Research Article
Evaluation of the Marine Impacts Resulting from the Using of Different Chemical Biocides in Cooling Water System of New Nuclear Power Plant
Mohamed Safwat Mohamed Tawfik, Associate Professor in Radiochemistry, Egypt.
Received Date:September 06, 2023; Published Date:September 27, 2023
Abstract
The objective of cooling water treatment is prevention of corrosion, scaling and fouling of cooling water systems. A successful water treatment
would appropriately suit the system metallurgy, operating conditions and the water used. In addition, the Program must be consistently applied
and monitored. Methods used different chemical and biological equations to manage the marine impacts resulting from the using of different types
of biocides as antifouling in cooling water system of nuclear power plant. Results showed that Using biocides is added to the incoming seawater, in
order to prevent biofouling in cooling water system, the complexity of a biocide reaction with different species that exist naturally in seawater is that
it produces different by-products. It is necessary to identify the most effective by-product in controlling bio-film development. Using chlorination
in seawater produces compounds that are toxic to aqueous life. These compounds’ concentrations and lifetimes are functions of pH, temperature,
salinity, dissolved organic matter and nitrogen, inorganic nitrogen and the amount of chlorine and mixing efficiency. The application of using
combining sodium hypochlorite with quaternary ammonium salt can be more effective in the killing of micro-fouling when using the seawater as
cooling system. Using chlorine dioxide as oxidizing alternative than sodium hypochlorite because of the following advantage:
a) Chlorine dioxide is effective over a broad pH range,
b) It does not react with organics to form THMs,
c) it does not react with ammonia-nitrogen,
d) it is effective at lower dose rates in contaminated systems compared to alternative biocides,
e) it does not react with triazole corrosion inhibitors, and
f) Chlorine dioxide may reduce the requirement for microbial control chemicals in the cooling water.
Keywords:Biocides; Marine impacts; Antifouling; Scale formation
Aim of the Work
Aim of the work is to manage the impacts resulting from the using of different types of biocides as antifouling in cooling water system of nuclear power plant.
Methods
This review illustrates the different types of cooling water systems in nuclear power plants. Showing the different types of biocides, focusing on the common types used in cooling water system of nuclear power plants, and evaluating the impacts resulted from using the common types of biocides in the cooling water systems with different water sources.
Introduction
The cooling system or the circulating water system provides a continuous supply of cooling water to the main condenser to remove the heat rejected by the turbine and auxiliary systems (e.g., the turbine bypass system) in conventional and nuclear power plants. In this process the cooling water becomes hot, and this energy is rejected into the atmosphere via cooling towers or directly to the seawater or to a river. Biocides are chemical substances used to control biofouling in heat exchangers by killing the living organisms. However, chlorine is the most widely used chemical for disinfecting in water treatment, but there is a high risk of the potential production of hazardous halogenated byproducts. Biocide is also called germicide and algaecide, micro biocides, or fungicide. The biocide used in the cooling water system is referred to as the cooling water biocide. According to the incomplete statistics, nearly 80 kinds of biocides, generally divided into oxidizing and non-oxidizing biocide two categories, are used in the current domestic industrial circulating cooling water. The oxidizing biocide used commonly includes chlorine, hypochlorite such as sodium hypochlorite and calcium hypochlorite etc., chlorine dioxide, ozone, bromine, and bromide.
The non-oxidizing biocide used commonly includes chlorine
phenol such as double chlorophenol, trichlorophenol and pentachlorophenol,
quaternary ammonium salt such as benzalkonium
chloride (geramine), organic amine such as rosin amine, etc., organic
sulfur compounds such as methylene sulfur cyano, etc., copper
salts such as copper sulfate and quaternary phosphorus salt [1].
There are many classified products of biocides as following groups:
The cooling system or the circulating water system provides a continuous
supply of cooling water to the main condenser to remove
the heat rejected by the turbine and auxiliary systems (e.g. the turbine
bypass system), in conventional and nuclear power plants. In
this process the cooling water becomes hot. This energy is rejected
to the atmosphere via cooling towers or directly to the seawater
or to a river as shown in Figures [1&2]. Note that, not all nuclear
power plants have cooling towers and conversely, the same kind
of cooling towers are often used at large coal-fired power plants as
well [3]. Fouling is the depositing of accumulation of particles or
material on mechanical systems. Effects of Fouling:
a) Reduce heat transfer efficiencies,
b) Decrease fluid flow,
c) Increases system pressures,
d) Increases operational cost [3].
Using seawater in cooling systems is a common practice in many parts of the world where there is a shortage of freshwater.
