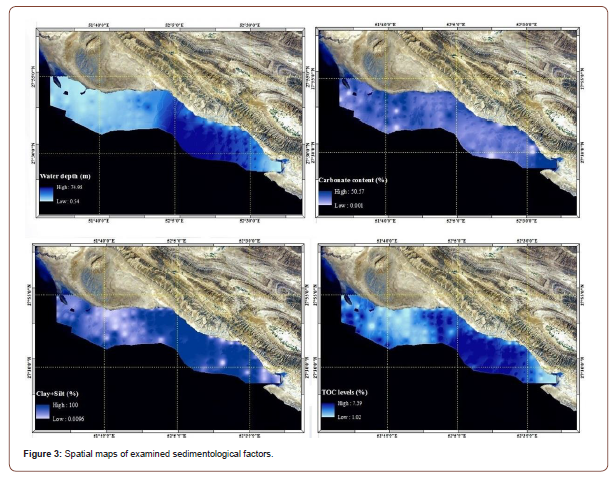
The organic matter levels in the EHZIC varies between 3.06% to 21.84% with a mean, median and CV(%) values of 12.93%, 12.87% and 51.66%, respectively (Table 1). TOM levels significantly increase when the water depth increases (r= 0.829, p<0.01). There is a significant positive association between TOM levels and fine fraction as well (r= 0.649, p<0.01). The detected TOM content thus probably follows the water depth and the grainsize factors which are both possibly a function of hydrographic gradient and current speed in the region of interest.
Carbonate content (%) of the examined zones is in the range of 10.41%-50.6% which reaches to a mean, median and CV(%) values of 24.94%, 22.40%, and 44.11%, respectively (Table 1). It is interesting to remember that the most portion of the Persian Gulf is a classic example of a mixed carbonate-siliciclastic ramp system for an arid climate[3]. (Figure 2) makes it obvious that the carbonate in sediments from EHZIC has a relatively homogeneous distribution (the lowest CV(%) levels in comparison) and its distribution pattern is in contrast with distribution trends of TOM, the water depth and the fine fraction. Spearman’s correlation analysis also shows that carbonate level in the EHZIZ is a negative correlate of water depth (r= -0.648, p<0.01), TOM (r= -0.899, p<0.01) and fine fraction (r= -0.574, p<0.01), confirming the spatial distributions observed Figure 3. It should be noted that the great values of carbonate content, the dominance of coarser sediments, and the lower water depth in the Nayband Bay (Figure 3) are comparable with Saudi Arabian-UAE coasts, where carbonate sands vastly covers the shallow waters [15]. The Nayband Bay therefore is an area with high levels of sandy carbonate particles containing lower organic matters.
Multiple regression analysis was performed to examine the existence of correlation relationships among the mentioned variables. The multiple regression analysis established a very significant regression equation (TOM(%)= 0.111water depth(m) + 0.025(Sil+Clay)(%) - 0.286Carbonate(%) + 15.441, Adjusted R2= 0.898, p< 0.01). Regarding to the R2 level, the equation can estimate the TOM(%) levels up to nearly 90% in the EHZIC. For instance, the TOM level of station 118 was 18.7% and the equation estimates its level by only 4.7% positive error i.e., 19.59%.
