Case Report
Intercellular and Intracellular Networks: Signal Processing and Evolution
Andrew V Kuznetsov*
A.O. Kovalevsky Institute of Biology of the Southern Seas, Russia
Andrew V Kuznetsov, A.O. Kovalevsky Institute of Biology of the Southern Seas, RAS, Leninsky Avenue 38, Moscow, 119991, Russia.
Received Date: August 30, 2023; Published Date: October 18, 2023
Abstract
The coordination of a group of specialized cells carrying out specific tasks in multicellular animals results in the development of the nervous system. Studies on the Ctenophora, Placozoa, and Ciliophora demonstrate that networks of protein-protein interactions with a similar level of complexity to brain networks carry out signal processing in individual cells.
Keywords:Protein-protein interactions; Neuronal networks; Ciliophora; Placozoa; Ctenophora
Introduction
It is supposed that the nervous system emerged in primitive multicellular organisms as a result of coordinating the activity of specialized cells performing different functions through chemical communication by means of neurotransmitters [1]. Thus, specific genes of the nervous system encoding synaptic proteins, voltagegated sodium, potassium, and calcium channels, etc. were identified in the genome of primitive Placozoa [2], but no neurons were observed while complex behavior of the organism. Orthologs of neurospecific proteins of higher animals like Glial Fibrillary Acidic Protein (GFAP) are identified the genome of Ctenophora [3], there is a diffuse network of neurons that covers the whole body of the animal and innervates the aboral organ that acts as a multiple sensor [4]. An elementary ability to make decisions in situational conditions has been proven in Mnemiopsis leidyi [5]. In addition, Trichoplax adhaerens was found to have a gene for the precursor of the major histocompatibility complex (proto-MHC), which is essential for the functioning of the vertebrate immune system [6]. Intensive alternative splicing, great variability, and the number of adhesion receptors indicate the common origin of the immune and nervous systems [7], which are evolved to solve pattern recognition tasks. It is unexpected that computational tasks inherent to neural networks can be solved not only by multicellular animals with highly developed nervous systems but also by single-cell organisms up to bacteria, which has been noted by both theorists [8] and experimentalists [9]. Indeed, a simple robot or an autonomous agent must be able to receive signals from the environment, process them, and perform responses with the help of actuators in order to survive in unfavorable conditions. Unicellular organisms show amazing examples of adaptation and patterns of behavior. Examples include chemical communication and quorum sensing, which provide preventive regulation in bacterial populations [10]. What mechanisms are responsible for the complex behavior observed in primitive organisms?
Protein-Protein Interactions Resemble Neural Networks
It has been shown that not only animals but also plants are capable of signal processing and decision-making [11], and “bio computations” occur due to the formation of a network of metabolic pathways and protein-protein interactions in response to incoming stimuli [12]. Given steadily repeating patterns of signals, the metabolic neural network is able to change its structure to adapt to the input signals [13]. This process resembles the training of a computer neural network with the the establishment weights. It can be manifested at the physiological grade and fixed at the genetic level in the course of long evolution as a result of the selection of correct solutions and corresponding computational structures.
Bioinformatics research has revealed protein-protein interaction networks that resemble a perceptron in evolutionarily ancient organisms, such as Trichoplax adhaerens, Trichoplax sp. H2 [14] and Mnemiopsis leidyi [15,16]. It turned out that signals from visual opsin receptors or mechanosensitive ion channels (Transient Receptor Potential channels and Piezo channels) can propagate rapidly across the cell membrane, penetrate with a delay into the cytoplasm or be slowly delivered to the nucleus, which provides a wide range of responses of the biological system from physiological to genetic levels.