However, bio fouling is one of the major operational problems associated with the usage of seawater in cooling systems. Micro fouling is caused by the activities of microorganisms, such as bacteria and algae, producing a very thin layer that sticks to the inside surface of the tubes in heat exchangers. This thin layer has a tremendously negative impact on heat transferred across the heat exchanger tubes in the system. In some instances, even a 250-micrometer thickness of fouling film can reduce the heat exchanger’s heat transfer coefficient by 50%. On the other hand, macro fouling is the blockage caused by relatively large marine organisms, such as oysters, mussels, clams, and barnacles. A biocide is typically added to eliminate, or at least reduce, bio fouling. In some cases, excessive bio fouling can obstruct the heat exchangers. To prevent this, biocides are used to control the biological activities of microorganisms and lessen the effect of fouling. Controlling microbial growth is usually achieved by using an oxidizing agent, such as chlorine, in an easy-to-disperse form, such as a hypochlorous acid or a hypochlorite ion, or in a gaseous form like chlorine gas or chlorine dioxide. An intermittent chlorine dosage of 2-5mg/L for 10 minutes per day can control microfouling, and a continuous dosage of 0.5 mg/L during the second to fourth week of breeding season can control the blockage caused by macrofouling. Under a continuous biocide dosage, aquatic organisms like oysters and mussels tightly close their shells and often die of asphyxiation [4]. These chlorine forms are most widely used due to cost and effectiveness factors. intermittent dosages, and macrofouling can be controlled by continuous dosages of biocide. Chlorine is a nonselective oxidant (it reacts with organics and inorganics), and it deactivates microbes [5].
Also, chlorine reacts with natural organic matter (NOM), leading to the formation of numerous by-products. Some of these byproducts are hazardous to aquatic life and human health. While there are other means of preventing biofouling, such as periodic cleaning with sponge balls, tube heating and drying, and antifouling paint, nonetheless, chlorine dosing is the most widely used method because of its cost-effectiveness and efficiency in controlling different organisms that cause fouling. Because of the strong interaction between the process cooling demand, operating conditions, and biocide needs and performance, it is important to develop an integrated approach to optimizing biocide usage and discharge by understanding the key process factors and seawater chemistry aspects, then reconciling them in an effective manner. The objective of this paper is to develop a systematic approach to the optimization of biocide usage and discharge by integrating seawater chemistry and process performance issues. This includes modeling the mechanism and kinetics of the biocide, relating the biocide kinetics to process conditions, and reducing biocide usage by lowering the cooling needs of the process via heat integration. The usage and discharge of seawater is linked to the process requirements, including cooling duties. So, any reduction in cooling duties will have a direct impact on the usage and discharge of both seawater and biocide [6].
There are some factors to be considered when considering biocides
dosing in sea water include
1) Biocide application rates and frequency,
2) Use of bio-dispersants or other cleaners,
3) Retention time and water velocity through the system,
4) Cooling system pH, chlorides, TDS, or other pertinent analytical
parameters, including any process contaminant load or
cooling system nutrients,
5) Microbiological flora load, including slimes, bio films, etc.,
6) 6)The compatibility and possible effects on other chemical inhibitors,
7) Cost comparisons of a proposed biocide with other possible
starting-point biocides,
8) Existing corrosion, deposits and other reported problems [7].
Several seawater characteristics impact biocide chemistry. These include concentration of ammonia, bromide, and organic carbon, pH, and salinity. Ammonia concentration in seawater typically ranges from 1.0 ppb to 1.0 ppm, and as the salinity increases, the ammonia concentration decreases. As the ammonia concentration decreases, it causes a shift from combined oxidants to free oxidants (e.g., HOBr). Bromide concentration is very low in freshwater but can go up to 65 ppm in high salinity seawater [8]. As organic carbon content decreases, there is a corresponding reduction in demand for the biocide dosage. There are un-reactive chemical constituents in marine or estuarine waters from chlorination. These constituents, such as sulfur, manganese ion, and iodide, play a key part in the chlorination of seawater. Components such as (Org-C, NO2-N, S, Mn, Fe) are oxidized to inert products like carbon dioxide and carboxylic acids, which help chlorine residuals disappear [9,10]. At low ammonia-nitrogen concentration and pH 6-8, the important oxidant species are HOBr, OBr-, and NBr3. But at high ammonia- nitrogen levels and same pH range (6-8), the important oxidant species is first NHBr2 and then NH2Br and NH2Cl [11]. Chlorination of river water produces predominantly CHCl3, while chlorination of sea or estuarine water would produce mainly CHBr3. Chlorination of seawater, with NaCl to 5 mg/liter as Cl2, at pH 8.1 and 0.01 mg/liter of natural ammonia nitrogen, would produce CHBr3 in one hour as the only trihalomethane. This shows that chloramines do not react with organic matter during water treatments. Chlorination of marine water with more than 3 g/Kg and at pH range 6-8 would produce five important oxidants (HOBr, OBr-, NH2Br, NHBr2, NBr3. At conditions where NH4 is rich in seawater, trihalomethane yield would become less, and bromamines would replace HOBr to reach equilibrium, and NH2Cl would become dominant. Residual oxidants decay slowly in high salinity water.
