Research Article
Efficacy of A Novel Antithrombogenic Coating for Cerebral Protection Devices
Suraj Kapa1*, Koji Kadowaki2, Chance Witt1, Christopher V Desimone1, Deepak Padmanabhan1, Hajime Sakakibara2, Takahiro Yagi2, Masaki Fujita2, Kazuhiro Tanahashi2, Alan Sugrue1, Omar Yasin1, Dorothy J Ladewig1 and Samuel J Asirvatham1
1Department of Cardiovascular Medicine, Mayo Clinic College of Medicine, USA
2Toray Industries, Inc., 1-1, Nihonbashi-Muromachi 2-chome, Chuo-ku, Japan
Suraj Kapa, Department of Cardiovascular Medicine, Mayo Clinic College of Medicine, Rochester, MN, USA.
Received Date:February 08, 2022; Published Date:February 25, 2022
Abstract
Background: There is a high rate of cerebral microemboli during invasive left-sided cardiac procedures. Cerebral protection may be useful to reduce risks. However, intravascular catheters may have risk for forming clot. We sought to evaluate effect of a novel antithrombogenic coating on clot formation on cerebral protection devices.
Method: Two different types of novel antithrombogenic coated filter materials (A- and S-coated) were evaluated in 3 canines and compared against uncoated devices. Brain MRIs prior to and after the procedure were done. Catheters were left in the left or right carotid arteries until occluded or for up to 4.5 hours. Antegrade cerebral blood flow was evaluated and clot occupation rate as a percentage of filter surface area was calculated.
Result: 4 non-coated, 3 A-coated, and 6 S-coated devices were evaluated. 3/4 non-coated catheters occluded over average 20 minutes (min) while one remained patent for 4.25 hours. 1/3 A-coated catheters occluded due to arterial spasm within 32 min while 2 remained patent over an average 3.5 hours. 3/6 S-coated catheters occluded after an average 29 mins while 3/6 remained patent over an average 4.1 hours. Average clot occupation was greater in non-coated (53+6%) than S-coated (38+11%) or A-coated (24+19%) catheters (p<0.05). No animals exhibited MRI evidence of microemboli on post-procedural MRIs.
Conclusion: Cerebral protection devices may be at risk of impeding antegrade flow due to clot deposition, arterial spasm, or mis-sizing. Clot deposition on filter material used for cerebral protection devices is a risk and novel antithrombogenic materials may reduce this risk.
Keywords:Stroke; Anticoagulation; Interventional cardiology; Microemboli; Thrombus
Introduction
Left-sided electrophysiology procedures, including pulmonary vein isolation and ventricular tachycardia ablation, are associated with a 20-40% risk of asymptomatic cerebral microemboli and as high as a 1-2% risk of transient ischemic attack or stroke [1- 4]. While initially asymptomatic, cerebral microemboli have been suggested to carry neuropsychologic sequelae in patients undergoing left-sided ablation procedures (2). Given the increasing numbers of ablations done for atrial fibrillation and ventricular tachycardia, approaches to reduce embolic risk have become of particular interest. One method for achieving this is cerebral protection devices, which generally consist of a catheter placed in either the aortic arch or carotid arteries with a deployable meshlike filter that can capture any material that may embolize.
The risks of cerebral protection devices, however, includes potential for impeding antegrade cerebral blood flow, particularly if the pores in the mesh occlude with clot or other substances. In addition, there is a risk of thrombus formation on long-dwelling intravascular catheters [5, 6]. As a result, novel materials that may limit thrombus formation to further reduce embolic risk or vascular occlusion may be useful. We sought to evaluate the feasibility of using a novel biologically compatible material coating for such cerebral protection devices.
Material and Method
According to an IACUC approved protocol, three canines were prospectively evaluated at Mayo Clinic in Rochester, MN.
Procedural protocol
Two different types of novel antithrombogenic coated filter materials (A-coated and S-coated) were evaluated in canines and compared against uncoated devices. All canines had brain MRIs prior to and after the procedure. Catheters were left in the left or right carotid arteries until occluded or for up to 4.5 hours while subjects were heparinized to maintain an ACT>300 seconds. Periodic contrast injections were performed to evaluate antegrade cerebral blood flow and, after removal of catheters, clot occupation rate as a percentage of the filter surface area was calculated.