Sessile infusoria are the most intriguing unicellular organisms because they can adapt to repeated stimuli, which is reminiscent of learning [17]. The genomes of 62 representatives of Ciliophora have been sequenced, some of which have been annotated, allowing us to study protein-protein interaction networks. We constructed a spatial model of rhodopsin-like GPCR transmembrane protein XP_001030210_2, which has 7 typical α-helices (Figure 1a), as well as a nearest neighbor tree (Figure 1b) and a 10-order interactive graph (Figure 1c) for Tetrahymena thermophila who is a free-living protozoon and occurs in fresh water. Similarly, the unannotated XP_001456900_1 protein, also including 7 transmembrane α-helices (Figure 2a), which reproduces the structure of the canonical opsin, was reconstructed in the slipper animalcule Paramecium caudatum. After that, first- and 10th-order protein neighbor graphs were constructed (Figure 2b and 2c). It should be noted that in addition to the channel formed by transmembrane α-helices, both XP_001030210_2 and XP_001456900_1 have an interactive domain at the N-terminus, which distinguishes them from vertebrate opsins. A well-developed network of protein-protein interactions with a nodal opsin-like protein may be involved in the phototaxis of infusoria (Figure 1 and Figure 2).
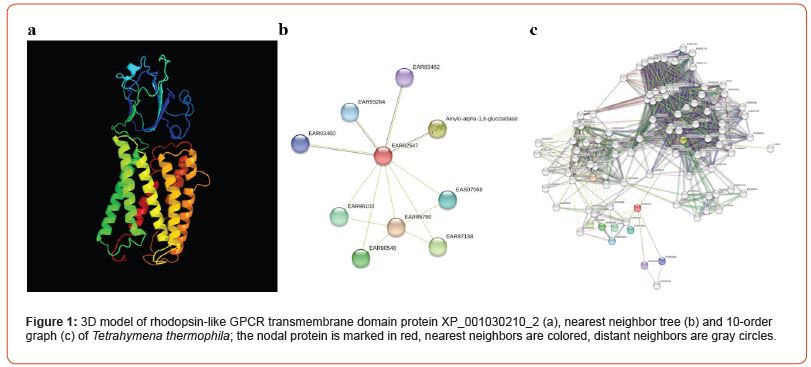
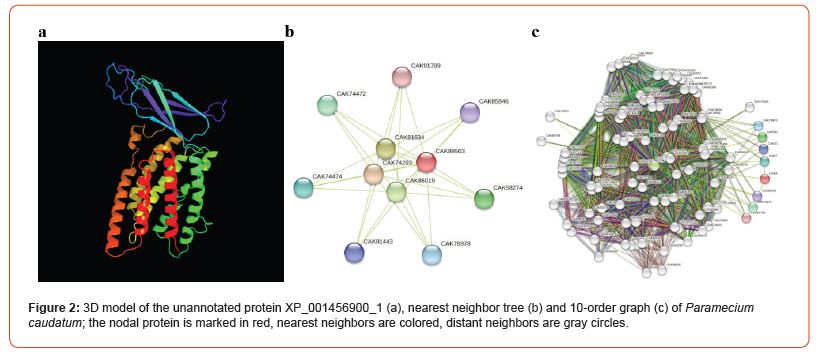
In addition, mechanosensory receptors, presumably Piezo proteins, were found in Tetrahymena thermophila, which interact with proteins involved in the transport of vesicles from the endoplasmic reticulum to the Golgi complex. The existence of complicated molecular mechanisms in ciliates allows these organisms to survive in complex and changing environmental conditions throughout their evolutionary history.
Conclusion
The protein-protein interaction networks discovered in Ctenophora, Placozoa, and Ciliophora are characterized by great complexity and resemble neuronal networks processing information in animals with developed immune and nervous systems that allows us to discuss the underestimation of the “cognitive” abilities of a single cell. The development of networks of metabolic pathways and protein-protein interactions is evident when studying free-living unicellular eukaryotes.
Acknowledgement
The author is grateful to his students EV Sergeeva, MV Fadeeva, and UD Roschina for their help and fruitful discussions in the laboratory.
Conflict of Interest
No conflict of interest.
References
- Leonid L Moroz, Daria Y Romanova, Andrea B Kohn (2021) Neural versus alternative integrative systems: molecular insights into origins of neurotransmitters. Philos Trans R Soc Lond B Biol Sci 376(1821): 20190762.
- Mansi Srivastava, Emina Begovic, Jarrod Chapman, Nicholas H Putnam, Uffe Hellsten, et al. (2008) The Trichoplax genome and the nature of placozoans. Nature 454(7207): 955-960.