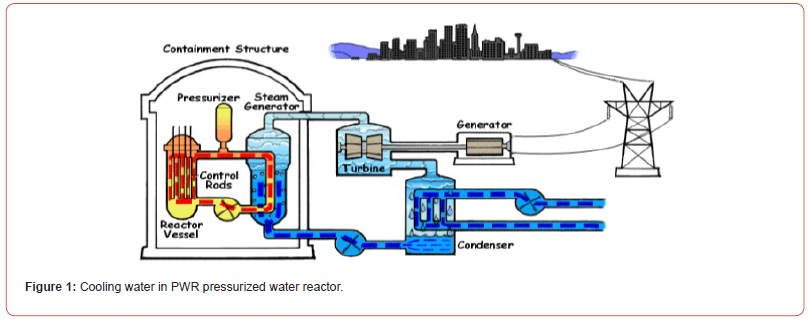
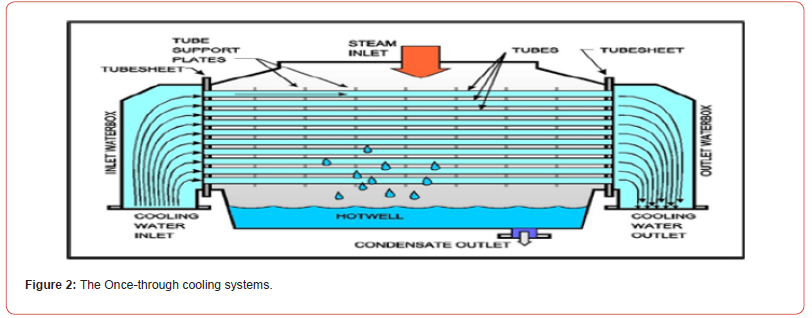
Table 1:The consumption and withdrawal amount of water for different types of power plants using once through cooling system.

Table 2:Common Types of biocides used in cooling water systems.
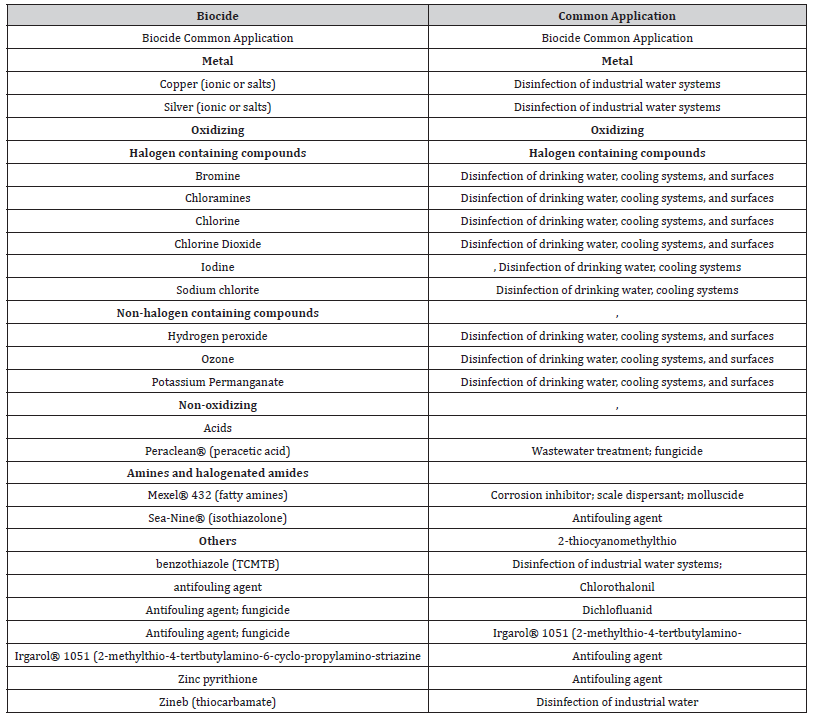
Negative impacts of using copper salts biocides on another aquatic biota
Copper effect on algae
Copper was found to be toxic for some algae. It can inhibit growth of Chlorella vulgaris and Dunaliella tertiolecta when concentration is about 4μg/L and 600μg/L respectively. Moreover, the photosynthetic activity can be blocked from 50μg/L for Chlorella pyrenoidosa, and visible lesions appear in macroalgae from 100μg/L. Molluscs are moderately sensible to copper oxides such as CuO and Cu2O when concentrations are between 1 and 2mg/L [12].
Copper effect on marine crustacean species
Copper can kill 50% of the population of different crustacean species after only 48h of exposure. Larvae are more sensitive than adults with respective LD50s 0.6 and 109mg/L for crab Carcinus maenas, values of 0.3 and 29.5mg/L were recorded and for shrimp Crangon 0.1 and 0.3mg/L for lobster larvae Homarus gammarus [13].
