Short Communication
The Potential of Pyrogallol as a Possible Antimalarial Drug Candidate
Alfaqih, H. and Abu-Bakar, N*
School of Health Sciences, Health Campus, Universiti Sains Malaysia, 16150 Kubang Kerian, Kelantan, Malaysia.
Dr. Nurhidanatasha Abu Bakar, Biomedicine Program, School of Health Sciences, Universiti Sains Malaysia, 16150 Kubang Kerian, Kelantan, Malaysia
Received Date: August 18, 2020; Published Date: October 05, 2020
Abstract
Pyrogallol is a phenolic compound naturally found in oak, hardwood plants and many fruits such as apricot, avocado and gall. This compound possesses antibacterial, antipsoriatic and antifungal properties. However, the oxidative properties of pyrogallol have been encouraging numerous researches into the potential health hazards of its consumption. Nevertheless, the oxidising agent could be used for the treatment of some diseases such as malaria. According to previous studies, some antimalarial drugs such as primaquine, chloroquine and derivatives of artemisinin have the capability to kill the parasites through the generation of oxidative stress by the free radicals they generate. In this review, the potential of pyrogallol as an antimalarial drug candidate will be discussed, focusing on the biological activities as well as the toxicological effects it has on human health.
Keywords:Pyrogallol, antimalarial drugs, oxidizing agents.
Abbreviations: G6PD: Glucose-6-phosphate dehydrogenase; P. falciparum: Plasmodium falciparum; ACTs: Artemisinin-based combination therapy; O2: Oxygen; ONOO: Peroxide Nitrite; H2O2: Hydrogen peroxide; OH: Hydroxyradical; ROS: Reactive oxygen species; Cu2+: Cupric ion; Fe3+: Ferric iron or iron (III); Mn2+: Manganese; Co2: Carbon dioxide; Fe2: Ferrous or iron (II); NPs: Nanoparticles
Introduction
Malaria is an infectious disease caused by parasites of the genus Plasmodium. The infection is transmitted to humans via female mosquitoes of the genus Anopheles. The disease has long been identified as one of the leading causes of mortality and morbidity around the world [1]. This has led to the discovery of many antimalarial drugs over decades of years; however, drugrelated problems have been existed in parallel with these drugs. Plasmoquine, the first 8 aminoquinoline was introduced as an antimalarial drug in 1926 [2]. However, the consumption of this drug led to the occurrence of haemolytic toxicity in patients with glucose-6-phosphate dehydrogenase (G6PD) deficient [3]. Due to this problem, a new class of antimalarial drugs known as chloroquine was introduced in early 1940s [4]. This drug was safer, inexpensive, less toxic and highly efficacious as compared with plasmoquine [5]. However, the chloroquineresistant strains of P. falciparum emerged in Asia specially in Cambodia [6]. Consequently, a number of antimalarial drugs have been introduced as alternatives for chloroquine such as antibiotics, atovaquone and antifolates. Likewise, the resistance of malaria parasites had occurred to these drugs. As a result, the combination therapy has been utilised to improve the treatment of uncomplicated malaria [7]. For instance, artemisinin-based combination therapy (ACTs) has widely been used to fight against malaria and demonstrated positive impacts in malaria treatment as compared to other combination therapies; unfortunately, the emergence of ACTs resistance has recently been reported in Cambodia [8].
Due to the problem of drug resistance, numerous researches have been conducted on the field of herbal medicine to discover new effective antimalarial drugs [9]. Nowadays, a number of plant species have proved invaluable in the fight against malaria such as Quercus infectoria Olivier, Clerodendrum viscosum Vent, Duranta repens L, Nyctanthes arbor-tristis L, Lantana camara L, Cinnamosma fragrans H, Dracaena reflexa Lamk, Desmodium mauritianum DC, Ficus megapoda Bak and Andropogon schoenanthus L [10, 11]. Q. infectoria is a medicinal herb, which belongs to the family Fagaceae [12]. The parts of this plant that have been utilised for medicinal uses are particularly root, stem, seed, leaf, bark and gall [13]. Previous studies reported that Q. infectoria possesses antimalarial properties However, the exact phytochemical constituents of this plant leading to the treatment of malaria remain searchable. A study on Q. infectoria reported that pyrogallol was found among the phytochemicals of the plant [14, 15]. Pyrogallol has a capability of generating free radicals [16], which may cause the inhibition of parasite growth. However, the pyrogallol-related toxicity remains a large gap that needs further studies.
