Mini Review Article
The Role of Mitochondrial Dysfunction in the Mechanism of Neurodegenerative Diseases
Esra Çelen1,2* and Hasibe Cingilli Vural2
1Faculty of Agriculture and Natural Sciences, Konya Food and Agriculture University, Turkey
2Department of Medical Biology, Meram Faculty of Medicine, Necmettin Erbakan University, Turkey
Esra Çelen, Faculty of Agriculture and Natural Sciences, Konya Food and Agriculture University, Turkey.
Received Date: October 15, 2018; Published Date: November 09, 2018
Abstract
The increase in the incidence of neurodegenerative diseases negatively affects the life quality of the individuals and is also a great burden to health sector. Neurodegenerative diseases, which are the most common forms of dementia, are Alzheimer’s disease, Parkinson’s disease, Huntington’s disease, Amyotrophic Lateral Sclerosis and Multiple Sclerosis. They are progressive diseases showing premature death of neurons and can be triggered by genetic and environmental factors. The nature of age-dependence of these diseases supports the view that the mechanisms of these diseases are linked to mitochondrial mutations. As the proportion of mutant mitochondria in the tissue increases, ATP production becomes inefficient and dysfunction is observed. Some mitochondrial functions are affected in neurodegenerative diseases. Some of them include decreased glucose metabolism, mitochondrial enzymatic damage and increased production of ROS. Changes in mitochondrial dynamics are mitochondrial fission and fusion disruption, reduction of mitochondria transport on axons, decrease in intracellular mitochondria rate, and deformation in shape. There is still no effective treatment option for neurodegenerative diseases. In this review, the known mechanisms of neurodegenerative diseases and mitochondrial bases will be discussed, and the roles of mitochondrial dysfunction in these diseases will be reviewed.
Keywords: Dementia; Mitochondria; Neurodegenerative disease; ROS; Aging
Introduction
The great number of people are diagnosed with age-related diseases with the increasing average age worldwide. Especially neurodegenerative diseases are great burden for the health sector and reduce the quality of life of patients. How age-related diseases appeared with aging is not clarified yet, however there are some mechanisms suggested. Neurodegenerative diseases cause dementia and cognitive disorders. One of the most acceptable mechanisms for the pathogenesis of neurodegenerative diseases is mitochondrial dysfunction [1].
The brain is thought to be the most vulnerable organ to the effects of aging. The brain, which is only 2% of the body weight, consumes about 20% of the energy required for the body. Because of its limited glycolytic capacity and its metabolically active structure, neurons have high energy demand. In order to compensate these needs, they also require significant amounts of mitochondrial function. Proper placement of mitochondria between these structures, accurate distribution and transport are very important for mitochondrial function [2].
Mitochondria
Mitochondria are called powerhouses of cell because they provide energy. They are double-membraned organelles with their genetic material in all eukaryotic organisms, ranging from 0.75 to 3 μm in diameter. Cells can vary in size and structure depending on their needs. They can multiply by energy demand, independent of cell division. The mitochondrion is composed of 4 different parts, each with different contents and functions (Figure 1). There is an intermembrane space between the inner and outer membrane where specialized proteins are placed. Inside the matrix surrounded by the inner membrane, there are peptidases, chaperone proteins enzymes, mitochondrial genome and enzymes necessary for metabolic pathways such as citric acid cycle, fatty acid oxidation and urea cycle [3]. Some events that occur in mitochondria can be listed as ATP synthesis, synthesis of certain metabolites, production of endogenous reactive oxygen species (ROS), Ca2+ homeostasis, programmed and unprogrammed cell death.