The TOM levels are a semi-quantitative proxy for organic carbon as well. Some authors reported that the total organic carbon contents (TOC) is approximately half of the calculated TOM levels [14]. Their proposal is mainly based on agrology data and soil organic matter. Besides, the regression analysis proposes a significant linear correlation between TOM% and TOC% for the sediments from the strait of Hurmuz (TOM%=1.614 ×TOC%+3.7604 , R2 = 0.81). However, in a comparison of methods for determination of organic carbon in marine sediments[12], suggested a factor of 0.33 for converting the TOM levels to its corresponding TOC levels. According to the late estimation, the mean level of TOC in sampling stations possibly reaches up to 4.36% per g dry wet (1.02%-7.6%) with a CV% value of 44%. As (Figure 3) emphasizes, the interpolated TOC levels signalize a heterogeneous TOC distribution in the region. Sediments from the Nayband Bay and the NWL accumulated lower levels of TOC, meanwhile the intermediate zone and the PSEEZ showed the higher corresponding values. This result is in a good agreement with the distribution algorithms observed in the case of water depth (Figure 3). The TOC level in the EHZIC is indeed a positive correlate of the water depth, and fine fraction.[17]Reported the TOC distribution in the Persian Gulf. They found that the TOC levels in sediments from the Persian Gulf (1.5%-2.8%, mean = 1.32%) exceed the natural background level (0.5%) of similar seas. [17] published similar results as well (0.45%−2.4%, mean = 1.04%) [18], also reported low levels of TOC which frequently ranged from 0.05% to 1% in studied coasts of 7 countries except the sediments from Dukhan, Qatar (2.34%) and BAPCO refinery area (4.78%), Bahrain. The sampling size of three mentioned studies (23, 47, and 55, respectively) however is not adequate enough to delineate a high-resolution picture for TOC distribution over the whole Persian Gulf. For example, [18] only examined three samples from the Iranian coast which result in a mean value equal to 0.4% for the Eastern part of the Persian Gulf. TOC levels also ranged from 0.5% to 6.5% (n= 33, mean = 2%) in sediments from the strait of [19] and between 0.4%-2.1% (n= 27, mean = 1.17%) in sediments from the Eastern Persian Gulf [20]. In comparison, our results hypothesize that the TOC values are especially high for the sedimentary bed of the EHZIC, except for the coarser sediments deposited mainly in the Nayband Bay and the NWL (Figure 3). More interestingly, these levels of TOC are among the greatest values has been reported so far for the Persian Gulf basin. Recently, Arfaeinia et al. (2017) have gathered sediment samples (n=21) from the Dayyer port to the Asaluyeh city and reported TOC levels from 8.9% to 11% (mean= 10.1%) in the region which testifies higher load/preservation of TOC in the region.
Supposing an ultimate atmospheric origin for the organic carbon (in a very simple form; CO2+H2 O+photosystems+sunlight (CH2 O) _(n ))+O2) [24,25] the mean TOC levels of the EHZIC suggest a transfer of averagely 3.63 millimole the green house CO2 g-1 dry weight of the examined sediments through the organic matters into the sedimentary records. By ignoring the turnover of carbon through detritus food chains as well as the degradation/mineralization of organic materials, some of this volume can be theoretically removed from the active parts of the carbon’s biogeochemical cycle. In comparison, the observed CO2 transfer through the organic matters of the EHZIC is about 2.02 times of the amount reported for the Chabahar Bay, the Sea of Oman [24].
On the other hand, the preservation or delayed decomposition of organic matters by sediments highlights some dramatic changes in the structure of biota involved [25]. The organic carbon values more than ~ 3% in Mid-Atlantic Integrated Assessment (MAIA) Estuaries [26] introduced those sedimentary beds as highly impacted regions containing impaired benthic communities. Hyland et al. (2005) suggested that risks of reduced species richness from organic loading and other associated stressors in sediments should be relatively high at concentrations greater than about 35 mg g-1. Oil pollution in the Persian Gulf accounts for 0.5-1.5% of TOC compared to the 0.5% of the natural background level. Data by [27] showed that TOC increase (>2.8%) shifts the diatom prevailed communities to flagellates/ dinoflagellates dominated communities and affects the benthic algae. Besides increasing primary production, benthic algae may out-compete corals and other reef-building organisms. Regarding to very high TOC levels in our study, the EHZIC is possibly experiencing impaired benthic fauna and sever selective pressures. Importantly, higher level of organic carbon not only results in nuisance by-products (Ammonia, Methane, and Sulfides) but also often co-occurs with elevated levels of other contaminants, eg, PAHs.