Catheter design and material modifications
The novel filter material consists of a nickel-titanium frame with a polyester mesh (Figure 1). The filter itself is composed of a polyester mesh with a novel antithrombogenic polymer coating that inhibits both enzymatic activity of thrombin and platelet adhesion (Toray Industries, Inc., Tokyo). Specifically, two different coatings were used – A-coated and S-coated – which were characterized by different investigative polymers both capable of inhibiting thrombin activity and platelet adhesion. Each pore in the polyester mesh was 100 μm in length on one side. The polyester yarn is 27 μm in diameter. The mesh filter is mounted on a self-expandable nickel-titanium frame to allow the filter to conform to the interior diameter of each branch artery. The diameter of the frame ring is 4 to 6 mm. The frame ring is connected to a stainless-steel shaft (0.2mm in diameter) with four polyester threads. A slidable tube which consists of a polyimide tube (distal part) and stainless-steel pipe (proximal part) is set over the core shaft. The filter ring can close when the operator pushes the slidable tube along the core shaft. The soft tip made from the polyether block amide is then attached to the distal end of the care shaft. The filter is delivered to the carotid artery via the femoral artery using a 6 French guiding catheter. Three different types of mesh were used – 1 non-coated, and two with the afore-mentioned antithrombogenic coating. A-coated devices again had a different anti-thrombogenic polymer than the S-coated devices.
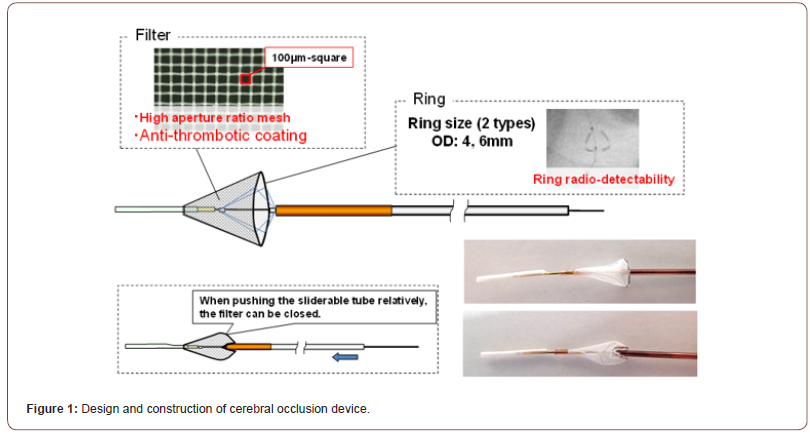
Clot deposition evaluation
The mesh filter was removed from the nickel-titanium flame of each catheter, and all mesh filter samples were fixed by 10% formalin solution. We observed all fixed samples by our method (Figure 2) and calculated clot occupation rate by the following equation.
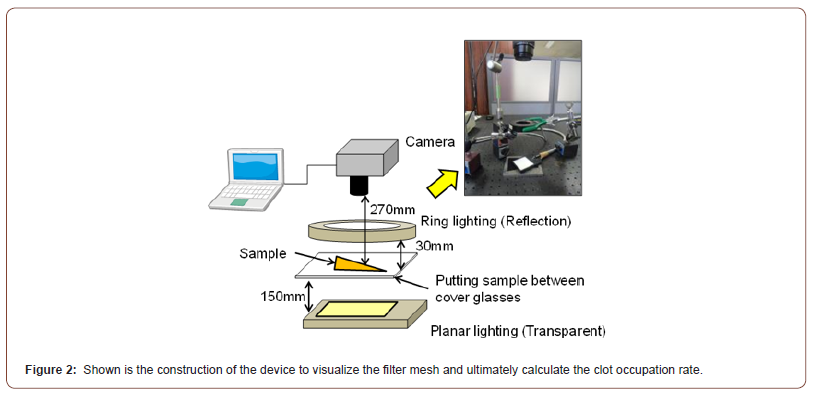
Clot occupation rate (%) = the area of clot adhesion/ the overall area of the filter mesh x 100
Evaluation of the amount of adsorbed fibrinogen in vitro
Briefly, 5mm X 5mm meshes were immersed in human plasma for 24 hours at 37oC and then rinsed with PBS-T (0.05%Tween20/ PBS) 3 times. After immersion in blocking solution for an hour at 37oC and then rinsing with PBS-T 3 times, the samples were next immersed into PBS-T containing a monoclonal mouse antifibrinogen antibody for an hour at room temperature, rinsed with PBS-T 3 times, and then immersed into PBS-T containing anti-IgG mouse goat polyclonal HRP for an hour at room temperature. After rinsing with PBS-T 3 times, the samples were immersed into TMB One solution for 5 minutes at room temperature. The reaction was stopped by the addition of 1N HCl and optical density (OD, 450/595nm) was measured by the plate reader.