Copper effect on fishes
After 96 h of exposure, copper reduces survival of some kinds of fishes by 50% at a low concentration 84.4 μg/L [14].
Negative impacts of using Chlorothalonil biocides on another aquatic biota
Toxicity of Chlorothalonil as anti- bio-fouling compound
Chlorothalonil antifoulant has previously been reported to be highly effective against several marine species such as crustaceans, invertebrates, and fishes [15].
Chlorothalonil effect on invertebrates
The toxicity of this biocide to aquatic invertebrates is unclear. Some studies show that chlorothalonil is the most toxic compound to the early developmental stages of species of marine invertebrates: The consequences of applying chlorothalonil on invertebrates were noted to be embryo toxicity, larval inhibition and mortality. Chlorothalonil also affects the shell deposition of Crassostrea virginica at low concentrations (between 5μg/L and 26μg/L) [15].
Chlorothalonil effect on crustaceans
Many crustacean species have been studied and have been found to be very sensitive to Chlorothalonil such as Daphnia magna. Furthermore, for three life history stages (embryo, larvae, adult) of the grass shrimp Palaemonetes pμgio, studies show that embryos are the least sensitive and that larvae are the most sensitive to chlorothalonil exposure, larvae exposed to a concentration between 250μg/L and 500μg/L become increasingly less active and remains motionless on the bottom of the beakers until death [16].
Optimization of using biocides in once through seawater cooling system with economic and environmental consideration
Using seawater in a once through cooling system as a type of cooling water system can lead to biofilm formation in the condenser tubes. Using biocides is added to the incoming seawater, in order to prevent bio fouling in cooling water system, the complexity of a biocide reaction with different species that exist naturally in seawater is that it produces different by-products. It is necessary to identify the most effective by-product in controlling biofilm development. In the meantime, any hazardous compounds produced throughout the cooling system until the discharge point must be monitored. Sometimes there are regulations on the propagation of the compounds at a certain radius from the discharge point. On the other hand, the usage of seawater is linked to the process requi requirements, including cooling utilities, as shown in (Table 1). Therefore, any reduction in cooling requirements will have a direct impact on the amount of seawater used and discharged, along with the usage and discharge of biocide. In order to successfully achieve that reduction in seawater and biocide, several design and operational changes must be made. Understanding the chemistry of the biocide in seawater and integrating it with the performance of the process. Also, we must try to integrate process units and streams to reduce seawater usage and discharge along with the usage and discharge of biocide and its by-products.
Reducing the cooling duties of the process will influence in lowering the usage and discharge of seawater, and, consequently, the discharged biocide will be reduced. There are two advantages of reducing cooling duties: cost-savings and pollution prevention. Further reduction in the usage of seawater is achieved by a more effective heat transfer, which would lead to a reduction in the amount of biocide discharged. Adding new biocide removal units (e.g., de-chlorination) must be considered and reconciled with all the approach steps, like reduction in cooling utilities and seawater flow rate, and optimizing biocide dosage. The philosophy behind this proposed design is that any reduction in cooling duties will result in lowering the usage and discharge of seawater, along with the usage and discharge of biocide. A further reduction in usage of seawater can be made by maximizing the span temperature of discharged seawater. The excess amount of biocide and its by-product before the discharge point can be treated by the conventional approach: a biocide removal unit [17]. The biocide dosage is proportional to the cooling duty. As such, reducing the cooling utility of the process leads to reducing the usage and discharge of the biocide. The rationale behind starting with heat integration is that it will generate cost savings from the reduction of cooling utilities. Heat integration is the synthesis between process streams to feasibly exchange heat between hot process streams and cold process streams. Thus, the outsources for heat and cooling are reduced. Therefore, heat integration creates an economic advantage by the savings made from fewer dependents on the outside source of heating and cooling. At the same time, this is a more environmentally friendly process. The heat integration thermal pinch analysis will define the minimum amount heating and cooling duties that can be achieved. The next step is to make a further reduction in seawater flow rate by enhancing the efficiency of seawater utilization [17].
The least complicated technique to reach that reduction is to maximize the discharged seawater temperature while complying with the environmental thermal pollution regulations. Then, the new seawater flow rate from the heat balance equation can be calculated after the reduction has been made from increasing the discharged temperature. After the reduction has been achieved in the seawater flow rate, the revised calculation can be performed by recalculating the reduced amount of biocide required for the seawater flow rate. The following step is to optimize the dosage of biocide, while meeting the requirements of the process of the minimum residual biocide remains in the cooling system to ensure the control over any bio fouling development. At the same time, reducing the amount of biocide and its by-products that are discharged back to the seawater saves aquatic life. The optimal biocide dosage is based on the knowledge of biocide chemistry. Chlorine will dissolve and hydrolyze rapidly (almost instantaneously) and completely to HOCl (hypochlorous) acid with a reaction rate constant [18]. Impacts of seawater d When chlorine is added to seawater, it produces hypochlorous acid and hydrochloric acid, HCl.