The results from CTD indicate that the mean Sigma-T and salinity levels in the PSEEZ waters (26.49 and 38.32 psu, respectively) are lower than the NWL and the Nayband Bay waters. The mentioned factors reach to their maximum in NWL waters where shallower regions are present. The low levels of Sigma-T values for water mass of the PSEEZ suggest that the solid particles entering to the EHZIC would drown comparatively faster in the PSEEZ. The lower salinity levels and the lower Sigma-T values in the PSEEZ’s water mass and its deeper sedimentary beds promote the burial efficiency of carbon in these zones. This consequently leads to more effective vertical transport of the suspended solids and the associated chemicals. Reynolds (1993) has reported two local currents in the PSEEZ. West-East currents flow along the coast in the depth of less than 10 m while West-Southeast currents flow in the higher depths. In this sense, pollutants discharged in the shallow waters (≤ 10 m), will be transferred by the West-East flows to the Nayband coasts. In the case of effluent discharge at deeper depths (> 10 m), West-Southeast currents probably conduct the pollutants toward the Southeast of the study region. As a conclusion, PAHs released from the PSEEZ can travel to the Nay band area, or to the Southeast of the study region where they are probably drowned or sequestered. The horizontal transport of solid particles is more possible in the Nayband Bay and the NWL where higher Sigma-T and salinity values of water masses as well as lower depths are observable. These areas also have lesser organic matter and fine sediments as well as small number of land/sea-based sources for eco-pollutants that underpin low capacity for carbon and contaminant accumulation.
B(a)P and Nap site specific criteria
Sedimentary substrates are of great interest in pollution assessment studies. The sediments are typically the most appropriate matrix to investigate the human-induced stresses in coastal marine ecosystems. Organic pollutants and chemical contaminants tend to settle, accumulate and re-release to overlaying water layers when conditions set well together.
Shallow waters have solid particles containing enough scavengers which trap, transport and sequester a variety of lipophilic eco-pollutants. This makes contaminant concentrations to be generally higher in areas with clay enriched sediments than those in sandy regions, where routinely lower concentrations are detectable [28].
After the settlement of pollutants in sedimentary media, Equilibrium Partitioning (EqP) among the sediment, pore water and water column regulate environmental exchange. In fact, sediment toxicity can be attributed to the concentration of pollutants dissolved in the interstitial pore water, which is considered a biologically available component [29]. Some authors have demonstrated the usefulness of the EqP approach for understanding the bioavailability of PAHs in the sediments [30]. According to the EqP, the ecological role of sediments as a sink or source for pollutants is related to the compounds’ lipophilicity and sediment intrinsic characteristics, primarily its organic carbon content. Benzo(a)pyrene is carcinogenic and its toxicological data are available. The EqP approach thus was applied to derive an organic carbon site-specific sediment criterion (SSCOC) to determine the bioaccumulation status for humans. To do that, two sets of information were employed; i) B(a)P saltwater quality criteria for the protection of Human from bioaccumulation i.e., 0.0006 μg L-1 and ii) the Kow partition coefficient of B(a)P i.e, 2,238,721 L kg-1 [31-32].
Naphthalene related site-specific criterion was also examined in order to assess protection status of benthic aquatic life from Naphthalene’s chronic toxicity. Saltwater quality criteria for the protection of benthic aquatic life from chronic Toxicity of Nap and its partition coefficient are 16 μg L-1 and 2344.23 L kg-1, respectively [31].
These data were employed to calculate the organic carbon sediment criteria using the following relationship:
SCOC=WQC×Kow [Equation 2].
To apply the SCOC on a site-specific base, the organic carbon levels of sediments must be measured for the studied samples. Consequently, the organic carbon site-specific sediment criteria can be determined by the Equation 3:
SSCOC= SCOC ×TOC [Equation 3]
(Figure 4) delineates the results of estimated SSCOC. Based on these interpolated maps, sediments from the PSEEZ can sequester more ng of B(a)P and Nap in their sediments before re-releasing them in amounts exceeding ambient saltwater quality criteria. Conversely, sediments from the Nayband Bay and the intermediate zone have low capacity for keeping the mentioned compounds (Figure 4). The later regions thus have more vulnerability against the chronic toxicity of benthic fauna from Na or B(a)P bioaccumulation in humans. According to (Figure 4), if levels of B(a)P in sediments from the Nay band Bay exceeds 13 ng g-1, its sediments will re-release B(a)P in levels higher than saltwater quality criteria of that chemical for human health bioaccumulation. Figure 4b suggests that Nap related chronic toxicity is possible if levels of Nap exceeds from 3.82 ng g-1 in sediments from the Nayband Bay.