Statistical analysis
Categorical variables were compared using a Fisher exact test given the small number of examples.
Result
A total of three canines were included. There were no acute complications with vascular access. The sequence of catheter exchanges between non-coated, A-coated, and S-coated devices and clot deposition rates are summarized in (Table 1). All canines maintain an ACT > 300 sec throughout the procedure from initial catheter deployment to final removal, with average ACT being 321+21.
Table 1:

A total of 4 non-coated, 3 A-coated, and 6 S-coated devices were evaluated (Table 2) summarizes time to occlusion of each in each canine.) 3/4 (75%) non-coated catheters occluded based on angiography over an average 20 minutes after deployment with the fourth remaining patent for 4.25 hours. 1/3 (33%) A-coated devices occluded over 32 minutes after deployment while the other 2 remained patent over 3.5 hours. Of note, in the case of the 1 A-coated device occlusion, on review of angiography it appeared that there was vasospasm of the carotid artery that was relieved with nitroglycerin injection. 3/6 (50%) S-coated catheters occluded over an average 29 mins after deployment while the remaining 3/6 remained patent over 4.1 hours.
Table 1:

2/6 S-coated catheters were felt to have occluded due to missizing of the device while 1/6 had heavy clot deposition. Average clot occupation rate was greater in coated (53+6%) than S-coated (38+11%) or A-coated (24+19%) catheters (p<0.05). There was no significant difference in clot coverage rate between S- and A-coated catheters. No animals exhibited MRI evidence of microemboli or other pathology on post-procedural MRIs.
To elucidate the different performance between A-coating and S-coating, the amount of adsorbed fibrinogen on non-coated, A-coated or S-coated mesh was evaluated in vitro (Figure 3). There was no significant difference in the amount of adsorbed fibrinogen between non-coated and S-coated mesh. On the other hand, about 45% lower adsorbed fibrinogen as compared to non-coated mesh was observed in A-coated mesh (p<0.05).
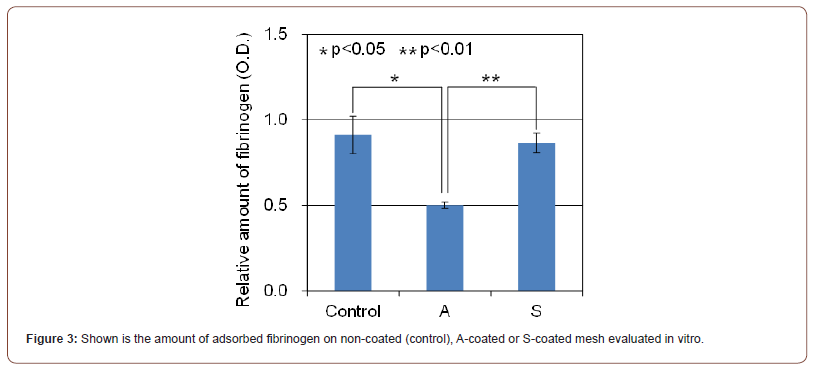
Discussion
Development of cerebral protection devices to facilitate invasive left-sided interventional and electrophysiologic procedures is of increasing interest given the findings of a significant frequency of both symptomatic and asymptomatic embolic events [1-4]. These events may occur irrespective of anticoagulation status either prior to or during the procedure. As a result, identifying novel ways to protect against cerebral events during invasive left-sided procedures may be important.