- Joseph F Ryan, Kevin Pang, Christine E Schnitzler, Anh Dao Nguyen, R Travis Moreland, et al. (2013) The genome of the ctenophore Mnemiopsis leidyi and its implications for cell type evolution. Science 342(6164): 1242592.
- Aronova MZ, Titova LK, Kharkeevitch TA, Tsirulis TP (1979) Ultrastructural peculiarities of the chemical transmission of the nerve impulse in the gravity receptors of some invertebrates. Z Mikrosk Anat Forsch 93(1): 91-103.
- Silakov MI, Kuznetsov AV, Temnykh AV, Anninsky BE (2023) Effect of monochromatic light on the behavior of the ctenophore Mnemiopsis leidyi (A. Agassiz, 1865). Biosystems 231: 104987.
- Jaanus Suurväli , Luc Jouneau , Dominique Thépot , Simona Grusea , Pierre Pontarotti, et al. (2014) The proto-MHC of placozoans, a region specialized in cellular stress and ubiquitination/proteasome pathways. J Immunol 193(6): 2891-2901.
- Dimitris Kioussis, Vassilis Pachnis (2009) Immune and nervous systems: more than just a superficial similarity? Immunity 31(5): 705-710.
- Fulda FC (2017) Natural agency: the case of bacterial cognition. J Am Philos Assoc 3: 69-90.
- Pamela Lyon (2015) The cognitive cell: bacterial behavior reconsidered. Front Microbiol 6: 264.
- Popat R, Cornforth DM, McNally L, Brown SP (2015) Collective sensing and collective responses in quorum-sensing bacteria. J R Soc Interface 12(103): 20140882.
- Ben Scheres, Wim H van der Putten (2017) The plant perceptron connects the environment to development. Nature 543(7645): 337-345.
- Amir Pandi, Mathilde Koch, Peter L Voyvodic, Paul Soudier, Jerome Bonnet, et al. (2019) Metabolic perceptrons for neural computing in biological systems. Nat Commun 10(1): 3880.
- Youri Timsit, Sergeant Perthuis Grégoire (2021) Towards the Idea of Molecular Brains. Int J Mol Sci 22(21): 11868.
- Kuznetsov AV, Grishin IYu, Vtyurina DN (2023) Spatial Models of Piezo Proteins and Protein‒Protein Interaction Networks in Trichoplax Animals (Placozoa). Molecular Biology (Mosk) 57(5): 895-897.
- Sergeeva EV, Fadeeva MV, Khavronyuk IS, Mamontov AA, Ershov AB, Kuznetsov AV (2022) Opsins of the ctenophore Mnemiopsis leidyi and a network of protein-protein interactions. Russian Journal of Biological Physics and Chemistry 7(2): 222-229.
- Kuznetsov AV, Vtyurina DN (2023) Spatial Reconstruction of TRPC Mechanoreceptors of the Ctenophore Mnemiopsis leidyi A. Agassiz, 1865. Molecular Biology (Mosk) 57(4):726-735.
- Dexter JP, Prabakaran S, Gunawardena J (2019) A complex hierarchy of avoidance behaviors in a single-cell eukaryote. Curr Biol 29(24): 4323-4329.e2.
-
Andrew V Kuznetsov*. Intercellular and Intracellular Networks: Signal Processing and Evolution. Arch Neurol & Neurosci. 15(5): 2023. ANN.MS.ID.000875.
-
Brain Disorders. Nerves, Psychology, Spinal Cord, Neuropathies, Anorexia Nervosa, vertigo, Hyperactivity Disorder, Seizure, Mental Health, Nervous System, Cortex, Dementia, Multiple , sclerosis, Cognitive neuroscience, Schizophrenia, Concussion, Alzheimer’s, Dementia, Depression, Motor , neurone Disease, Parkinson’s, Stroke, Addiction, Neurodegenerative Diseases, Consciousness, Dyslexia, Brain Disorders, Traumatic Stress Disorder, Migraine, Epilepsy, Hypertension, Anxiety, Sleep Disorders Autism, Aneurysm.
-
This work is licensed under a Creative Commons Attribution-NonCommercial 4.0 International License.