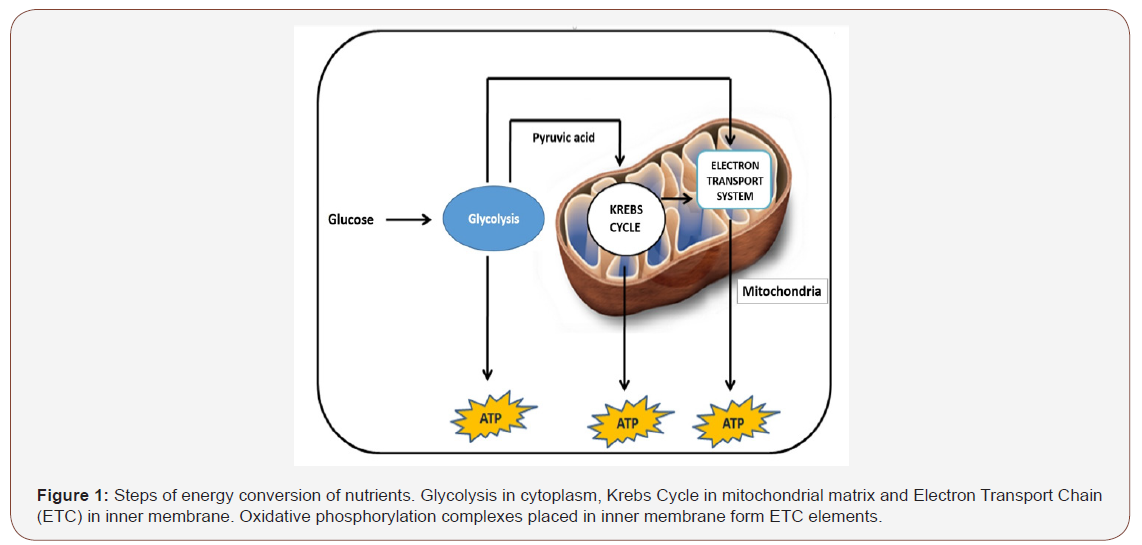
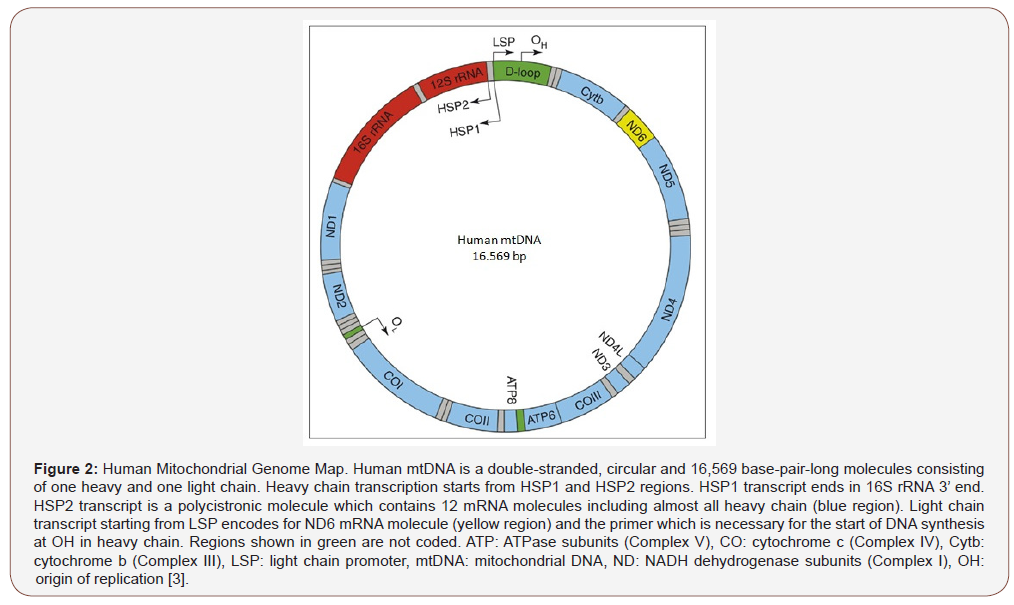
The great majority of 1400 proteins functioned in mitochondria is encoded by nuclear genome and then transported into this organelle [4]. Replication and transcription of mtDNA occurs independently of the nuclear genome. Human mtDNA is a doublestranded, circular molecule spanning 16.569 base pairs and containing 5523 codons (Figure 2) [5]. Thirteen of the thirty-seven genes code for proteins that make up the respiratory chain. These proteins are the subunits of complex I, complex III, complex IV and complex V in OXPHOS complexes. Complex II is encoded by nuclear genome.