The latter is a relatively strong acid, and it will dissociate completely to a hydrogen ion and a chloride ion, while hydrochlorous acid, HOCl, is a relatively weaker acid, and it will dissociate slightly to a hydrogen ion and a hypochlorite OCl-. Since the hydrogen ion appears in this reaction, this equilibrium is pH dependent. The distribution of the various species (HOCl, OCl-) at equilibrium is a function of pH. Hypochlorous acid is the most germicidal species, but it is a weak acid that will dissociate to hydrogen and hypochlorite ions. In terms of disinfection effectiveness, hypochlorous acid is much stronger (almost two orders of magnitude) than the hypochlorite ion. Hypochlorous acid will reach its maximum concentration at pH ranges between 4 and 6. However, the effectiveness of a chemical species as a disinfectant does not necessarily correlate with its effectiveness in removing biofilms. Weakening the polysaccharide matrix of microbial cells is one way to control the development of biofilms on the tube surface of heat exchangers. There is experimental evidence that shows that chlorination is more effective in causing biofilm detachment at pH values greater than pH 8, Naturally, seawater contains organic and nonorganic species. Of particular importance are ammonia and bromide species. Their concentrations vary depending on the nature of the water [18].
For instance, seawater contains bromide with concentrations as high as 65mg/l, but it will go as low as a negligible amount in fresh waters. Ammonia concentrations depend on pollutants of the water. In highly polluted water, ammonia concentration is as high as 15 ppm, while in unpolluted water the concentration is lower than 0.05mg/l. At full strength salinity, seawater ammonia concentration ranges between 0.001 and 1.0ppm. Ammonia, as well as other reactive nitrogenous compounds, will be chlorinated to yield monochloramine (NH2Cl), and will react further to produce dichloramine (NHCl2). In freshwater, and a very traceable amount in seawater, dichloramine will react further with hypochlorous acid, producing trichloramine (NCl3). All of the reactions of ammonia and combined chlorine with hypochlorous acid are made by replacing the hydrogen atom of the ammonia molecule with a chlorine atom from a combined chlorine molecule. These reactions depend on pH, temperature, and contact time, but mainly on the chlorine to ammonia ratio. All the free chlorine (hypochlorous acid) will be converted to monochloramine at pH 7-8 (the fastest conversion is at pH 8.3) when there is 1:1 molar ratio of chlorine to ammonia (5:1 by weight) or less.
Then, within the same range of pH, dichloramine is produced at a molar ratio of 2:1 of chlorine to ammonia (10:1 by wt.). This reaction is relatively slow, so it may take up to an hour. Also, within the same range of pH, trichloramine will be produced at a molar ratio of 3:1 of chlorine to ammonia (15:1 by wt.) and at equal molar ratios, but at pH 5 or less. The two reactions producing di- and tri-chloramine are known as the breakpoint reactions where the chloramines are reduced suddenly to the lowest level. The significance of the breakpoint reaction is that chlorine reaches its highest concentration and germicidal efficiency (at 1:1 molar ratio of chlorine to ammonia) just before reaching this point. Also, at the breakpoint, monochloramine and dichloramine react together (which reduces chlorine residuals) to produce nitrogen gas, nitrate, and trichloramine. Dichloramine decomposes to an intermediate reactive product (NOH) which consumes mono-, di-chloramine, and hypochlorous acid, producing nitrogen gas and nitrate. Also, excessive chlorine will form trichloramine [19].
Using chlorine dioxide as alternative in the case of optimization of biocides
Chlorine dioxide (ClO2) is effective as both a disinfectant and an oxidant in water treatment. Chlorine dioxide is a broad-spectrum micro biocide effective over a wide pH range. Unlike chlorine, chlorine dioxide does not react with organic materials to form trihalomethanes (THMs). Chlorine dioxide is also non-reactive with ammonia-nitrogen and with most treatment chemicals (corrosion and scale inhibitors) present in cooling water systems. Chlorine dioxide is effective in the control of microbiological growth in industrial cooling waters under conditions unfavorable to chlorine. It is particularly effective in systems having a high pH, ammonia-nitrogen contamination, persistent slime problems, or where the microbial contamination is aggravated by contamination with vegetable or mineral oils, phenols or other high chlorine-demand producing compounds [20]. Chlorine dioxide’s chemistry is best summarized not by what it reacts with so much as by what it does not react with. Chlorine dioxide does not react with water nor does its chemical form or biocidal activity change with changes in pH. Chlorine dioxide also does not react with ammonia nitrogen or most organic contaminants and treatment chemicals present in the cooling water. Consequently, the dosage required for biocidal control remains fairly constant over a wide range of cooling water conditions. This makes chlorine dioxide an excellent choice for cooling water that has a high pH, or that has high levels of organic or ammonia contamination. Chlorine dioxide is generally applied directly into the suction of the cooling system’s recirculating pump. Alternatively, it may be applied beneath the water in the sump as close to the pump intake as possible. Depending upon how critical the cooling system is, two different treatment schemes may be used: an intermittent treatment scheme and a continuous treatment scheme. Since both treatment schemes are based upon establishing a chlorine dioxide residual, to be effective the chlorine dioxide demand of the cooling water must be known. Typically, the demand is determined over a 5-minute period. The relationship between chlorine dioxide demand dose and residual is as Follows [21]. Residual = Dose – Demand (1)
A comparison of chlorine dioxide and other oxidizing biocides should be conducted from the perspective of performance rather than on a cost per pound of chemical basis. When chlorine dioxide is scrutinized in this way, it becomes more cost competitive with alternative biocide programs, especially in systems, which operate at high pH or are contaminated with organics. The higher the con tamination level the more economically viable chlorine dioxide becomes. For a clean system, the amount of chlorine dioxide required to treat a system would commonly be 1/4 to 1/5 of that of chlorine. For a contaminated system, the amount required may be as low as 1/20. (Figure 1) gives an indication of chlorine dioxide economics versus the economics of other common oxidants based on system contamination.