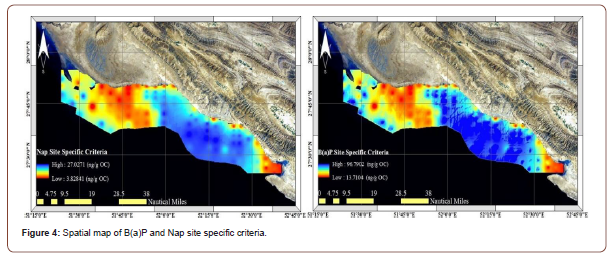
Naphthalene and B(a)P, levels and spatial distribution
Naphthalene level is between 1.27 to 264.47 ng g- dry wet
(mean= 57.02, median= 35.17) in the studied sediments. Analysis
of statistical distribution of Na levels shows that there is an obvious
positive skewness (+1.69). Besides, the third quartile of Na is 80.39
ng g- dry wet. In other words, 75% of sediments have levels below
the mentioned quartile. Thus, the examination of skewness and the
third quartile strongly suggest that there are numbers of heavily polluted sediments that greatly affects the dataset. (Figure 5) is an
interpolated map that indicates spatial distribution of Na in a continuous
surface. It should be noted that hot spots of Na levels are
in the PSEEZ region where source of PAHs are available (Figure 1).
As mentioned, the sedimentology condition of the PSEEZ (Figure
3) is suitable for accumulating of eco-pollutants. Sediment quality
guidelines (SQGs) are scientific tools to evaluate potential effects of
sedimentary eco- pollutants on marine and estuarine [33]. Effects
range low (ER-L), effects range median (ER-M), threshold effects
level (TEL), and probable effects levels (PEL) are empirical SQGs
which correlate sediment chemistry data and related biological responses
[3]. The sediment quality guidelines express those adverse
biological effects are expected rarely (
Regarding to the map of Na levels (Figure 5) and its site-specific criteria (Figure 4), a probability map for Na toxicity was depicted (Figure 6). To do that, Na values were made into a binary code (0 or 1). In indicator kriging, if values are above a threshold, they became a 1 and otherwise a 0. Predictions using indicator kriging are interpreted as the probability of exceeding or not exceeding the threshold. As we mentioned, Nap related chronic toxicity is possible if levels of Nap exceed from its site-specific criteria. In other words, chronic toxicity of Na is possible when the ratio of Na levels to its site-specific criteria exceeds the unit. Therefore, after calculating of the ratios and their introduction to ArcGIS software, the threshold value was set at 1. The resulted map highlights how the probability of Naphthalene’s chronic toxicity has been distributed in the EHZIC. Noting to the lowest level of probability (7%), leak of Na from the sediments in amounts of causing toxicity is always probable in the EHZIC. Naphthalene is a light, volatile PAH and its solvability in saltwater is 22 μg /ml 25ºC [26]. Compounds with high vapor pressor and low octanol/water coefficients have high tendency to solvent exchange process in which a compound moves easily among the compartments of environment [33]. Thus, Na can exchange in the region as a result of the mentioned process. The perfect capacity of Na for the solvent exchange promotes its leak probability in the EHZIC and justifies the observed probabilities. The PSEEZ and the Nayband Bay are the zones with the highest probability of chronic toxicity resulted from Na leak. High level of deposited Na (Figure 4) is the main factor of the observed probability in the PSEEZ. In spite of this, the Nayband Bay has low levels of Na (Figure 4). The low levels of Na also co-occur with the low levels of organic carbon (Figure. 3). With these in view, high levels of Na in the PSEEZ and low levels of organic carbon in the Bay present the higher probabilities observed. The NWL show various probabilities (Figure 6). The Motaf region and its high current speeds (Figure 2) can permanently change the levels of organic matter or Na. It consequently affects the leak of Na and the probability map.