Molecular events that occur in mitochondria cause electrochemical potential formation between membranes. This is necessary for the synthesis of adenosine triphosphate (ATP), which is the main energy source of cells necessary for many cellular events [6]. ETC is a chain system consisting mainly of 4 proteinmetal complexes (I-IV). ATP production is provided by the flow of electrons over this chain (Figure 3). Nicotinamide adenine dinucleotide (NADH) molecules are the first molecules to enter ETC in mitochondria. Another integral protein necessary for oxidative phosphorylation is ATP synthase or complex V. Electron flow through the ETC ultimately ends with ATP production in complex V. This process, starting with the NADH molecule and ending with ATP production, is called oxidative phosphorylation (OXPHOS) [7].
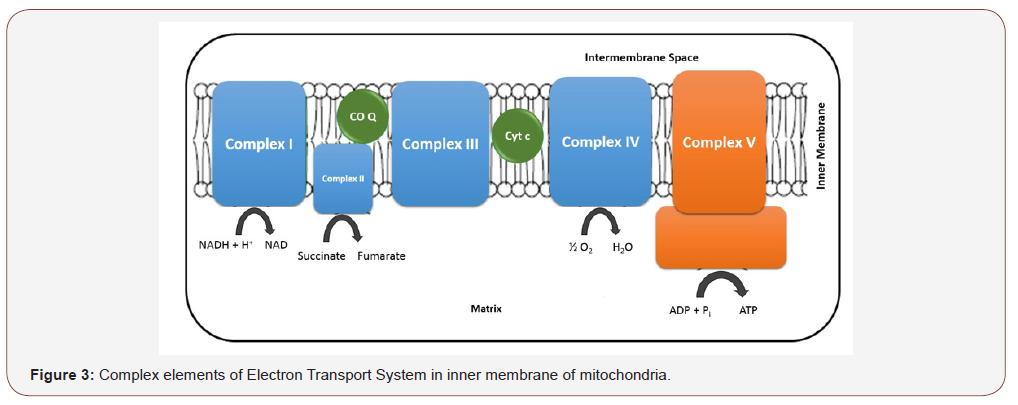
Mitochondrial Dynamics
Neurons are polarized cells with their dendritic and axonal extensions. The proper distribution and transport of mitochondria on these structures are very important. Thanks to these events, mitochondria, which provide continuous fission and fusion events to maintain integrity and quantity, are responsible for cellular events such as ATP production, Ca2+ homeostasis, reactive oxygen species (ROS) production and cell death. Fission and fusion events are regulated by large dynamin-related GTPase proteins. The mechanism responsible for the initiation of the fission event is still unclear. The principal regulatory molecule is Drp1 (dynaminrelated protein 1), which is localized in the cytoplasm. During the fission event, cytosolic Drp1 is recruited into the mitochondrial outer membrane by receptor proteins such as Mff, Fis1 and MiD 48/51. A ring-like structure is formed as a result of oligomerization [1]. Dyn2, a dynamin -like protein, has also been shown to be involved in the final phase of membrane fusion after Drp1 has been recruited and polymerized [8].
Mitochondrial fission
Due to the high-yield ROS production and the loss of DNA repair mechanisms, mitochondria are vulnerable to major damages. For damaged mitochondria, quality control mechanisms in cells have developed. Through these mechanisms, damage is tried to be repaired and normal function is acquired. A mitochondrion which is irreversibly damaged must be eliminated. The fission event has been shown to play a role in the removal of damaged mitochondria by autophagy [9]. PINK1 and Parkin proteins, which are mutants in familial Parkinson’s disease, play a role in the canonical pathway of this phenomenon, also called mitophagy [10].