Advantages of Chlorine Dioxide
It was observed that many advantages could be observed through the using of chlorine dioxide ; as chlorine dioxide is effective over a broad pH range, Chlorine dioxide does not react with organics to form THMs, Chlorine dioxide does not react with ammonia- nitrogen, Chlorine dioxide is effective at lower dose rates in contaminated systems compared to alternative biocides, Chlorine dioxide does not react with triazole corrosion inhibitors, Chlorine dioxide is very effective for biofilm and algae control, and Chlorine dioxide may reduce the requirement for microbial control chemicals in the cooling water.
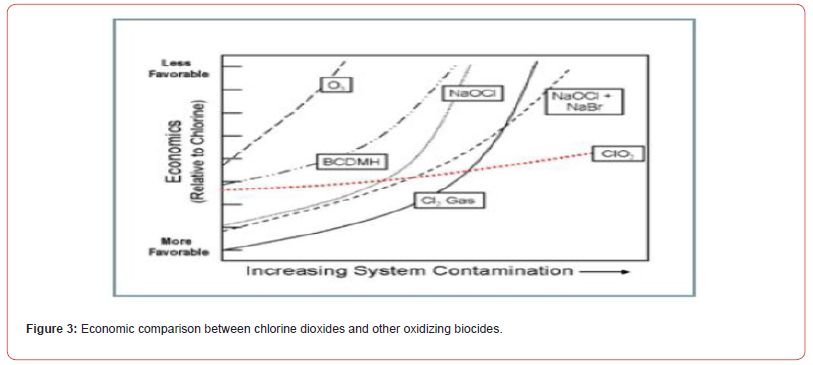
For control of bacterial slime and algae in industrial recirculating and one-pass cooling systems, the required dosages will vary depending on the exact application and the degree of contamination present. The required chlorine dioxide residual concentrations range between 0.1 and 5.0 mg/L. Chlorine dioxide may be applied either continuously or intermittently. The typical chlorine dioxide residual concentration range is 0.1-1.0 mg/L for continuous doses, and 0.1-5.0 mg/L for intermittent doses. The minimum acceptable residual concentration of chlorine dioxide is 0.1 mg/L for a minimum one-minute contact time [22].
Method of Feed of chlorine dioxide
Chlorine dioxide is a gas produced by activating sodium chlorite with an oxidizing agent or an acid source. Sodium chlorite is converted to chlorine dioxide through a chlorine dioxide generator and applied as a dilute solution. Chlorine dioxide solutions should be applied to the processing system at a point, and in a manner which permits adequate mixing and uniform distribution. The feed point should be well below the water level to prevent volatilization of the chlorine dioxide [23].
Using different mathematical equations in the case of optimization of biocides
All scenarios from the chemical dosing of treatment and post-treatment, and all the costs incurred from heating loss due to fouling and heat exchanger cleaning, have to be incorporated in the decision for any design or operation changes. The objective function is built on the economic criterion. It will permit the most feasible solution that would also cost the least to operate the plant. This approach might allow for biofilm growth and removal by periodic heat exchanger cleaning at the minimum overall operating cost. On top of that, there are a variety of options for chemical dosing. For each dosing course, the response of bio fouling development will vary accordingly. Thus, there will be an impact on the other operating costs. Also, the nitration and the ambient temperature variations through different seasons of the year will govern the microorganisms’ behavior inside the cooling system. All the different aspects must be integrated to make one plan that considers the impact on all of the related design and operation changes. The dynamics of the process itself will add difficulty to selecting the optimal scenario among the options. The objective function is to minimize the annual total cost that is incurred directly and indirectly from fouling. It will include the annual operating cost of chemicals (chlorine) used to treat the cooling water against bio fouling, the annual fixed and operating costs of removing chemical residuals from the effluent water, the cost of cleaning heat exchanger units, the cost of the loss incurred by bio fouling, and the annual gross profit loss from shutting down the operation for cleaning the heat exchanger [24].