There are limited studies to date on cerebral protection devices [7-9]. Devices assessed thus far in small clinical studies appear safe. However, any indwelling catheter may carry risk of developing thrombus over a surface exposed to the vasculature. Furthermore, whether deployed in the carotids or the aortic arch, if the mesh or catch device used to protect against embolic material reaching the brain occludes, this may result in reduced cerebral perfusion and potentially watershed or ischemic infarcts due to lack of antegrade flow. Thus, identifying approaches to protect against these complications may be useful.
We demonstrate that a novel biocompatible anti-thrombogenic coating can reduce the rate of clot deposition on catheter surfaces and permit longer duration of patency in an animal model. Despite clot deposition on the mesh material of almost all catheters, no cerebral embolic events were seen, suggesting that the device itself was successful in preventing distal embolization. Additionally, according to the comparison between S-coated catheters and A-coated catheters, these results suggested that A-coating showed a better anti-thrombogenic performance than S-coating, probably due to the ability of A-coating to prevent the fibrinogen adsorption on the mesh. This is likely directly related to the unique enzymatic inhibitors used.
One interesting finding from our study was the frequency of vascular occlusion attributable to events other than occlusion of the mesh of the deployed catheter. In one out of four catheters that were coated with antithrombogenic material, the mechanism of vascular occlusion appeared to be vascular spasm. In two of the remaining four, it was hypothesized that the mechanism of vascular occlusion was due to mis-sizing of the device relative to the size of the carotid artery. A less likely explanation was vascular spasm given the lack of such apparent findings on angiography. A third possibility was that contents (eg, thrombus) occluding the mesh may have embolized during removal of the device and thus were not obvious upon device removal. However, this is unlikely given the absence of MRI findings of cerebral emboli. While the post-hoc evaluation of the non-coated devices suggested that extensive clot deposition likely led to vascular occlusion, the absence of significant clot burden on the S-coated devices suggested against this as a cause. In (Figure 4), we propose one mechanism by which device mis-sizing may have contributed to apparent vascular occlusion.
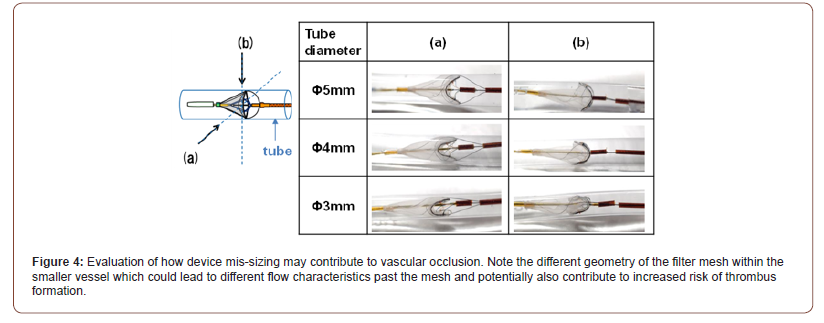
One thing that is unknown is whether evidence of vascular occlusion had or would have had any significant neurologic consequences as neurologic assessments on the canines were not performed pre-euthanasia. Thus, the incremental clinical benefit of such devices remains to be seen. However, with increasing research into subclinical cerebral microemboli during intracardiac procedures, consideration of novel devices to prevent these events may prove useful. Furthermore, whether use of anti-thrombogenic coatings may confer benefit for other indwelling devices, such as pacing and defibrillator leads, left atrial appendage occlusion devices or ventricular assist device cannulate, remains to be seen.