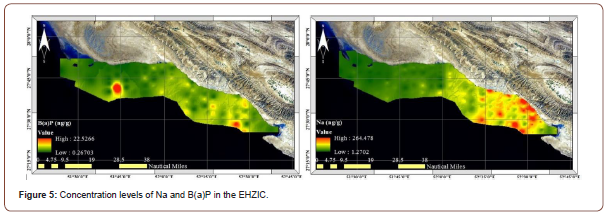
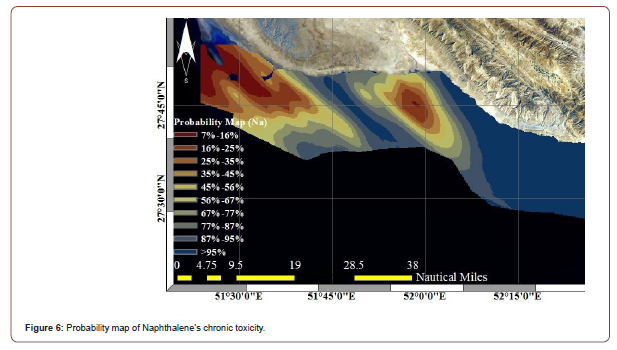
Levels of B(a)P is from 0.26 to 22.56 ng g-1 dry wet (mean= 2.69, median= 1.80) in the EHZIC. The dataset of B(a)P doesn’t follow the normal distribution (skewness= 3.52). The third quartile of B(a) P is 3.90 ng g- dry wet. It means that only a quarter of sediments have levels higher than 3.90 ng g-1 dry wet and the others have the lower values. Figure 5 delineates an interpolated map for B(a)P and demonstrates that hotspots of B(a)P pollution are mainly localized in several offshore regions. Besides, B(a)P levels are relatively higher in the PSEEZ (Figure 5) where source and sedimentological factors meet the required necessities as presented by (Figure 3). The examination of SQGs confirms that B(a)P levels are much lower than its ER-L (430 ng g-) and TEL (88.2 ng g-) levels and the biota rarely suffers from adverse effects of B(a)P.
(Figure 4) shows that the levels of B(a)P site-specific criterion for bioaccumulation status for humans are higher than Na site specific criterion. It means that B(a)P is more sequestered by the sediments before rereleasing to the extent of deriving bioaccumulation hazards. Moreover, The levels of B(a)P is virtually low (Figure 5). With this in view, the ratios of B(a)P to its site-specific site criteria would be significantly low (0.0001-0.65). Third quartile of the calculated ratios is 0.04. Hence, only a quarter of ratios are between this quartile and the maximum ratio. There is no ratio more than unit. Accordingly, the binary coding for setting of the indicator kriging is totally impossible. In other words, release of the mentioned eco-pollutant from sediments of the EHZIC is not enough to exert bioaccumulation hazards for human.
As (Figure 1) emphasizes, corals have been scattered in the PSEEZ and the Nayband national Park. The corals are the modular and sessile animals and serve as the foundation species wherever they inhabit. They are sensitive to the land-based/sea-based fluctuations (coastal development, overfishing, watershed and marine pollution). (Figure 7), adapted from Reef Base [33], delineates that the region’s corals are facing with low to medium and medium to very high threats in the cases of today’s marine pollution (A), and today’s integrated local threats (B), respectively. [34] predicted very high to Critical Threats by 2050s (C), too. This inconvenient prediction can be unfortunately enforced by chronic toxicity of Na, as well.
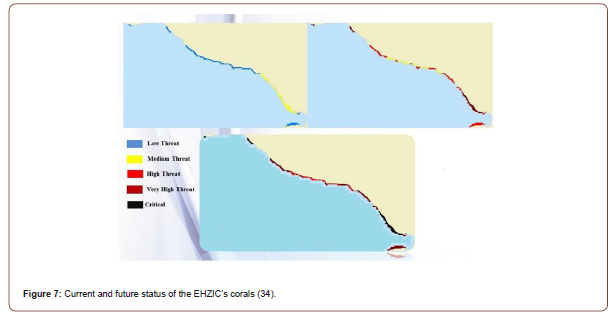
Conclusion
This study allowed us to report a strong and significant correlation among water depth, TOM and carbonate levels as well as grainsize in sediments from Environmentally Hot Zones of the Iranian Coast of the Persian Gulf.