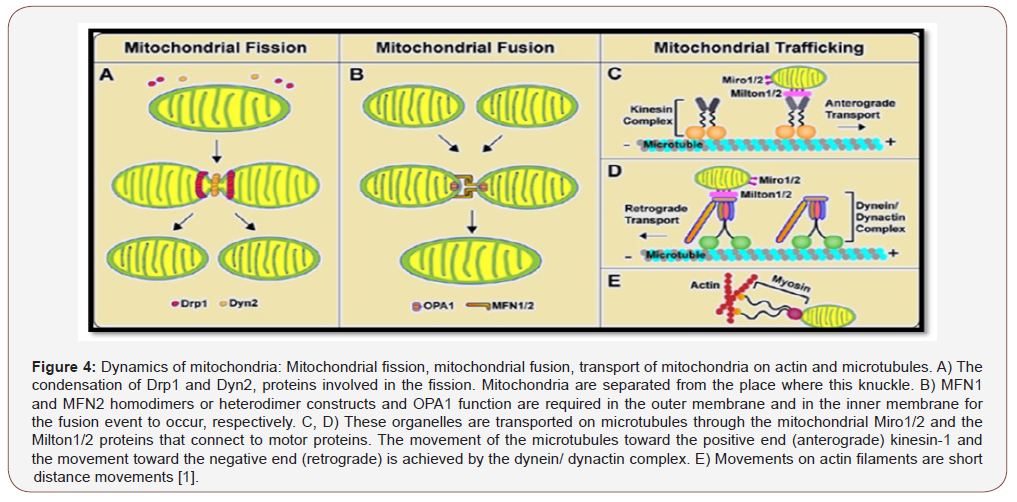
Mitochondrial fusion
Mitochondrial fusion occurs with fusion of both outer and inner membranes (Figure 4). The fusion of the outer membrane is regulated by Mfn1 (Mitofusin 1) and Mfn2 (Mitofusin 2), while the fusion of the inner membrane is regulated by OPA1 (Optic atrophy protein 1). Homo-oligomeric or hetero-oligomeric complexes of Mfn1 and Mfn2 proteins allow the outer membranes to stay together [11,12].
Mitochondrial movement
In physiological and pathological situations, mitochondria act on regions which require energy. Localizations within the cell are regulated by two-sided movements on microtubules (rapid motion) and actin filaments (slow motion) [12]. The motor protein kinesin-1, which provides anterograde movements of mitochondria on microtubules, and the motor dynein/ dynactin complex, which provides retrograde movements, is in interaction with Milton and Miro (Figure 5) [13,14].
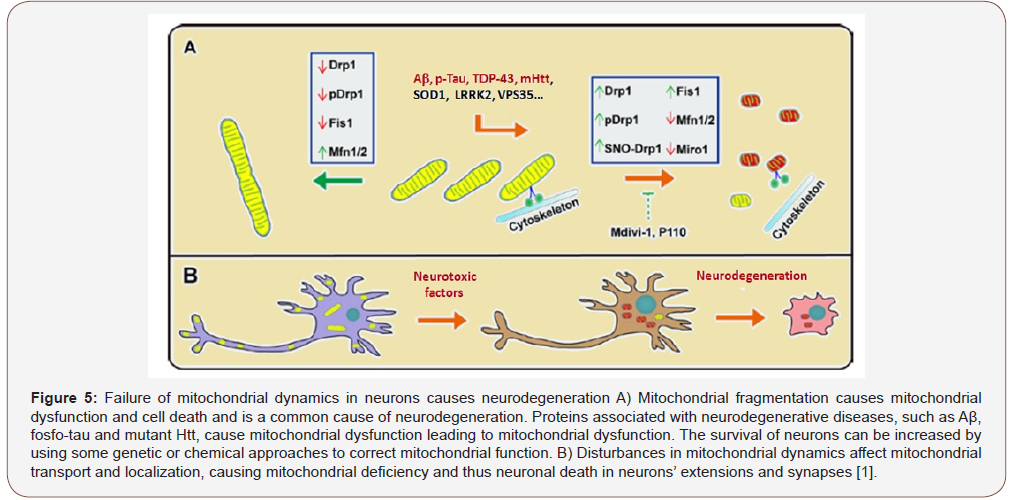
Mitochondrial Dysfunction in Neurodegeneration
The studies in literature show that mitochondrial dysfunctions have devastating effects on neuronal function and structure and are seen in all major neurodegenerative diseases [1]. Because mitochondria are closely related to cellular mechanisms, mitochondrial dysfunctions can be said to be at the center of the pathogenesis of neurodegenerative diseases (Figure 5).