Limitations
The most significant limitation of our study lies in the small number of animals evaluated. Whether similar outcomes would have borne out amongst a larger number of animals is unclear. Another limitation is the potential for embolization of material during device removal which may have resulted in underestimation of clot deposition on the device surface. This is unlikely given the lack of apparent embolic events on post-procedural MRI. However, it is possible that some material may have embolized and dissolved thus making assessment of clot deposition inconsistent. In addition, effects on the carotid intima were not specifically studied, and thus whether deployment of such catheters could lead to intimal damage that could, in turn, also serve as a nidus for thrombus formation, was not studied. Finally, neurologic effects of catheter use in the carotids – particularly as it relates to transient vascular occlusion - were not specifically evaluated.
Conclusion
Cerebral protection devices may help protect against embolism of material during invasive cardiac procedures. Novel antithrombogenic coatings may allow for a lower rate of occlusion of the exposed mesh or catchment device while preventing additional thrombus formation on exposed surfaces. Future research is required to evaluate the utility of these devices during cardiac ablation or other procedures in which thromboembolic events may occur.
Acknowledgement
KK, HS, TY, MF, and KT are employees of Toray, Inc, which has IP rights over the specialized polymers used in this study.
Conflicts of Interest
No conflict of interest.
References
- Takami M, Lehmann HI, Parker KD, Welker KM, Johnson SB, et al. (2016) Effect of left atrial ablation process and strategy on microemboli formation during irrigated radiofrequency catheter ablation in an in vivo model. Circ Arrhythm Electrophysiol 9: e003226.
- S Kochhäuser, H H Lohmann, M A Ritter, P Leitz, F Güner, et al. (2015) Neuropsychological impact of cerebral microemboli in ablation of atrial fibrillation. Clin Res Cardiol 104: 234-240.
- Isaac R Whitman, Rachel A Gladstone, Nitish Badhwar, Henry H Hsia, Byron K Lee, et al. (2017) Brain emboli after left ventricular endocardial ablation. Circulation 135: 867-877.
- Robert Larbig, Ralf Dittrich, Simon Kochhaeuser, Patrick Leitz, Fatih Guener, et al. (2016) Influence of clnical parameters an anticoagulation on intraprocedural cerebral microembolic signals during pulmonary vein isolation. PLoS One 11: e0157886.
- Dorbala S, Cohen AJ, Hutchinson LA, Menchavez-Tan E, Steinberg JS (1998) Does radiofrequency ablation induce a prothrombotic state? Analysis of coagulation system activation and comparison to electrophysiology study. J Cardiovasc Electrophysiol 9: 1152-1160.
- Maleki K, Mohammadi R, Hart D, Cotiga D, Farhat N, et al. (2005) Intracardiac ultrasound detection of thrombus on transseptal sheath: Incidence, treatment, and prevention. J Cardiovasc Electrophysiol 16: 561-565.
- Christian-Hendrik Heeger, Andreas Metzner, Michael Schlüter, Andreas Rillig, Shibu Mathew, et al. (2018) Cerebral protection during catheter ablation of ventricular tachycardia in patients with ischemic heart disease. J Am Heart Assoc 7: e009005.
- Samir R Kapadia, Susheel Kodali, Raj Makkar, Roxana Mehran, Ronald M Lazar, et al. (2017) Protection against cerebral embolism during transcatheter aortic valve replacement. J Am Coll Cardiol 69: 367-377.
- Gary M Ansel, L Nelson Hopkins, Michael R Jaff, Paolo Rubino, J Michael Bacharach, et al. (2010) Safety and effectiveness of the INVATEC MO.MA proximal cerebral protection device during carotid artery stenting: Results from the ARMOUR pivotal trial. Catheter Cardiovasc Interv 76: 1-8.
-
Suraj Kapa, Koji Kadowaki, Chance Witt, Christopher V Desimone, Deepak Padmanabhan etc all. Efficacy of A Novel Antithrombogenic Coating for Cerebral Protection Devices. On J Cardio Res & Rep. 6(3): 2022. OJCRR.MS.ID.000640.
-
Heart, Cardiology, Cardiac contraction, Vascular pulsation, Pericardium, Vascular system, Hidden intrathoracic diseases, Aortic valve
-
This work is licensed under a Creative Commons Attribution-NonCommercial 4.0 International License.