Annual Total Cost = Operating Cost of Chemicals to Treat the
Water + Annualized Fixed and Operating Costs of Chemicals for Removing
Residuals + Annual Heat Exchanger Cleaning Cost + Cost of
Heat Loss Due to Fouling + Gross Profit Loss Due to Shutting Down
the Process for Heat Exchanger Cleaning [25]. The mathematical relation
which can be linear, that represents the relationship between
the rate constants with chemical concentration is:
K= a* Cch + b (2)
The disinfection rate constant, K, must be calibrated by ϕ which
can be determined by dosing the plant that is going to use this model
with a certain chemical concentration for one hour per day and
developing a graph from the data collected to represent the biofilm
thickness versus time in days. By using a regression analysis for the
curve, the destruction rate constant can be determined. From the
disinfection rate constant K and destruction rate constant at the
same dosage, ϕ can be calculated as follows:
γ = ϕ* k (3)
The chemical residual concentration, C ChRs, at the effluent before
discharge or treatment can be determined by developing a kinetic
model of the process. The consumption of chemicals is due to
the reaction with organic and nonorganic compounds, biofilm, and
corrosion. The chemical decay in kinetics in a batch cooling system.
May be described as follows:
dCch/ dtOp = KW*CW /γ h – W/ γ h -KbC (4)
Based on examining numerous experimental results for the kinetics of seawater treated with a chemical (i.e., chlorine), decay kinetics have been correlated to several factors, including temperature, pH, and contact time, but mainly on the ratio of chlorine dosage to ammonia. The reduction or decay of chlorine in seawater is due to reactions with organic and nonorganic compounds in seawater. The chlorine decay occurs in three stages, starting with a very fast rate during the first two minutes due to a reaction with inorganic reducing agents. The second phase is slower and usually does not last more than two hours. It mainly involves reactions with the organic compounds that started in the first phase. Then, chlorine decays continuously at a very slow rate. Of particular importance is the dependence on residence time and temperature [26].
Conclusion and Recommendation
Using seawater in a once through cooling system as a type of
cooling water system can lead to biofilm formation in the condenser
tubes. Using biocides is added to the incoming seawater, in order to
prevent bio fouling in cooling water system, the complexity of a biocide
reaction with different species that exist naturally in seawater
is that it produces different by-products. It is necessary to identify
the most effective by-product in controlling bio film development.
Using chlorination in seawater produces compounds that are toxic
to aqueous life. These compounds’ concentrations and lifetimes are
functions of pH, temperature, salinity, dissolved organic matter and
nitrogen, inorganic nitrogen and the amount of chlorine and mixing
efficiency. The application of using combining sodium hypochlorite
with quaternary ammonium salt can be more effective in the killing
of micro fouling when using the seawater as cooling system. Using
chlorine dioxide as oxidizing alternative than sodium hypochlorite
because of the following advantage:
a) Chlorine dioxide is effective over a broad pH range,
b) It does not react with organics to form THMs,
c) it does not react with ammonia-nitrogen,
d) it is effective at lower dose rates in contaminated systems compared
to alternative biocides,
e) it does not react with triazole corrosion inhibitors, and
f) Chlorine dioxide may reduce the requirement for microbial
control chemicals in the cooling water.
Ethics Approval
All of the experimental protocols were not needed to get ethic approval as there were no experimental animals used.
Acknowledgement
None.
Conflict of Interest
No conflict of interest.
References
- Xuejun Xie, Yuan-lin Zhang, Yu Zhang, Hao Fu, Mao-cai Gong, at al., (2017) Study on the Application of Biocides in the Seawater Cooling System and Their killing Effect in the Laboratory. International Conference on Manufacturing Engineering and Intelligent Materials (ICMEIM).
- Biocide Standards Reference Guide 3 Accu Standard is accredited to ISO Guide 34, ISO/IEC 17025 and certified to ISO 9001. ISO/IEC.2014. 125 Market Street New Haven, CT 06513 USA. pp 1-25.
- K. D. Zammit (2012) Water Conservation Options for Power Generation Facilities, POWER 156(9): 54-58.
- Freese, Freese SD, Nozaic DJ (2007) Chlorine Based Disinfectants: How Do They Compare? Water Science & Technology 55 (10): 403-441.
- Bin Mahfouz A, Atilhan S, El-Halwagi M, Batchelor B, Linke P, et al. (2009) Process integration and kinetic analysis of seawater cooling in industrial facilities: Design for energy and the environment, Proceedings of the7th International Conference on the Foundations of Computer Aided Process Design. El-Halwagi M, Linninger A, Taylor and Francis, London, England, pp 223-231.