The inaccurate fusion of mitochondria caused point mutations and deletions in mtDNA and resulted in the accumulation of nonfunctional mitochondria. Fusion of different mitochondrial contents and lipid membranes is of great importance for mtDNA repair. Furthermore, in order for healthy cell populations of mitochondria to form, the mitochondrial metabolites must be distributed equally [15,16].
Oxidative stress
Free radicals are formed both during physiological and pathological events. In physiological concentrations, ROS play important roles in signal transduction pathways such as cell cycle regulation, phagocytosis, and enzyme activation. On the other hand they may cause DNA, lipid and protein damage at high concentrations. Oxidant-antioxidant balance must be established to prevent cellular damage. Oxidative damage that occurs when the balance can not be established is particularly effective in diseases such as neurodegenerative diseases, cancer, diabetes and aging [17].
In normal physiological conditions, ROS are involved in events such as immune response, inflammation, learning, memory. However, when they are overproduced, these molecules disrupt the structures of proteins and DNA as a result of oxidative stress. ROS production occurs during mitochondrial OXPHOS. Some of the ways in which the oxidative stress is mediated by the cell are superoxide dismutase (SOD) and glutathione systems. There has to be a balance between free radical production and the detoxification events that occur through these systems. If this balance is disturbed, the ROS production is heavily stressed causing cellular functions to deteriorate. This leads to confusion as mitochondrial dysfunction which is known to cause many pathologies [2].
For ATP production, mitochondria require about 85% oxygen (O2). The electrochemical potential in the inner membrane of the mitochondria that controls ATP production is important in the molecular level that changes mitochondrial movement in response to mitochondrial dysfunction. In particular, complexes I and III form ROS, including oxygen radicals and hydrogen peroxide, which can damage cells, nucleic acids and proteins. This structure causes diseases related to mitochondrial dysfunction including neurodegeneration. As a result of electron transport chain activity, free radicals are formed in the form of O2 as by-products. mtDNA, which is located close to the ROS production region, does not already have a repair mechanism that of nuclear DNA and protective histone proteins. mtDNA is thus exposed to oxidative damage and has higher mutation rate. Another effect of oxidative stress is triggering neuronal cell death leading to the pathogenesis of many neurodegenerative diseases.
Neurons are cells that need high energy. Glucose oxidation is the most common energy source in the brain. Oxidations of sources like fatty acids provide low ATP production rate. In order to meet the energy, need in brain, oxidative stress is produced. As a result, neurons meet their energy needs in the form of ATP from the mitochondrial OXPHOS system [2]. Along with aging, neurons are damaged as a result of mitochondrial dysfunction and neurodegenerative diseases such as Alzheimer’s disease (AD), Parkinson’s disease, Huntington’s disease, Amyotrophic lateral sclerosis (ALS) and Multiple sclerosis (MS) begin to appear.
Mitochondrial Dysfunction in Neurodegenerative Diseases
Alzheimer’s disease
Alzheimer’s Disease (AD), the most common neurodegenerative disease, was first reported by a German doctor, Alois Alzheimer. His patient was thought to be mentally ill. After her death, doctor configured that there was plaque formation in her brain [18]. According to the areas affected in brain, AD patients show different symptoms over time, usually beginning with memory loss. Long-term memory loss also occurs in patients who start to see hallucinations following the disturbances in logical thinking and emotions. It may cause death by affecting respiration and heart in advanced stages. While there is no definitive treatment, existing treatments are slowing the process down. AD is a disease with multifactorial etiology in which both genetic and environmental factors are risk factors [19].