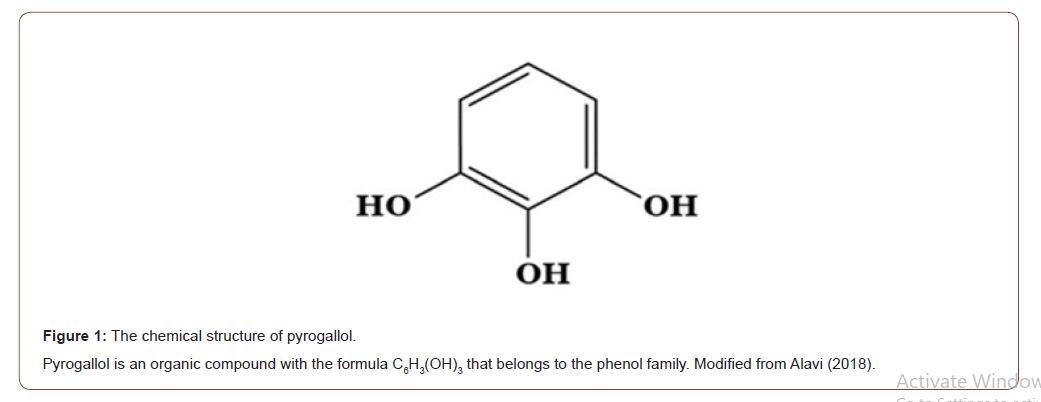
Pyrogallol also known as pyrogallic acid or 1,2,3-trihydroxybenzene, an organic phenol compound that exists naturally in many plants such as oak, eucalyptus, Terminalia chebula and Myriophyllum spicatum (Figure 1) [17-19]. People are naturally exposed to pyrogallol through several ways including consumption of tea, use of hair colouring creams, inhaling smoke produced during cooking mutton, fish and so on [17, 20]. Pyrogallol was found for the first time in the natural extract of the seeds of Abrus precatorius [21]. It is also found as a contaminant in anthocyanins, alkaloids, flavones and tannins and produced during its disposal, industrial use and isolation [17]. Resorcinol, its metabolite, is produced as a thermal breakdown material in effluents of wastewater during the process of converting coal. Nowadays, pyrogallol is obtained commercially via decarboxylation of gallic acid under high pressure and temperature [22]. It is utilised by various industries and consumer products [17].
Pyrogallol possesses antibacterial, antipsoriatic, antifungal and oxidative properties [18, 23-25]. It is autoxidised rapidly in solutions ranging from pH 3.5 to 4.5 [26] and generates free radicals such as molecular oxygen (O2), peroxide nitrite (ONOO−), hydrogen peroxide (H2O2), and hydroxyradical (OH-) by the Haber- Weiss reaction Inui et al. [16, 26]. The auto-oxidation of pyrogallol is extremely influenced via metallic ions as Cu2+, Fe3+ and Mn2+ increase while Co2+ reduces the rate of auto-oxidation [17]. These free radicals may enable the inhibition of parasite growth. Previous studies reported that the antimalarial drugs such as primaquine, chloroquine and derivatives of artemisinin consist of free radicals, which are a source of oxidation that may cause parasite death [27]. For instance, reactive oxygen species (ROS) are produced by artemisinin in the digestive vacuole through the activation of endoperoxide bridge and free Fe+2 can increase the production of ROS by Fenton process; hence, it has been suggested that artemisinin-activated ROS may induce parasite death via reducing the capability of the parasite’s antioxidant defence system to eliminate free radicals [28]. As mentioned above, pyrogallol is autooxidised in the pH ranging from 3.5 to 4.5, thus, this compound can function within the parasite’s digestive vacuole as this organelle also has the pH ranging from 3.7-6.5 [29]. This indicates that pyrogallol has a great potential to be a future antimalarial drug candidate.
Research studies reported several toxic end points occurred after exposure to pyrogallol [30,31]. The exposure to pyrogallol could cause many toxic side effects such as carcinogenesis, mutagenesis and hepatotoxicity [17, 20, 32-34] through the induction of the oxidative stress, which in turn leads to the destruction of different types of human cells. It could impair immune response through the inhibition of phagocytosis as well as suppressing the proliferation of lymphocytes due to its strong potential for generating free radicals [35]. As a result, Nanoparticles (NPs) is suggested to be used for reducing the toxicity of the drug molecules. Numerous studies reported that the conjugation of antimalarial drugs with some types of nanoparticles enables the direct action of the drugs on the parasite’s digestive vacuole, thus achieving high efficacy and low toxicity [36].
Conclusion
To sum up, the present review provides a better understanding of how pyrogallol can be the potential drug candidate against the malaria parasite through the production of free radicals. It has also discussed the treatment-related toxicity and offers a possible solution in a very brief way.
Acknowledgement
This review was supported by the Fundamental Research Grant Scheme (FRGS) (203/PPSK/6171225).
Conflict of Interest
No conflict of interest.
References
-
Alfaqih, H. and Abu-Bakar, N. The Potential of Pyrogallol as a Possible Antimalarial Drug Candidate. Acad J Microbiol & Immun. 1(1): 2020. AJMI.MS.ID.000503.
-
Glucose-6-phosphate dehydrogenase, Artemisinin based combination therapy, Hydrogen peroxide, Reactive oxygen species, Nanoparticles
-
This work is licensed under a Creative Commons Attribution-NonCommercial 4.0 International License.