A transfer of averagely 3.63 millimole the green house CO2 (per g dry weight) was suggested by deposition of organic matters into the sedimentary records of the region.
Spatial mappings by GIS techniques created continuous maps for water depth, grainsize, TOM and carbonate levels in the region of interest. This in turn gives us two-dimensional view and highlighted that the EHZIC can be divide to several sub-regions mainly, the NWL (the Motaf region, the Dayyer-Nakhilou national Park), the intermediate zone, the PSEEZ, and the Nayband region. Moreover, the examination of interpolated maps and the presence of heavy industries strongly introduced the PSEEZ as a region with possibly high burdens of organic carbon and its co-varying eco-pollutants.
The sedimentological maps made a question about the distribution and bio-effects of eco-pollutants in the EHZIC where a mosaic of different receiving substrates is available. The B(a)P and Nap thus evaluated pollution sequestration capacity of the study area. From the examination of site-specific criteria maps, zones of environmental vulnerability were stablished in the Intermediate and the Nayband Bay where low levels of organic matter outline more bioavailability for eco-pollutants entering. As a conclusion, in the mentioned regions, Nap and B(a)P related risks were comparatively more possible.
The sediment quality guidelines suggested that the biota of the EHZIC is suffering from B(a)P and Na related adverse hazards, rarely and rarely-occasionally.
Indicator kriging analysis and the probability map help us to summarize the probability of Naphthalene’s chronic toxicity in the EHZIC. The examinations claimed that the leak of Na from the sediments in amounts of causing toxicity is always probable in the EHZIC. According to the probability map, the PSEEZ and the Nayband Bay were the zones with the highest probability of such chronic toxicities.
The evaluation of site-specific B(a)P criterion showed that this compound could be more sequestered by the sediments before rereleasing to the extent of deriving bioaccumulation hazards. The ratios of B(a)P to its site-specific criterion were significantly lower than unit and re-release of the mentioned eco-pollutant from the sediments of interest was not probable.
As Sheppard, 1993 has emphasized, high salinity and temperature fluctuations of the Persian Gulf have forced the inhabited biota to live at the extreme limits of its ecological tolerance. Moreover, the EHZIC is a basin with enriched TOC levels which suffers from the baseline syndrome. Therefore, having a stressful, semi-enclosed basin whit a poor flushing rate and high levels of sedimentary organic matter is a matter of concern. On the other hand, the selective pressure of harsh natural conditions in combination with enriched/ polluted sediments can favor a few pollution-tolerant, r-selected opportunistic organisms, the pollu-types/pollu-species. Such naturally selected organisms are possibly able to tolerate multiple, co-occurring stressors of the Persian Gulf. Discovering and tracking of such pollu-types/pollu-species is an open field of science we must try.
Acknowledgement
We gratefully acknowledge the financial support of the Iranian National Institute for Oceanography and Atmospheric Science, INIOAS (Grant number of 392-D.P-011.02). Darya Negar Pars Consulting Engineers, Hamid Rezai Marnani, Aref Farhang Mehr, Hesam Hajimirza and Mahsa Masjedi are kindly appreciated for their cooperation. AlamTaj Aghadadashi, who did always her best, is cherished with sincere thanks.
Conflict of Interest
• All authors have participated in (a) conception and design, or analysis and interpretation of the data; (b) drafting the article or revising it critically for important intellectual content; and (c) approval of the final version.
• This manuscript has not been submitted to, nor is under review at, another journal or other publishing venue.
• The authors have no affiliation with any organization with a direct or indirect financial interest in the subject matter discussed in the manuscript
• The following authors have affiliations with organizations with direct or indirect financial interest in the subject matter discussed in the manuscript:
Vahid Aghadadashi is a faculty Member at the Iranian National Institute for Oceanography and Atmospheric Science and the work has been financially funded by the mentioned Institute (INIOAS (392-D.P-011.02).