In the advanced stage of AD, Aβ plaques and tau neurofibrillary tangles are formed. These structures are thought to weaken the communication between neurons and cause the cells to lose their nutrition and die. Similarly, mitochondrial dysfunction is thought to be the cause of disease due to its apperance at early stage of AD. So there is possibility for the diagnosis and treatment of the disease [7].
Aβ amyloid precursor protein (APP) is a protein that is formed by proteolysis by α-secretase, β-secretase or -secretase enzymes. The α-secretase enzyme cleaves APP from its transmembrane region at a distance of 12 amino acids, forming the α-APPs fragment. The β-secretase enzyme cleaves at a region which is 16 amino acids closer to the amino terminus of APP to form a β-APPs fragment. After these enzymes, a second cleavage is made by -secretase. -secretase enzyme cleavage forms p3 fragment and Aβ peptide after α-secretase cleavage and β-secretase enzyme cleavage, respectively. The enzyme that determines the length of the Aβ peptide is also -secretase enzyme. This physiological process may be more active in AD cases. Another possibility for AD, is the formation of precipitates due to the situations such as faults in fragmentation of Aβ peptide and misfolding [20].
Tau is a microtubule-associated protein that is expressed predominantly in neurons and is usually localized in axons. Tau is responsible for the regulation of the microtubules through the microtubule-binding region and the phosphorylation regulates microtubule-binding capacity and stabilization negatively. Tau fibrillary tangles are characteristics of certain neurodegenerative diseases, including AD. They are commonly referred to as tautopathies. The affinities of hyperphosphorylated tau proteins to microtubules are reduced and form intracellular precipitates. The etiology of tau hyperphosphorylation and the conditions are still unclear and are usually studied on experimental animal models [21,22].
The high metabolic demand of neurons, restricted regeneration capacity of brain and low antioxidant level make the brain more prone to oxidative stress. The increase in mitochondrial dysfunction and apoptosis, which are thought to be effective in the AD mechanism, also accompanied by a low level of antioxidant [17]. Studies have shown that oxidative stress, induced by superoxide anion, hydroxyl radical, hydrogen peroxide and nitric oxide, cause neurodegeneration in AD [23,24].
Parkinson disease
What James Parkinson discovered is a progressive neurodegenerative disease that affects movements. It is known that one million people in America have Parkinson Disease (PD). Existing treatment options aim to improve symptoms. The known mechanism of the disease is the dopamine reduction due to death of dopaminergic (DA) neurons. As a result, involuntary movements are encountered in patients. It is known to be affected by genetic and environmental factors (1, 2). Mutations in the familial form, particularly the autosomal recessive PD-related proteins PINK1, Parkin and DJ-1, have been shown to be effective in modulating mitochondrial dynamics and morphology by modulation of Drp1 expression and activity. Pathogenic mutations or suppression of Parkin cause an increase in Drp1 level and an abnormal mitochondrial fission event [25].
In DA neurons, Lewy bodies, which are known to be composed of α-synuclein structures, are encountered. Oxidative stress has been shown to cause the formation of oxidized α-synuclein and that these form of α-synuclein are more precipitated than normal α-synuclein forms. Similarly, there are publications showing that the α-synuclein increase is also caused by oxidative stress [1,2].
Amyotrophic lateral sclerosis
Also known as Lou Gehrig’s disease, Amyotrophic Lateral Sclerosis (ALS) disease is a neurodegenerative disease that results in progressive degeneration of the brain stem and motor neurons in the vertebrate. Muscle weakness, difficulty in speaking, swallowing and breathing are certain symptoms of ALS. Just like in PD, inclusion bodies are encountered. These inclusion bodies are composed of TDP-43 molecules. Although the mechanism of the disease has not yet been fully elucidated, TDP-43 is the basic compound discovered. There are abnormalities in mitochondrial morphology in sporadic and familial forms. Mitochondrial fragmentation was found in some cell and animal models [1].