- Ben Waren A, Heitz A, Joll C, Kagi R (2006) A new method for calculation of the chlorine demand of natural and treated waters. Water Res 40(8): 2877-2884.
- Intissar Amaraa, Wafa Mileda, Rihab Ben Slamab, Neji Ladharic (2018) Antifouling processes and toxicity effects of antifouling paints on marine environment. A review. Environ Toxicol Pharmacol 57: 115-130.
- Colin Frayne (2001) Cooling Water Treatment. Principles and Practices the Selection and Application of Nonoxidizing Biocides for Cooling Water Systems. Metro Group, New York.
- Pinkernell U, Nowack B, Gallard H, Gunten U (2008) Methods for the photometric determination of reactive bromine and chlorine species with ABTS. Water Research 34(18): 4343-4350.
- Rajagopal S, Velde GVD, Venugopalan VP, Jenner HA (2005) Chlorine and Chlorine Residuals, Water Encyclopedia, John Wiley & Sons, New York, NY pp 23-34.
- Helz R, SUgam R (2005) Chlorine specification in seawater. Chemosphere 10(1): 41-57.
- Sayato Y, Moto T, Ueno H (2006) Disinfection by products in the chlorination of organic nitrogen compounds, Water Supply 13(3): 171-176.
- Bin Mahfouz A, El-Halwagi M, Batchelor Bill, Atilhan S, Linke P, et al., (2009) Process integration and kinetic analysis of seawater cooling in industrial facilities, Design for Energy and the Environment, Water Chlorination: Environmental Impact and Health, Ann Arbor Science, Ann Arbor, MI 3: 349-368.
- Haas CN (2002) Benefits of using a disinfectant residual. American Water Works Association 91(1): 65-69.
- Verween A, Vincx M, Degraer S (2009) Comparative toxicity of chlorine and peracetic acid in the biofouling control of Mytilopsis leucophaeata and Dreissena polymorpha embryos. International Biodeterioration & Biodegradation 63(4): 523-528.
- Lattemann S, Hopner T (2008) Impacts of seawater desalination plants on the marine environment of the gulf, Protecting the Gulf’s Marine Ecosystems from Pollution, 191-205.
- Birkhauser Verlag, Switzerl, Sriyutha Murthy P, Venkatesan R, Nair KVK, Ravindran M (2004) Biofilm control for plate heat exchangers using surface seawater from the open ocean for the OTEC power plant. International Biodeterioration & Biodegradation 53(1): 133-140.
- Lee Y, Kim H, Lee U (2004) Formation of chlorite and chlorate from chlorine dioxide with Han River Water. Korean Journal of Chemical Engineering 21(3): 647-653.
- Standard Methods for the Examination of Water and Wastewater, APHA, AWWA and WEF, Washington, D.C. (20th, 2014).
- Nebot E, Casanueva JF, Casanueva T, Sales D (2007) Model for fouling deposition on power plant steam condensers cooled with seawater: effect of water velocity and tube material. International Journal of Heat and Mass Transfer 50: 3351-3358.
- Lijesen MG (2007) The real-time price elasticity of electricity. Energy Economics 29(2): 249-258.
- Venkatesan R, Murthy PS (2009) Macrofouling control in power plants. Marine and Industrial Biofouling 4(II): 265-291.
- Tai C, Jiang G (2005) Dechlorination and destruction of 2,4,6 trichlorophenol and pentachlorophenol using hydrogen peroxide as the oxidant catalyzed molybdate ions under basic condition. Chemosphere 59(3): 321-327.
- Yebra DM, Kiil S, Weinell CE, Dam-Johnson K (2006) Effects of marine microbial biofilms on the biocide release rate from antifouling paints- a model-based analysis. Progress in Organic Coatings 57(1): 56-66.
- Zhou J, Xue G, Zhao HB, Wang YH, Guo MF (2004) Kinetics of chlorine decay in water distribution systems, Journal of Donghua University 21(1): 140-145.
- Sriyutha Murthy P, Venkatesan R, Nair KVK, Ravindran M (2004) Biofilm control for plate heat exchangers using surface seawater from the open ocean for the OTEC power plant. International Biodeterioration & Biodegradation 53(1); 133-140.
-
Mohamed Safwat Mohamed Tawfik*. Evaluation of the Marine Impacts Resulting from the Using of Different Chemical Page 2 of 9 Biocides in Cooling Water System of New Nuclear Power Plant. Ad Oceanogr & Marine Biol. 3(3): 2023. AOMB.MS.ID.000564.
-
Azolla, Spirulina platensis, GIFT tilapia, Digestive enzymes, Immunity, Carotenoid content, Isocaloric, Anisonitrogenous, Spirulina platensis, Dissolved Oxygen
-
This work is licensed under a Creative Commons Attribution-NonCommercial 4.0 International License.