References
- Mortimer JA, Donnelly M (2011) Eretmochelys imbricata. IUCN Red List of Threatened Species. Version 2008. International Union for Conservation of Nature.
- Sheppard CRC, Al-Husiani M, Al-Jamali F, Al-Yamani, F Baldwin, et al. (2010) The Gulf: A young sea in decline. Marine Pollution Bulletin 60(1): 13-38.
- Aghadadashi V, Mehdinia A, Molaei S (2016) Origin, toxicological and narcotic potential of sedimentary PHRs and remarkable even/odd n-alkane predominance in Bushehr Peninsula, the Persian Gul. Marine Pollution Bulletin 114(1): 494-504.
- MOOPAM (Manual of Oceanographic Observation and Pollution Analysis) (2000) Regional organization for the protection of marine environment (ROPME, Kuwait), pp. 220.
- Keshavarzifard M, Moore F, Keshavarzi B, Sharifi R (2017) Distribution, source apportionment and health risk assessment of polycyclic aromatic hydrocarbons (PAHs( in intertidal sediment of Asaluyeh, Persian Gulf. Environmental Geochemistry and Health 40(2): 721-735.
- Sheppard CRC (1993) Physical environment of the gulf relevant to marine pollution: An Overview. Marine Pollution Bulletin 27(1): 3-8.
- Vazirizadeh A, Kamalifar R, Safahieh AR, Mohammadi M, Khalifi A, et al. (2011) Macrofauna community structure of Bardestan mangrove swamp, Persian Gulf. World Journal of Fish and Marine Sciences 3(1): 323-331.
- Szava-kowats RC (2008) Grain size normalization as a tool to assess contamination in marine sediments: Is the <63 µm fraction fine enough?. Marine Pollution Bulletin 56(4): 629-632.
- Tansel A, Fuentes C, Sanchez M, Predoi K, Acevedo M (2011) Persistence profile of polyaromatic hydrocarbons in shallow and deep Gulf waters and sediments: effects of water temperature and sediment-water partitioning characteristics. Marine Pollution Bulletin 62(12): 2659-2665.
- Hyland J, Balthis L, Karakassis I, Magni P, Petrov A, et al. (2005) Organic carbon content of sediments as an indicator of stress in the marine benthos. Marine Ecology Progress Series 295(1): 91-103.
- Hargrave BT, Holmer M, Newcombe CP (2008) Towards a classification of organic enrichment in marine sediments based on biogeochemical indicators. Marine Pollution Bulletin 56(5): 810-824.
- Leong LS, Tanner PA (1999) Comparison of methods for determination of organic carbon in marine sediments. Marine Pollution Bulletin 38(10): 875-879.
- Heiri O, Lotter AF, Lemcke G (2001) Loss on ignition as a method for estimating organic and carbonate content in sediments: reproducibility and comparability of results. Journal of Paleolimnology 25(1): 101-110.
- Wang Q, Li Y, Wang Y (2011) Optimizing the weight loss-on-ignition methodology to quantify organic and carbonate carbon of sediments from diverse sources. Environmental Monitoring and Assessment 174(1-4): 241-257.
- Mathews CP, Kedidit S, Fita NI, Al-Yahya A, Al-Rashid K (1993) Preliminary assessment of the effects of the 1991 Gulf War on Saudi Arabian prawn stocks. Marine Pollution Bulletin 27(1): 251-271.
- Mehdinia A, Aghadadashi V, Sheijooni-Fumani N (2015) Origin, distribution and toxicological potential of polycyclic aromatic hydrocarbons in surface sediments from the Bushehr coast, the Persian Gulf. Marine Pollution Bulletin 90(1-2): 334-338.
- Al-Abdali F, Massoud MS, Al-Ghadban AN (1996) Bottom sediments of the arabian gulf-III. Trace metal contents as indicators of pollution and implications for the effect and fate of the Kuwait oil slick. Environmental Pollution 93(3): 285-301.