The first discovered mutation in ALS is the mutation of the SOD1 enzyme. In the SOD1 mutant experimental models, changes in the expression of the fission / fusion regulators Drp1, OPA1, Mfn1 and Fis1 have been reported. The deletion-type mutation in TDP-43, the molecule that forms the inclusion bodies, causes hereditary ALS. Less than 10% of ALS is in the familial form and less than 4% of the TDP-43 mutant forms of ALS in the familial form. Mutations in mitochondrial fragmentation and change in fission/ fusion regulators were found in TDP-43 mutant forms [1,2].
Discussion
The mechanisms of neurodegenerative diseases have not been fully elucidated. It is known that they affect different regions of the brain and cause progressive changes. With the gradual discovery of mechanisms, the roles of mitochondrial dysfunction in these diseases have begun to be uncovered. It is seen in the literature that mitochondrial dynamics are tried to be corrected and that antioxidant applications are widely used to eliminate the effects of oxidative stress. There is still no effective treatment for neurodegenerative diseases. In this context, it is very important to support the studies in the field of neurology with molecular studies. The reduction of question marks is expected with the discovery of the molecular substructures and mitochondrial dynamics of these diseases, which have complex mechanisms. Genetic counseling should also be available for neurodegenerative diseases which are known to be genetic or suspected to be hereditary. Just as in other hereditary diseases, genetic counseling services may provide early diagnosis for patients and also their relatives and may give advice on treatment options for these diseases. The elucidation of the mechanisms of multifactorial neurodegenerative diseases is of crucial importance in the discovery of the relationship with mitochondrial dysfunction in order to prevent neurodegeneration, to improve neuronal function and to offer new treatment options.
Conclusion
The increase in the incidence of neurodegenerative diseases negatively affects the life quality of the individuals. Neurodegenerative diseases are progressive age-dependent diseases. Mitochondrial dysfunction is one of the proposed mechanisms of neurodegenerative diseases. Some functions which are showed to be disrupted are glucose metabolism, ROS production and mitochondrial dynamics. The elucidation of the relationship between mitochondrial dysfunction and neuronal dysfunction may give opportunity to improve potential treatments.
Acknowledgemnet
None.
Conflict of Interest
No conflict of interest.
References
- Asin-Cayuela J, Gustafsson CM (2007) Mitochondrial transcription and its regulation in mammalian cells. Trends Biochem Sci 32(3): 111-117.
- Cadonic C, Sabbir MG, Albensi BC (2016) Mechanisms of mitochondrial dysfunction in Alzheimer’s disease. Mol Neurobiol 53(9): 6078-6090.
- Cha MY, Kim DK, Mook-Jung I (2015) The role of mitochondrial DNA mutation on neurodegenerative diseases. Exp Mol Med 47(3): e150.
- Chen H, McCaffery JM, Chan DC (2007) Mitochondrial fusion protects against neurodegeneration in the cerebellum. Cell 130(3): 548-562.
- Cheng X, Kanki T, Fukuoh A, Ohgaki K, Takeya R, et al. (2005) PDIP38 associates with proteins constituting the mitochondrial DNA nucleoid. J Biochem 138(6): 673-678.
- Espinoza M, De Silva R, Dickson DW, Davies P (2008) Differential incorporation of tau isoforms in Alzheimer’s disease. J Alzheimer’s Dis 14(1): 1-16.
- Gao J, Wang L, Liu J, Xie F, Su B, et al. (2017) Abnormalities of Mitochondrial Dynamics in Neurodegenerative Diseases. Antioxidants 6(2): 25.
- Glater EE, Megeath LJ, Stowers RS, Schwarz TL (2006) Axonal transport of mitochondria requires milton to recruit kinesin heavy chain and is light chain independent. J Cell Bio 173(4): 545-557.
- Petry FR, Pelletier J, Bretteville A, Morin F, Calon F, et al. (2014) Specificity of anti-tau antibodies when analyzing mice models of Alzheimer’s disease: problems and solutions. PloS One 9(5): e94251.
- Picard M, Wallace DC, Burelle Y (2016) The rise of mitochondria in medicine. Mitochondrion 30: 105-116.
- Grimm A, Eckert A (2017) Brain aging and neurodegeneration: from a mitochondrial point of view. J Neurochem 143(4): 418-431.
- Guo X, Macleod GT, Wellington A, Hu, Panchumarthi S, et al. (2005) The GTPase dMiro is required for axonal transport of mitochondria to Drosophila synapses. Neuron 47(3): 379-393.
- Ishihara N, Eura Y, Mihara K (2004) Mitofusin 1 and 2 play distinct roles in mitochondrial fusion reactions via GTPase activity. J Cell Sci 117(26): 6535-6546.
- Khacho M, Slack RS (2015) Mitochondrial dynamics in neurodegeneration: from cell death to energetic states. AIMS Mol Sci 2(2): 161-174.
- Lee JE, Westrate LM, Wu H, Page C, Voeltz GK (2016) Multiple dynamin family members collaborate to drive mitochondrial division. Nature 540(7631): 139-143.
- Manoharan S, Guillemin GJ, Abiramasundari RS, Essa MM, Akbar M, et al. (2016) The Role of Reactive Oxygen Species in the Pathogenesis of Alzheimer’s Disease, Parkinson’s Disease, and Huntington’s Disease: A Mini Review. Oxid Med Cell Longev 2016: 8590578.
- Tarawneh R, Holtzman DM (2012) The Clinical Problem of Symptomatic Alzheimer Disease and Mild Cognitive Impairment. Cold Spring Harb Perspect Med 2(5): a006148.
- Roberts RF, Fon EA (2016) Presenting mitochondrial antigens: PINK1, Parkin and MDVs steal the show. Cell Res 26(11): 1180-1181.
- Saka E (2010) Pathophysiology of Alzheimer’s Disease: Experimental and Genetic Findings. Turk J Geriatr 3: 21-26.
- Santel A, Fuller MT (2001) Control of mitochondrial morphology by a human mitofusin. J Cell Sci 114(5): 867-874.
- Sas K, Robotka H, Toldi J, Vécsei L (2007) Mitochondria, metabolic disturbances, oxidative stress and the kynurenine system, with focus on neurodegenerative disorders. J Neurol Sci 257(1-2): 221-239.
- Smith MA (1998) Alzheimer disease. Int Rev Neurobiol 42: 1-54.
- Twig G, Elorza A, Molina AJ, Mohamed H, Wikstrom JD, et al. (2008) Fission and selective fusion govern mitochondrial segregation and elimination by autophagy. The EMBO Journal 27(2): 433-446.
- Van Dyke K (1997) The possible role of peroxynitrite in Alzheimer’s disease: a simple hypothesis that could be tested more thoroughly. Med hypotheses 48(5): 375-380.
- Xie Z, Wei M, Morgan TE, Fabrizio P, Han D, et al. (2002) Peroxynitrite mediates neurotoxicity of amyloid β-peptide1–42-and lipopolysaccharide-activated microglia. J Neurosci 22(9): 3484-3492.
-
Esra Çelen, Hasibe Cingilli Vural. The Role of Mitochondrial Dysfunction in the Mechanism of Neurodegenerative Diseases. Arch Neurol & Neurosci. 1(4): 2018. ANN.MS.ID.000520.
-
"Mitochondrial, Dysfunction, Neurodegenerative diseases, Dementia, Alzheimer's disease, Parkinson's disease, Huntington's disease, Amyotrophic Lateral Sclerosis, Multiple Sclerosis, Genetic, Neurons, Mitochondrial mutations, Glucose metabolism, Axons, ROS, Aging, Cognitive disorders, Glycolytic capacity, Brain
-
This work is licensed under a Creative Commons Attribution-NonCommercial 4.0 International License.