- Delshab H, Parvin Farshchi, Behnam Keshavarzi (2016) Geochemical distribution, fractionation and contamination assessment of heavy metals in marine sediments of the Asaluyeh port, Persian Gulf. Marine Pollution Bulletin 115(1-2): 401-411.
- Massoud MS, Al-Abdali F, Al-Ghadbanb AN, Al-Sarawi M (1996) Bottom sediments of the Arabian Gulf-II. TPH and TOC contents as indicators of oil pollution and implications for the effect and fate of the Kuwait oil slick. Environmental Pollution 93(3): 271-274.
- Mortimer JA, Donnelly M (2008) Eretmochelys imbricata. IUCN Red List of Threatened Species. Version 2008. International Union for Conservation of Nature.
- Agah H, Rahmanpour S, Sheijooni Fumani N (2013) Organic carbon and organic matter levels in sediments of the Strait of Hormoz, the Persian Gulf. The Journal of Persian Gulf 4(13): 31-37.
- Agah H, Hashtroudy MS, Baeyens W (2012) Trace metals and major elements in sediments of the Northern Persian Gulf. The Journal of Persian Gulf 3(7): 45-58.
- Reynolds RM (1993) Physical oceanography of the Gulf, Strait of Hormuz, and the Gulf of Oman - Results from the Mt. Mitchell Expedition. Marine Pollution Bulletin 27(1): 35-59.
- Burdige DJ (2007) Preservation of organic matter in marine sediments: Controls, mechanisms, and an imbalance in sediment organic carbon budgets?. Chemical Reviews 107(2): 467-485.
- Arndt S, Jórgensen BB, Larowe DE, Middelburg JJ, Pancost RD, et al. (2013) Quantifying the degradation of organic matter in marine sediments: A review and synthesis. Earth Science Reviews 123(1): 53-86.
- Hashtroudi MS, Aghadadashi V (2018) Sediment-associated polycyclic aromatic hydrocarbons and potential eco-hazards in Chabahar Bay, Iran. Marine Pollution Bulletin 129(5): 875-883.
- US EPA (1991) Proposed technical basis for establishing sediment quality criteria for nonionic organic chemicals using equilibrium partitioning. US Environmental Protection Agency, Office of Science and Technology, Health and Ecological Criteria Division, Washington, DC 20460.
- Kersten M, Smedes F (2002) Normalization procedures for sediment contaminants in spatial and temporal trend monitoring. Environmental Monitoring 4(1): 109-115.
- US EPA (2002) Mid-Atlantic Integrated Assessment (MAIA) Estuaries 1997-1998
- Hawthorne SB, Miller DJ, Kreitinger JP (2006) Measurement of total polycyclic aromatic hydrocarbon concentrations in sediments and toxic units used for estimating risk to benthic invertebrates at manufactured gas plant sites. Environmental and Toxicological Chemistry 25(1): 287-296.
- Pataki GE, Cahill GP (1999) Technical Guidance for Screening Contaminated Sediments. New York State Department of Environmental Conservation Division of Fish, Wildlife and Marine Resources.
- Wania F (2006) Potential of degradable organic chemicals for absolute and relative enrichment in the Arctic. Environment and Science Technology 40(2): 569-577.
- Macdonald RW, Mackay D, Hickie B (2002) Contaminant amplification in the environment. Environmental Science and Technology 36(23): 457-467.
- Tupper M, MK Tan, SL Tan, MJ Radius , S Abdullah (2011) Reef Base: A global information system on coral reefs.
-
Vahid Aghadadashi, Saeideh Molaei, Ali Mehdinia. Sediment Characteristics and Estimation of Toxicity Site Specific Criteria for Sediments of Iranian Coasts, The Persian Gulf. Ad Oceanogr & Marine Biol. 3(2): 2022. AOMB.MS.ID.000557.
-
Grainsize, Organic matter, Pars special economic energy zone, Persian gulf, Probability map, Sediment
-
This work is licensed under a Creative Commons Attribution-NonCommercial 4.0 International License.